Definitions of TNM
Primary tumor (T)
Clinical
TX
Primary tumor cannot be assessed
T0
No evidence of primary tumor
T1
Clinically inapparent tumor neither palpable nor visible by imaging
T1a
Tumor incidental histologic finding in 5% or less of tissue resected
T1b
Tumor incidental histologic finding in more than 5% of tissue resected
T1c
Tumor identified by needle biopsy (e.g., because of elevated PSA)
T2
Tumor confined within prostatea
T2a
Tumor involves one-half of one lobe or less
T2b
Tumor involves more than one-half of one lobe, but not both lobes
T2c
Tumor involves both lobes
T3
Tumor extends through the prostate capsuleb
T3a
Extracapsular extension (unilateral or bilateral) including microscopic bladder neck involvement
T3b
Tumor invades seminal vesicle(s)
T4
Tumor is fixed or invades adjacent structures other than seminal vesicles such as external sphincter, rectum, bladder, levator muscles, and/or pelvic wall
Pathologic (pT)c
pT2
Organ confined
pT2a
Unilateral, one-half of one side or less
pT2b
Unilateral, involving more than one-half of side, but not both sides
pT2c
Bilateral disease
pT3
Extraprostatic extension
pT3a
Extraprostatic extension or microscopic invasion of bladder neckd
pT3b
Seminal vesicle invasion
pT4
Invasion of rectum, levator muscles, and/or pelvic wall
Regional lymph nodes (N)
Clinical
NX
Regional lymph nodes were not assessed
N0
No regional lymph node metastasis
N1
Pathologic
pNX
Regional nodes not sampled
pN0
No positive regional nodes
pN1
Metastases in regional node(s)
Distant metastasis (M)e
M0
No distant metastasis
M1
Distant metastasis
M1a
Non-regional lymph node(s)
M1b
Bone(s)
M1c
Other site(s) with or without bone disease
Table 21.2
AJCC anatomic stage and prognostic groups for prostate cancera
Group | T category | N category | M category | PSA | Gleason |
---|---|---|---|---|---|
I | T1a–c | N0 | M0 | PSA < 10 | Gleason ≤ 6 |
T2a | N0 | M0 | PSA < 10 | Gleason ≤ 6 | |
T1–2a | N0 | M0 | PSA X | Gleason X | |
IIA | T1a–c | N0 | M0 | PSA < 20 | Gleason 7 |
T1a–c | N0 | M0 | PSA ≥ 10 < 20 | Gleason ≤ 6 | |
T2a | N0 | M0 | PSA < 20 | Gleason ≤ 7 | |
T2b | N0 | M0 | PSA < 20 | Gleason ≤ 7 | |
T2b | N0 | M0 | PSA X | Gleason X | |
IIB | T2c | N0 | M0 | Any PSA | Any Gleason |
T1–2 | N0 | M0 | PSA ≥ 20 | Any Gleason | |
T1–2 | N0 | M0 | Any PSA | Gleason ≥ 8 | |
III | T3a–b | N0 | M0 | Any PSA | Any Gleason |
IV | T4 | N0 | M0 | Any PSA | Any Gleason |
Any T | N1 | M0 | Any PSA | Any Gleason | |
Any T | Any N | M1 | Any PSA | Any Gleason |
Risk Stratification and Primary Treatment of PCa
After initial diagnosis, the decision to proceed with further diagnostic imaging is guided by which treatment options are available to the patient, taking into consideration his preference, age, and comorbidity. The management of PCa is challenging because the disease has variable clinical and histopathologic patterns [16].
A critical step in the initial management that affects the choice of treatment and predicts long-term outcome is tumor staging, which allows risk stratification. AJCC staging (Table 21.1) is based on at least three factors, that is, the serum PSA level, the Gleason score, and the tumor stage [17–19]. Higher PSA levels are associated with more advanced pathological stage and higher likelihood of distant disease. The Gleason score is the most commonly employed index for histologic grading of PCa; it ranges between 2 and 10, 2 representing the least aggressive and 10 the most aggressive pattern [20]. To calculate the Gleason score, the pathologist classifies the microscopic glandular pattern of the specimen into 1 of 5 categories: (1) The glands are well formed and closely packed, resembling normal prostate; (2) the glands are larger with more tissue between them but are still well formed; (3) some of the cells invade the surrounding tissue, but glands are still recognizable; (4) many cells are invading surrounding tissue, with few recognizable glands; and (5) sheets of cells are invading surrounding tissue, no recognizable glands. The pathologist looks for the pattern seen in >50% of the cells and uses that score for the primary grade. The pathologist then looks for the pattern seen in >5% and <50% of the tissue for the secondary grade. The final Gleason score is the sum of the primary and secondary grades.
It is important to emphasize that, in the setting of initial diagnosis, tumor staging is based only on small biopsy specimens; thus, discrepancies may occur between the initial stage and final pathological stage (when radical prostatectomy is performed). Another variable that is available only after radical prostatectomy is the status of resection margins; positive margins are associated with worse outcome [21].
As part of risk assessment, it is necessary to assess whether or not patients have distant disease. Imaging techniques provide useful information for this purpose. In addition, PSA velocity (PSAV) and PSA doubling time (PSADT) may be considered, when available, for definition of risk [19, 22–24]. High PSAV and short PSADT are associated with a greater likelihood of distant disease [25].
Nomograms are frequently used at initial diagnosis to noninvasively predict staging and guide therapy. Nomograms are graphical, manually accessible calculating devices derived from multivariate analysis that predict the risk of certain clinical outcomes (e.g., lymph node disease, highly aggressive cancer) on the basis of a set of biological variables (e.g., PSA, PSADT, Gleason score, pathological stage). Several nomograms have been validated in large clinical studies and are now available in routine clinical practice [26, 27].
The choice of treatment should be patient specific, risk-adjusted, and aimed at improving cancer control while reducing the risks of treatment-related complications. The therapeutic approaches for patients with PCa include: (1) watchful waiting, (2) radical prostatectomy, (3) radiotherapy, (4) brachytherapy, and (5) antiandrogenic hormonal therapy. At present, there are not sufficient data to state the superiority of a given therapeutic approach over another. Variability in PCa treatment reflects not only patients’ characteristics but also the philosophy of the referring physicians (e.g., urologists vs. radiotherapists). In spite of this variability, some general recommendations can be given [2, 5].
Watchful Waiting
The terms “watchful waiting” or “active surveillance” are used to describe a treatment strategy that includes an active decision to postpone treatment until it is required. The rationale for such approach stems by observations indicating that patients with well-differentiated PCa managed conservatively have a 20-year PCa-specific survival rate as high as 80–90% [28, 29]. However, patients who are offered watchful waiting must be followed up carefully, and patient’s compliance is a primary requirement for watchful waiting. Highly compliant patients, with low-risk PCa and limited life expectancy (<10 years), are ideal candidates for deferred treatment.
Radical Prostatectomy
Currently, radical prostatectomy is the only treatment for localized PCa that has shown a cancer-specific survival benefit when compared to conservative management in a prospective, randomized trial [30]. Nerve-sparing radical prostatectomy represents the approach of choice in men with normal erectile function and organ-confined disease, as it improves quality of life without compromising oncological outcome [31].
Lymphadenectomy for PCa is generally limited to pelvic lymph nodes, an approach that is considered to be sufficient in low-risk patients. In high-risk patients, lymphadenectomy extended to retroperitoneal lymph nodes is appropriate. In comparison to pelvic lymphadenectomy, extended lymphadenectomy results in an upstaging in the majority of patients [32].
Radical prostatectomy is a valuable approach for patients with locally advanced tumor. Survival rates are about 95%, 90%, and 79% at 5, 10, and 15 years, respectively [2, 33]. Although it is frequently adopted, neoadjuvant androgen deprivation therapy does not provide a significant advantage in overall survival and progression-free survival.
External Beam Radiation Therapy
Intensity-modulated external beam radiotherapy (or proton beam therapy) is an alternative to radical prostatectomy. The more precise and conformal delivery of radiation therapy has resulted in lower, but not absent, acute and late toxicity [34]. A critical issue in external beam radiation therapy of PCa is that the therapeutic effective dose is limited by radiodosimetric constraints. At least 72 Gy is recommended for the management of low-risk PCa, as it has been shown that the biochemical disease-free survival is significantly longer with this radiation dose as compared to lower doses [35]. Dose escalation up to about 80 Gy has a significant impact on patients’ survival. In patients with high-risk disease, external irradiation with dose escalation up to 80 Gy improves 5-year biochemical disease-free survival, but seems insufficient to cover the risk of relapse outside the pelvis [36].
Adjuvant External Radiotherapy
Adjuvant external beam radiotherapy may be given after prostatectomy to selected patients to improve the clinical outcome. Suitable candidates for immediate adjuvant radiation therapy are patients with multifocal disease, positive surgical margins, Gleason score >7, or PSA nadir >0.1 ng/ml after radical prostatectomy [37]. Studies compared immediate postoperative radiotherapy to radiotherapy delayed until local recurrence in patients with locally advanced PCa after retropubic radical prostatectomy. Immediate postoperative radiotherapy proved to be well tolerated with a risk of grades 3–4 urinary toxicity in 4% of patients. Immediate postoperative radiotherapy after surgery significantly improved 5-year survival by approximately 20% [38].
Brachytherapy
Transperineal low-dose brachytherapy is safe and efficient, and it can be applied to low-risk patients [38]. Most patients experience acute urinary symptoms shortly after implantation, while up to 20% develop chronic urinary morbidity. Results of permanent implants have been reported from different institutions with a median follow-up ranging between 36 and 120 months [39]. Recurrence-free survival after 5 and 10 years was reported to range from 71% to 93% and from 65% to 85%, respectively. There is no benefit in adding neoadjuvant or adjuvant androgen deprivation to transperineal low-dose brachytherapy [39].
Adjuvant Antiandrogenic Hormonal Therapy
There are two main classes of drugs that are useful in antagonizing the growth-stimulating effect of androgens on prostate cells. (1) Agonists of the luteinizing hormone-releasing hormone (LHRH) have become the standard of care in hormonal therapy because they avoid the physical and psychological discomfort associated with orchiectomy and have minimal cardiotoxicity [40]; they modulate pituitary signaling, thereby hindering gonadal steroid synthesis. (2) Nonsteroidal antiandrogens (e.g., bicalutamide) act by directly antagonizing the binding of endogenous androgens to the androgen receptor, thus inhibiting the downstream mitogenic pathways [41]. These two types of drugs can be used for combined androgen blockade, a treatment regimen that can be complete, that is, uninterrupted, or intermittent [2]. The idea of intermittent androgen blockade is to preserve quality of life and to reduce treatment-associated costs without compromising the therapeutic efficacy of such endocrine manipulation. Trials demonstrated the feasibility of intermittent androgen blockade in metastatic or biochemically recurrent disease, with PSA response rates and symptom improvement similar to those of complete androgen blockade [35]. Patients who develop progressive disease and become resistant to combined androgen blockade require chemotherapy; at present, docetaxel is the preferred first-line cytotoxic agent [42].
Radiopharmaceuticals
Bone Scintigraphy
99mTc-labeled diphosphonates are currently used for scintigraphic imaging of the skeleton, mostly 99mTc-methyldiphosphonate (99mTc-MDP) and 99mTc-hydroxymethyldiphosphonate (99mTc-HDP). When skeletal metastases become symptomatic, bone pain is the main symptom, followed by pathological fracture, hypercalcemia, and spinal cord compression [43]. Metastases to the skeleton initiate a cascade of events that in most cases of PCa result in increased remodeling with activation of osteoblasts (sclerotic osteoblastic lesions), thus causing focal increase in tracer uptake. On the contrary, purely osteolytic metastases are rare, they do not induce an osteoblastic reaction and therefore may be scintigraphically negative or appear as a photon-deficient area [44, 45]. Although bone scintigraphy has higher sensitivity than plain-film radiography [46], false-negative bone scans can result from the absence of reactive osteoblastic changes or in the presence of slow-growing lesions in which the osteoblastic reaction bone is not detectable. The specificity of bone scintigraphy is even more limited because uptake of the radiotracer is not tumor specific and can be caused by a wide variety of benign bone abnormalities [47].
In patients with advanced, metastatic disease, the kidneys can be difficult to see in bone scans because of substantial uptake of the radionuclide tracer by multiple bone metastases (termed a “superscan”) [48], a finding associated with poor prognosis (Fig. 21.1).
Technique
Whole-body imaging is performed 3 h following i.v. administration of about 740 MBq of 99mTc-MDP. Two-head gamma cameras allow simultaneous acquisition of planar anterior and posterior images. When needed, single-photon emission computed tomography (SPECT) is employed to acquire tomographic images over a certain field of view. In the current generation of SPECT tomographs, hybrid SPECT/CT imaging allows precise anatomic correlation of functional findings, increasing both sensitivity and specificity of bone scintigraphy [47]. Correlating bone scan findings with CT is rapidly becoming the standard of practice.
18F-Fluoride
The uptake mechanism of the PET tracer 18F-fluoride in bone is similar to that of 99mTc-MDP. After diffusion through capillaries into bone extracellular fluid, fluoride ions exchange with hydroxyl groups in the hydroxyapatite bone crystals to form fluoroapatite, which is deposited mainly at the surface, where bone remodeling and turnover are greatest. Accumulation of 18F-fluoride uptake in malignant bone lesions reflects bone turnover as well as increased regional blood flow [49, 50]. Bone uptake of 18F-fluoride is twofold higher than that of 99mTc-MDP. 18F-fluoride is not bound to protein, thus resulting in faster blood clearance and better target-to-background ratio. Regional plasma clearance of 18F-fluoride is 3–10 times higher in bone metastases than that of normal bone.
Technique
PET scanning with 18F-fluoride is performed 60–90 min after tracer injection. Similar to other PET/CT scanning and at variance with bone scintigraphy, scanning is performed from the skull to the midthigh. Injected activity amounts to 296–444 MBq.
Lymphoscintigraphy and Radioguided Sentinel Lymph Node Biopsy
Because of difficulties of extended and standard pelvic lymph node dissection (high morbidity and, respectively, low sensitivity), lymphoscintigraphy with sentinel lymph node (SLN) biopsy is being proposed to assess the feasibility of selective dissection of the primarily draining lymph nodes of the prostate. The SLN concept is based on the assumption that cancer spread through the lymphatic system is a gradual process [51]. If the first lymph node is negative for metastatic disease, node involvement in subsequent nodes is not expected, thereby avoiding the need for an extended dissection.
Technique
After injection of a radiopharmaceutical at a tumor site, the carrier molecules enter the lymphatic pores and migrate into the lymph vessels, draining them from there towards the first lymph node station. Either 99mTc-nanocolloidal albumin or 99mTc-sulfur colloid (depending on local availability) is used for this examination. The radiocolloid is injected into the prostate transrectally under ultrasound guidance. Since the tumor is not palpable, a peritumoral injection cannot be performed. Since the radiotracer is injected in both prostate lobes, many lymphatic pathways are identified; some of them may not be related to the tumor site. Therefore, the aim of prostate lymphoscintigraphy is to identify all primary lymph nodes draining from the prostate, which inevitably include the true SLN [51]. Another difficulty relates to accumulation of radioactivity in the bladder that may partially interfere with the identification of the lymphatic pathways. Planar dynamic and static images may be acquired up to 2–3 h postinjection. Late acquisition leads to detect more lymph nodes. SPECT/CT fusion imaging can be used to increase the accuracy of the technique [52]. The examination is completed in the surgery room, where a handheld gamma probe is used to detect the SLN intraoperatively.
Radioimmunoscintigraphy
111In-capromab pendetide is an immunoscintigraphic agent (commercialized with the brand name of ProstaScint®, available in the USA and Singapore) based on a murine IgG monoclonal antibody that reacts with a glycoprotein expressed by the prostate epithelium known as prostate-specific membrane antigen (PSMA) [53]. Expression of PSMA is significantly enhanced in malignant prostate cells compared to nonmalignant cells and is upregulated in higher grade, androgen-insensitive, as well as metastatic lesions [54], whereas the antigen is rarely expressed by a limited number of non-prostatic malignant tumors. In 1996, the FDA approved 111In-capromab pendetide for use in imaging of soft tissue but not bone, in the clinical settings of presurgical staging of newly diagnosed patients who are deemed high risk for pelvic lymph node metastases. In addition, it is approved for post-prostatectomy patients with a rising PSA, for whom there is a clinical suspicion of metastatic disease [55]. Immunoscintigraphy is performed after labeling with 111In.
Technique
About 180–220 MBq of 111In-capromab pendetide is injected intravenously. Static images of the pelvis extended to the abdomen are acquired 3–4 days after injection. SPECT/CT images may be acquired to increase diagnostic accuracy of the technique. The acquisition of dual-peak SPECT using 99mTc-labeled red blood cells (to provide anatomic landmarks) in addition to ProstaScint® can further increase diagnostic accuracy [56].
Positron Emission Tomography
Over the last two decades, the development and widespread distribution of PET, and subsequently PET/CT, has substantially powered the possibilities of noninvasively evaluating patients with PCa [57, 58]. In particular, PET/CT has allowed integrating functional and morphological imaging, thus substantially increasing the diagnostic yield of in vivo imaging. There are currently several agents available for imaging PCa (Table 21.3).
Table 21.3
Radiopharmaceuticals used for prostate cancer imaging
99mTc-MDP/99mTc-HDP | Evaluation of bone metastases; widely used in follow-up; poor sensitivity for low PSA values; useful for assessing response to antiandrogenic therapy and chemotherapy (possible false positives owing to the “flare” phenomenon) |
111In-capromab pendetide | Evaluation of nodal recurrence (at initial staging or after rising PSA); not very useful to assess local recurrence and bone disease |
99mTc-colloid lymphoscintigraphy | For sentinel lymph node mapping/biopsy; may be used to limit the extent of lymphadenectomy before radical prostatectomy |
[18F]FDG | Not very useful, due to the low glycolytic activity of PCa; can be used to evaluate aggressiveness and androgen insensitivity in advanced stages of the disease |
[11C]choline | Better than [18F]FDG to evaluate local disease, due to low urinary [11C]choline excretion; greater sensitivity compared to [18F]FDG also for detecting lymph node and skeletal metastases; the major obstacle for routine use is short half-life of 11C (need for in-house cyclotron) |
[18F]choline | Better than [18F]FDG; less urinary excretion and longer half-life of 18F compared to 11C; similar sensitivity as for [11C]choline |
[11C]acetate | Better than [18F]FDG; comparative value versus 11C-choline not yet established (requires large-scale head-to-head studies); an 18F-labeled analog has recently been synthesized |
[11C]methionine | Limited data; potentially useful for evaluating recurrent disease; compares favorably with [18F]FDG; advantages versus radiolabeled choline or acetate not known |
18F-fluoride | More accurate than conventional 99mTc-MDP bone scintigraphy, especially if performed with PET/CT scanner; however, not yet cost-effective |
18F-fluoro-5α-dihydrotestosterone | Limited data; less sensitive than [18F]FDG; can be used to monitor treatment response and possibly to assess androgen insensitivity |
[18F]Fluorodeoxyglucose
The rationale for the use of [18F]fluorodeoxyglucose ([18F]FDG) is that tumor glucose consumption is increased and that tumor metabolic activity relates to the biological behavior. [18F]FDG is a glucose analog that differs from glucose by the substitution of a hydrogen atom with a hydroxyl group on the second carbon atom. It is transported bidirectionally across the plasma membrane by the same transporter as that for glucose (GLUT family). Within the neoplastic cell, [18F]FDG is phosphorylated to [18F]FDG-6-P by hexokinase but, at variance with glucose, is not further metabolized. Thus, trapping of [18F]FDG within the cell is related to glucose metabolism and overall to the increased energetic demand of neoplastic cells.
Technique
PET scans with [18F]FDG are typically performed about 1 h after injection. About 300–400 MBq is injected depending on body weight. Patients are asked to fast overnight to avoid competition of uptake by endogenous glucose, while good hydration enhances [18F]FDG elimination through the kidneys. Five 1-min-long dynamic scans centered on the pelvis are initially acquired. This is necessary for visualizing early [18F]FDG uptake in the prostatectomy bed, a sign consistent with local recurrence. Tracer accumulation in the bladder in delayed images does not allow accurate assessment of focal uptake in this region [59]. After dynamic acquisition, static acquisition is performed from the midthigh to the base of the skull. The sensitivity of [18F]FDG PET/CT for initial staging of well-differentiated PCa (Gleason 5–7) is relatively low. On the other hand, in patients with hormone-refractory disease, [18F]FDG PET/CT is valuable for delineating sites of active disease.
Radiolabeled Choline
Radiolabeled choline is by far the most successful PET tracer for studying PCa [60]. Interest in this tracer is derived from studies indicating increased choline kinase activity in PCa and relative increased concentration of choline-derived phospholipids (phosphorylcholine) [61–63]. Within the cells, choline is phosphorylated by choline kinase, the first enzyme involved in phospholipid synthesis. Other enzymes are necessary for synthesis of choline-derived membrane phospholipids. The exact metabolic mechanism underlying the trapping of choline is yet unknown. Increased biosynthesis of membrane phospholipids in rapidly duplicating tumor cells was initially postulated as the primary mechanism accounting for choline uptake [60], but it is possible that multiple metabolic pathways contribute to tracer uptake. The extent of uptake of [11C]choline is not related to cell proliferation rate, as estimated by Ki67 [64].
Choline can be labeled with either 11C or 18F [60]. 11C labeling has the advantage of yielding a natural compound; however, due to the short half-life of 11C (20 min), an on-site cyclotron is necessary. Due to the longer half-life of 18F (109.8 min), 18F-labeled choline compounds such as 18F-fluoroethylecholine (FEC) and 18F-fluoromethyldimethyl-2-hydroxyethylammonium (FCH) have been synthesized. The main difference between 11C- and 18F-labeled tracers is that 18F-labeled compounds have some urinary excretion starting as early as 3–5 min after injection. In vitro studies revealed that cellular uptake and phosphorylation by choline kinase is very similar for FCH and natural choline, while it is lower for FEC. Thus, FCH seems to be a better tracer than FEC for PCa imaging [65].
Technique
Acquisition includes early dynamic scanning of the prostatic bed and subsequent static acquisitions. However, early dynamic acquisition has less importance than in the [18F]FDG studies. The liver is the primary site for choline metabolism, and urinary excretion is much lower compared to that of [18F]FDG. Nevertheless most centers perform early dynamic PET/CT studies with radiolabeled choline. This allows, under normal conditions, to assess the prostatic bed with late static acquisition. Urinary excretion is minimized by inviting patients to avoid drinking in the 6 h preceding the exam. Static acquisition is initiated as early as 5 min postinjection. In fact, [11C]choline is cleared very rapidly from the blood, and optimal tumor-to-background contrast is reached within 5–10 min.
[11C]Acetate
[11C]Acetate has been used for many years for estimating mitochondrial oxidative metabolism in the myocardium. [11C]Acetate was shown to accumulate in the prostate tumors to a greater extent than [18F]FDG [66]. It is postulated that metabolic activity in the tumor occurs in a low-oxygen microenvironment, in association with an increased lipid synthesis that accompanies rapid cell growth. PCa itself is associated with an increase in fatty acid synthesis and the overexpression of fatty acid synthase [67]. Dynamic acquisitions showed an initial high tracer uptake and much slower clearance of tissue tracer activity than those from nonneoplastic parenchyma. In vitro studies showed that tumor cells incorporated radioactivity into the lipid-soluble fraction, mostly represented by phosphatidylcholine and neutral lipids. Moreover, the lipid-soluble fraction of radioactivity accumulation in cells showed a positive correlation with cell growth [47, 67]. These findings suggest that radiolabeled acetate incorporation is mainly due to the enhanced lipid synthesis. An 18F-labeled derivative of acetate has been recently synthesized and preliminarily evaluated in clinical trials.
Technique
Acquisition protocols are similar to those employed for [11C]choline PET.
Staging of Prostate Cancer with Imaging Techniques
Imaging techniques may be useful for the detection of PCa, even though they are mostly valuable for staging purposes. A combination of different imaging techniques is usually adopted at initial assessment.
Transrectal Ultrasound
At present, TRUS is frequently used for the detection and for local staging of PCa. The technique is widely available and easy to use and provides well-contrasted quality images. PCa most frequently presents as a hypoechoic area within the peripheral zone. However, up to 40% of cancers are isoechoic, thus resulting in suboptimal sensitivity of the technique [68]. Moreover, hypoechoic areas within the peripheral zone may also be found in benign processes, such as prostatitis [68]. Thus, the ability of TRUS to delineate cancer foci is limited, with sensitivity and specificity varying around 40–50% [69]. In addition to diagnosis, TRUS is used for local staging of PCa. Altered morphology of the prostate capsule and seminal vesicle enlargement identifies locally advanced PCa [70]. However, the prediction of extracapsular extension of PCa varies widely, with sensitivities ranging from 50% to 92% and specificities ranging from 58% to 86% [71, 72]. Contrast-enhanced TRUS is a new technique that is under investigation for the assessment of PCa [73]. A study showed that contrast-enhanced TRUS improved the sensitivity of TRUS in tumor detection from 38% to 65%, with no significant change in its specificity (80%) [72].
CT
Despite the recent developments in detector technology, CT still has a limited role in the detection and local staging of PCa [74, 75]. CT has limited soft tissue contrast resolution, which prevents the accurate depiction of the prostate contours as distinct from adjacent muscles and ligaments. Zonal anatomy is difficult to discern on unenhanced scans but can often be seen early after a bolus of iodinated contrast medium. Nonetheless, tumors are very difficult to identify unless they are large [76]. CT may be helpful in the evaluation of patients with locally advanced PCa, although its incremental value compared to TRUS is uncertain [75, 77]. CT is useful for the detection of metastatic lymph node involvement, as PCa typically spreads initially to pelvic lymph nodes and retroperitoneal lymph nodes. Nevertheless, CT has limited sensitivity for nodal staging due to the fact that node disease is present only in a minority of patients at initial presentation (most commonly in the form of micrometastasis). For lymph nodes smaller than 15 mm, the correlation between lymph node diameter and presence of disease is relatively poor [76, 78, 79]. CT has been used to detect bone metastases, but bone scintigraphy is superior to CT in the diagnosis of bone metastases [75].
MR
Magnetic resonance (MR) imaging is very sensitive for the noninvasive, anatomic evaluation of PCa at initial staging [80, 81]. On MR imaging, PCa is typically seen on T2-weighted images as a focus of decreased signal intensity. As with CT, this finding is nonspecific. The accuracy of MR imaging in the local staging of PCa varies widely across studies (from 50% to 90%) [77, 80, 81]. The accuracy of MR may be enhanced by using spectroscopy (MRS). MRS provides metabolic information about prostatic tissue, by displaying the relative concentrations of various compounds within contiguous voxels. Typical changes observed in PCa include decreased citrate level (because of enhanced citrate-oxidating metabolism) and increased choline concentration (because of the high phospholipid cell membrane turnover in proliferating malignant tissue) [76, 82]. Therefore, voxels containing PCa display an increased choline-to-citrate ratio MR, particularly through the use of using diffusion-weighted imaging, is a very promising technique for detection of bone metastases, but has the noteworthy disadvantage of not allowing a whole body scan [75].
Lymphoscintigraphy and Radioguided SLN Biopsy
Because of the difficulties previously discussed, SLN lymphoscintigraphy and radioguided biopsy are still considered experimental in PCa patients, although several studies report promising findings [83–85]. In particular, Wawroschek et al. demonstrated the feasibility of SLN mapping in 348 patients with PCa. 99mTc-nanocolloid was injected into each lobe of the prostate under TRUS guidance. After removing the SLNs, the patients underwent either modified or extended lymphadenectomy, depending on their preoperative risk stratification. The SLN technique demonstrated metastasis in 24% [83] of patients.
Lymph node metastases are often located in the hypogastric region, a region that is not included in the standard pelvic lymph node dissection. More than 60% of N+ patients are falsely classified as pN0 stage if only a standard pelvic lymph node dissection is done [84]. Thus, the sensitivity of the technique is higher (>95%) when performing extended pelvic lymph node dissection compared to a standard pelvic lymph node dissection (80%).
Recently, a large study was carried out in more than 2,000 men who underwent SLN dissection alone or in combination with either standard or extended lymphadenectomy after radical retropubic prostatectomy [85]. Lymph nodes positive for metastases were found in 17% of patients. The scintigraphic detection rate was 97.6% and the intraoperative detection rate was 98%. These results demonstrate that SLN scintigraphy in PCa is potentially a reliable procedure for nodal staging. SLN staging in PCa is associated with reduced surgical complexity and reduced complication rate compared with extended lymph node dissection while retaining maximal sensitivity for detection of lymphatic metastases [85].
Bone Scintigraphy
Bone scintigraphy is used to rule out skeletal metastasis in PCa patients. Patients with bone metastases have a poor prognosis; the median survival after diagnosis of metastasis is few years, depending on the characteristics of the patient’s primary tumor, disease burden, presence of metastases in other organs, and levels of biochemical markers of bone turnover [43].
Through the use of nomograms, data such as PSA level, digital rectal examination, and biopsy results can be used to identify patients at high risk of metastatic disease at their initial diagnosis, which can reduce the need for bone imaging [86]. The sensitivity of bone scintigraphy is rather poor in patients with relatively low serum PSA levels, and the percentage of patients with positive bone scan remains as low as 1% for PSA <20 ng/mL and 5% for PSA values as high as 30–40 ng/mL [87–89]. Patients with low-risk cancer are unlikely to have metastatic bone involvement. For this reason, the routine use of bone scintigraphy for primary staging in PCa patients with low risk has been discouraged. However, bone scintigraphy is indicated in symptomatic patients with bone pain, with increased serum alkaline phosphatase levels, or with PSA levels >10 ng/mL or Gleason score ≥7 [2] (see clinical examples in Fig. 21.1).
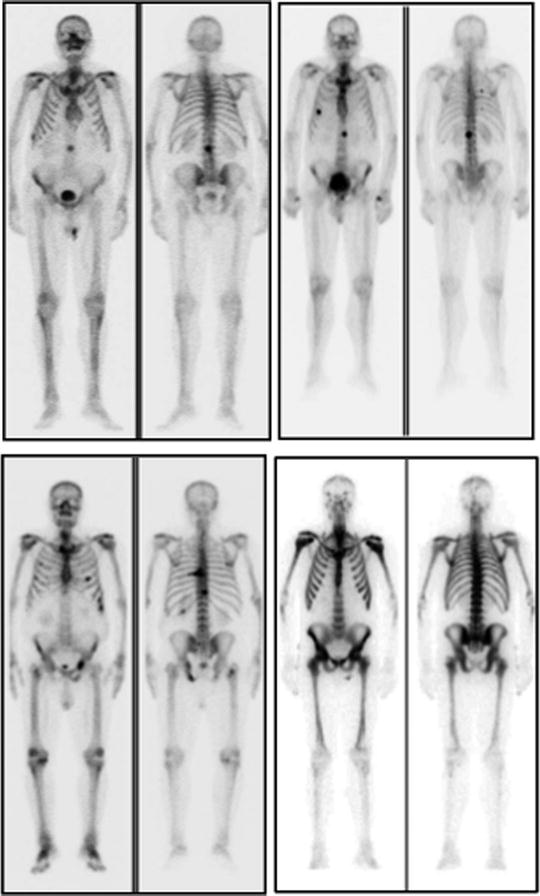
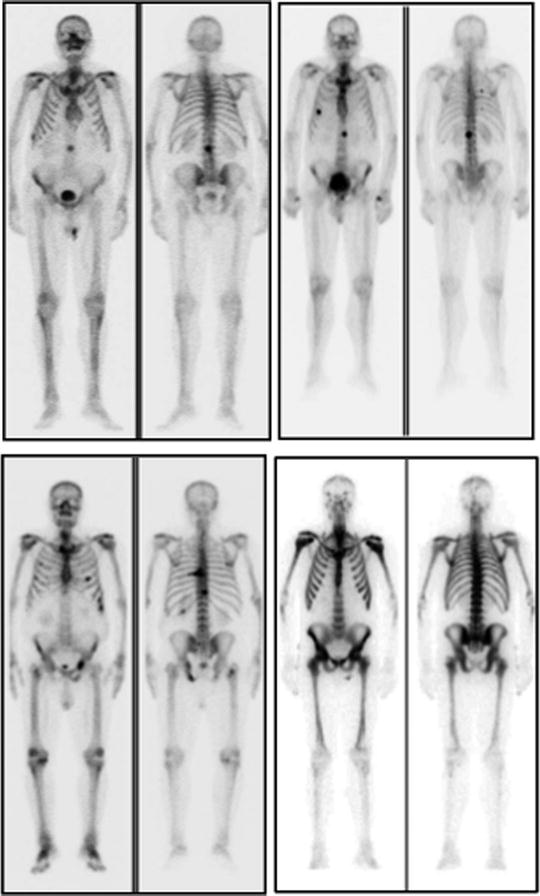
Fig. 21.1
Examples of 99mTc-HDP whole-body planar scans performed for staging in patients with newly diagnosed prostate cancer. Upper left panel (serum PSA 80 ng/mL): single metastatic lesion in lumbar spine, while uptake is slightly increased also in the lateral aspect of the tenth right rib. Upper right panel (serum PSA 115 ng/mL): metastatic lesions in spine and rib cage, while bilaterally increased uptake in first carpo-metacarpal joints is caused by degenerative changes (rhizarthritis). Lower left panel (serum PSA 180 ng/mL): multiple metastatic lesions in spine, rib cage, pelvis, and right femoral neck. Lower right panel (serum PSA 540 ng/mL): diffusely increased uptake virtually in the whole skeleton. This pattern (which is not exclusively but typically observed in patients with prostate cancer) is usually defined as “superscan” and is characterized by only faint or no visualization of the kidneys (as normally seen in a bone scan because of physiologic renal excretion of 99mTc-phosphonates). This patient only complained of flu-like symptoms since about 2 weeks and mild, vague pain in the lumbar tract of the spine, which he attributed to trauma that occurred 2 months earlier
The spine is the most common site for metastases arising from several neoplasms. Metastatic spread is the cause of 20–50% of solitary spine lesions, and 30–50% of patients with metastatic involvement of the spine are asymptomatic. As the spine is a frequent site for degenerative joint disease, the diagnostic accuracy of planar bone scintigraphy is low, particularly for a single focus of abnormal increased tracer uptake. Many studies showed that SPECT can minimize the shortcomings of planar bone scintigraphy in the assessment of the spine. SPECT has, in comparison to bone scintigraphy, improved sensitivity (87–92%), specificity (about 91%), and overall accuracy (90%) [49, 90–92].
The addition of SPECT (possibly performed with hybrid SPECT/CT equipment) to planar acquisition improves the diagnostic accuracy (especially specificity) of bone scintigraphy for detecting malignant bone involvement (Fig. 21.2) and allows a straightforward comparison with other tomography-based techniques, such as CT and MR.
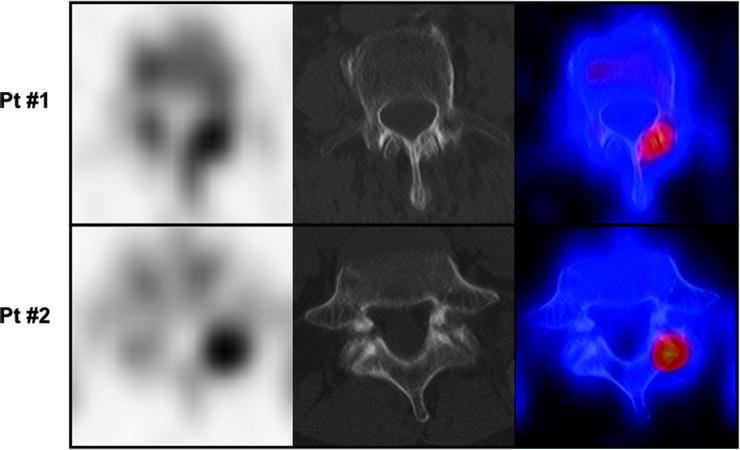
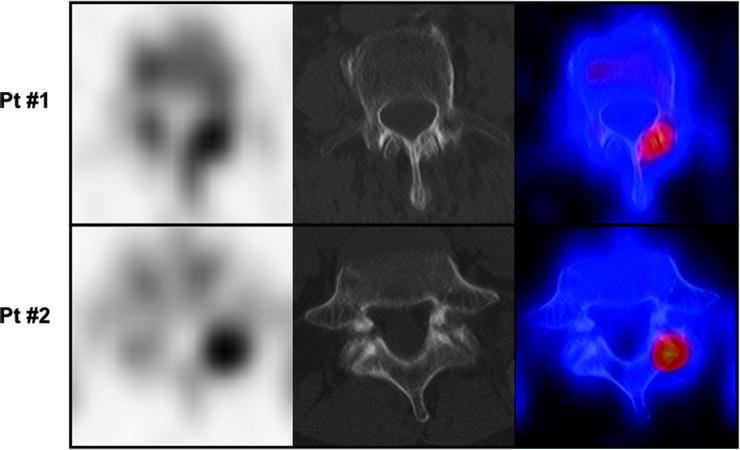
Fig. 21.2
Transaxial sections focused on vertebral bodies (SPECT images in left panels, CT images in center panels, SPECT/CT fusion images in right panels) obtained in two different patients undergoing 99mTc-MDP scintigraphy in whom the planar scan had detected isolated foci of increased uptake in the spine. In patient #1 (upper row), increased uptake corresponds to the interpeduncular joint and is caused by degenerative changes. In patient #2 (lower row), the focus of increased uptake corresponds to the left pedicle, and it represents a metastatic lesion (From Römer W, Beckmann MW, Forst R, Bautz W, Kuwert T. SPECT/Spiral-CT hybrid imaging in unclear foci of increased bone metabolism: a case report. Rontgenpraxis. 2005; 55: 234–237, with permission)
Radioimmunoscintigraphy
The role of 111In-capromab pendetide for local staging is debated. Despite initial studies that reported promising results, many other authors reported that 111In-capromab pendetide is not reliable in detecting seminal vesicle invasion [93]. However, 111In-capromab pendetide may be used to enhance the accuracy of lymph node staging in PCa patients. In patients with a high likelihood for extraprostatic disease, lymph node involvement can be detected with 62–75% sensitivity and 72–86% specificity, whereas CT and MR imaging do not perform as well [94–96]. False-negative scans occur with pathologic lymph nodes that contain microscopic or small tumor volumes [95]. As a consequence, a negative scan does not eliminate the need for a staging lymphadenectomy. Immunoscintigraphy with 111In-capromab pendetide provides evidence over and above serum PSA and Gleason score for predicting extraprostatic disease in pelvic lymph nodes. Furthermore, 111In-capromab pendetide provides important prognostic information, as patients with PCa that overexpress PSMA in the prostate gland have twice the recurrence rate and a shorter time to recurrence than those with normal PSMA expression [97]. The accuracy of the technique may be increased by the use of hybrid SPECT/CT to avoid false-positive studies (Fig. 21.3) [98].
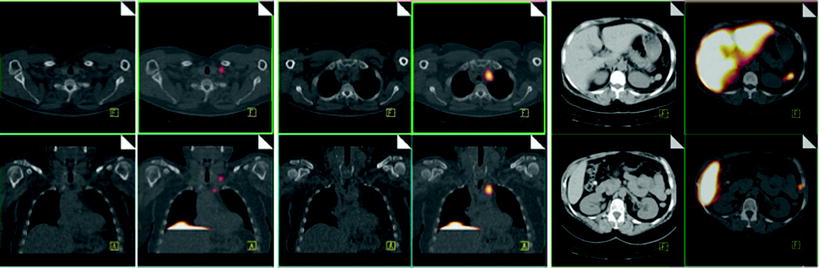
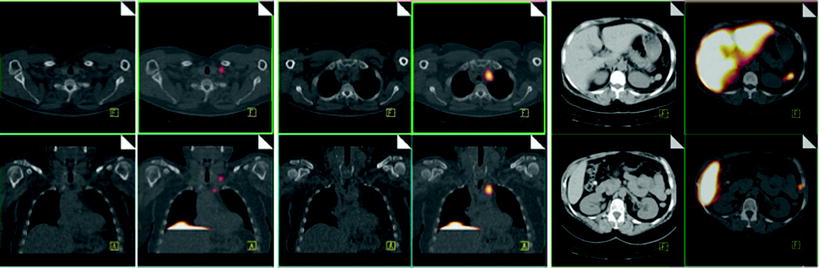
Fig. 21.3
Immunoscintigraphy with 111In-capromab pendetide performed to evaluate status post-prostatectomy. SPECT/CT images show metastasis in left supraclavicular lymph nodes (left panel), in left paratracheal lymph nodes (center panel), and in the abdomen (right panel). (Courtesy of the Cleveland Clinic)
PET
PET and PET/CT has been used to noninvasively stage PCa before surgery or before other initial treatments. Overall, studies assessing the role of PET at initial staging are fewer than studies performed for disease restaging, and studies with either [11C]choline or 18F-labeled agents outnumber studies with [18F]FDG. The accuracy of PET/CT has been assessed for both tumor and distant staging.
Many primary PCas show relatively low [18F]FDG uptake, and prostate assessment can be masked by tracer accumulation in the bladder. Low [18F]FDG uptake in nodal and bone metastases has also been observed [108, 114–119].
More favorable findings were reported with radiolabeled choline. To allow a comparison between histopathologic data and PET/CT findings, the tracer uptake in the prostate was analyzed by identifying six regions of interest (apex, mid, and base) corresponding to the six histopathologic sextants (of prostate). In nonselected samples, the accuracy of the technique to identify PCa was 60–80%. Causes for this finding are that PCa is often multicentric and tumor volume is much smaller than the volume of the whole prostate. On the other hand, focal uptake may occur in benign diseases (such as prostatitis and benign hyperplasia), thus possibly causing false positives. Moreover, the PET signal is smeared onto neighboring regions because of partial volume effect. Accuracy of the technique may be increased by dual time imaging, whereby tracer uptake will increase in malignant areas, but not in nonmalignant areas, by registering PET volumes to MR volume and by including in the analysis only patients at high risk or with tumors larger than 5 mm.
Regarding lymph node staging, varying degrees of sensitivity and specificity were reported depending on the inclusion criteria. Lymph node metastases were detected with a sensitivity as high as 80% and specificity as high as 100%. Values were generally higher in subject-based analysis than in lesion-based analysis [126, 127].
In patients with intermediate-risk and high-risk PCa, [11C]choline PET/CT had a low sensitivity for detecting lymph node metastases (60%) but performed better than clinical nomograms based on traditional risk factors, with equal sensitivity and better specificity.
Because of the low incidence of bone metastases at initial staging, PET scanning with either [11C]choline or 18F-fluoride is unlikely to be useful in patients with PSA <20 ng/mL and clinical or instrumental suspicion for osseous metastases.
Restaging of Prostate Cancer with Imaging Techniques
Following primary treatment, biochemical failure of PCa, characterized by measureable or rising PSA levels, occurs in about 30–50% of patients within 10 years following treatment [99, 100]. The PSA threshold used for the definition of biochemical failure depends on the primary treatment. Following radical prostatectomy, PSA levels greater than 0.2 ng/mL on at least two consecutive samples at 3 months apart indicate biochemical failure [101]. In patients treated with antiandrogenic therapy or radiotherapy, a PSA value greater than 2 ng/mL above the nadir after therapy represents recurrent cancer [102, 103].
Bone Scintigraphy
Identification of skeletal metastases has important clinical implications. Patients with metastases are offered androgen withdrawal and bisphosphonate therapy. External beam radiation therapy is generally reserved for treating lesions causing intractable pain. The primary goal of scintigraphic assessment in patients with high-risk PCa is to detect bone metastases early, to initiate therapy to limit the progression of these lesions. The extent of osseous metastatic disease from PCa is an independent prognostic factor per se.
Bone scintigraphy is recommended as the first-line modality in the most recent European Association of Urology guidelines for PCa. The probability of detecting bone metastases is increased when a PSA >20 ng/mL is identified. Fast PSA kinetics, locally advanced disease, short time from surgery to biochemical recurrence, and Gleason score ≥8 increase the detection rate of bone metastases. Skeletal metastases are, however, observed also in patients with PSA levels <5 ng/mL [43–121].
Generally, bone scintigraphy has high sensitivity in detecting osteoblastic metastatic bone disease. A known limitation of bone scintigraphy is its relative lack of specificity, which can be overcome with SPECT/CT. The CT findings help distinguish degenerative disease or vertebral fracture from metastases. Often, review of the bone SPECT can help distinguish malignant from benign findings. For example, focal uptake involving the vertebral body and pedicles is typically an ominous sign, whereas increased uptake at the edge of the vertebral body adjacent to the disc and extending beyond the vertebral body contour and focal uptake in the facet joints are more typical of benign degenerative disease (Fig. 21.2). SPECT bone scans have sensitivity of 87–92% and specificity of 82–92% [90, 104].
Bone scintigraphy may be used to monitor the response to treatment in PCa patients with known bone metastases and to assess tumor burden (Fig. 21.4). The bone scan may be assessed visually, on a lesion-by-lesion basis, and scoring semiquantitative indices may be obtained on the basis of the number and distribution of lesions. Such scoring systems have prognostic value, even though they are time consuming and therefore not widely adopted for clinical care. A potential bias in assessing the response to therapy with bone scintigraphy is related to the occurrence of the flare phenomenon; this is a paradoxical increase in tracer uptake in patients who ultimately display a favorable outcome (on the basis of bone scintigraphy alone, such patients would be classified as having progressive disease). The flare phenomenon has been reported in 6–25% of PCa patients with bone metastasis. The flare phenomenon occurs most frequently in the 3 months after initiation of a new treatment (either hormonal or chemotherapy) and rarely persists beyond 6 months. It may result from increased blood flow caused by an inflammatory response or from enhanced turnover of the new bone laid down as part of the healing process. In PCa, if the scan taken 3 months after introduction of therapy shows worsening of disease, there is a high probability that this is real progression of osseous disease. If, however, the patients’ clinical parameters indicate a response, then flare should be considered. A follow-up scan at 6 months can resolve the issue. False positives may occur also following trauma [43].
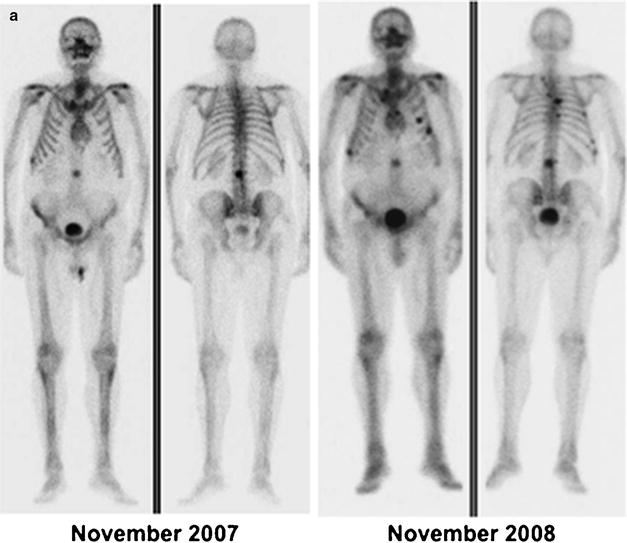
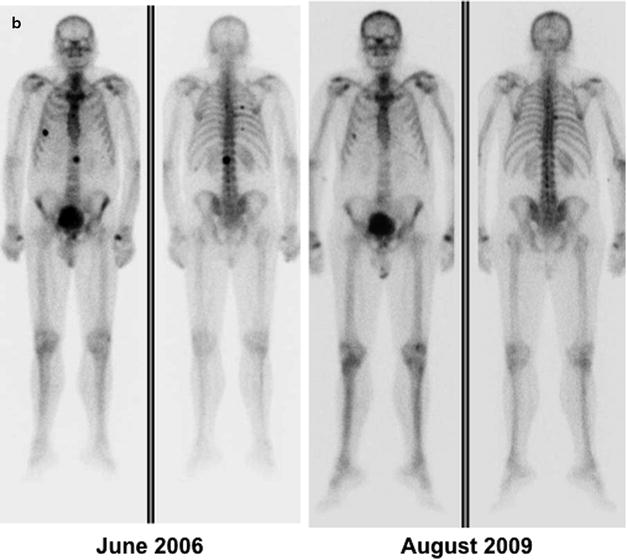
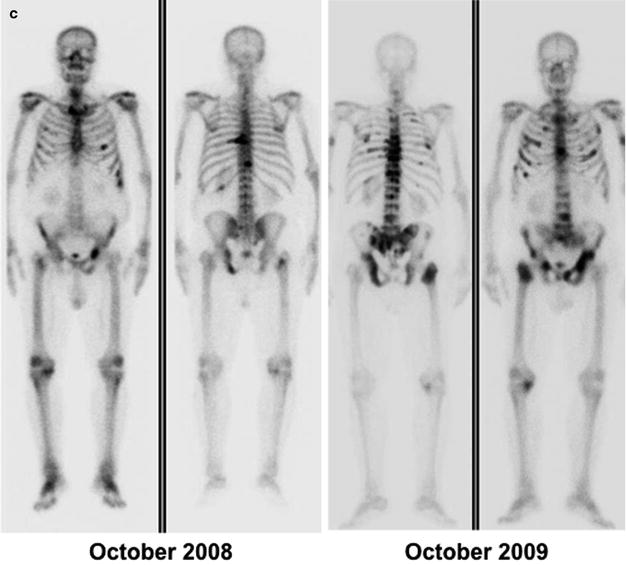
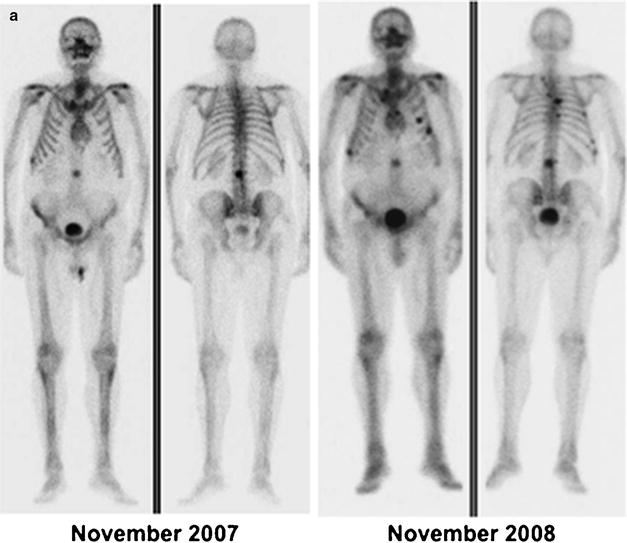
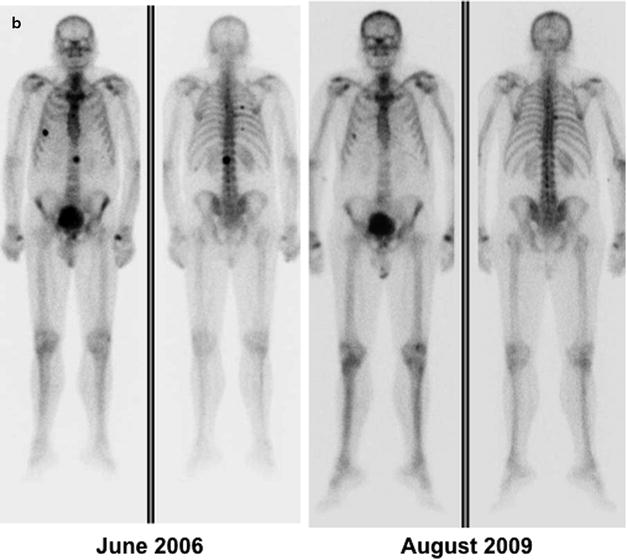
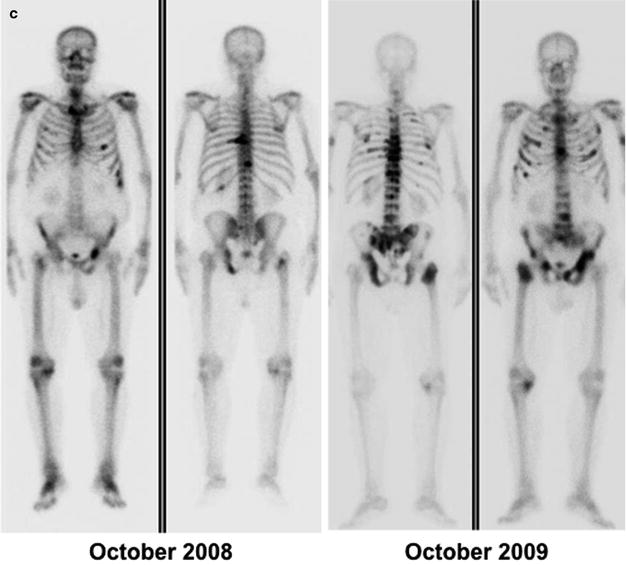
Fig. 21.4
(a) Metastatic lesion in lumbar spine shown by whole-body bone scintigraphy with 99mTc-HDP (anterior and posterior projections) in patient with newly diagnosed prostate cancer and with serum PSA 80 ng/mL (left panel); uptake is slightly increased also in the lateral aspect of the tenth right rib. One year later (right panel), the bone scan shows disease progression despite hormonal therapy (serum PSA 210 ng/mL). (b) Skeletal metastases from prostate cancer in the spine and rib cage (left panel, serum PSA 115 ng/mL) improving after 3 years on hormonal therapy (right panel, serum PSA 2.4 ng/mL). (c) Patient with hormone-refractory prostate cancer (serum PSA 180 ng/mL) and scintigraphic findings of bone metastasis in the spine, rib cage, pelvis, and right femoral neck (left panel). At the 1-year follow-up evaluation, scintigraphy shows progression of disease despite chemotherapy (right panel, serum PSA 320 ng/mL)
ProstaScint®
111In-capromab pendetide immunoscintigraphy is primarily used for restaging PCa with biochemical recurrence, to identify the site of relapse [105]. In patients with biochemical progression of disease, 111In-capromab pendetide immunoscintigraphy scanning had 67–94% sensitivity and 42–80% specificity for the detection of lymph node metastases [94, 106]. Coregistration of immunoscintigraphic images with MR imaging or CT could improve the specificity of the examination [90, 104] (Fig. 21.5). However, the suboptimal quality images combined with the availability of successful PET tracers reduce the need for imaging with this agent.
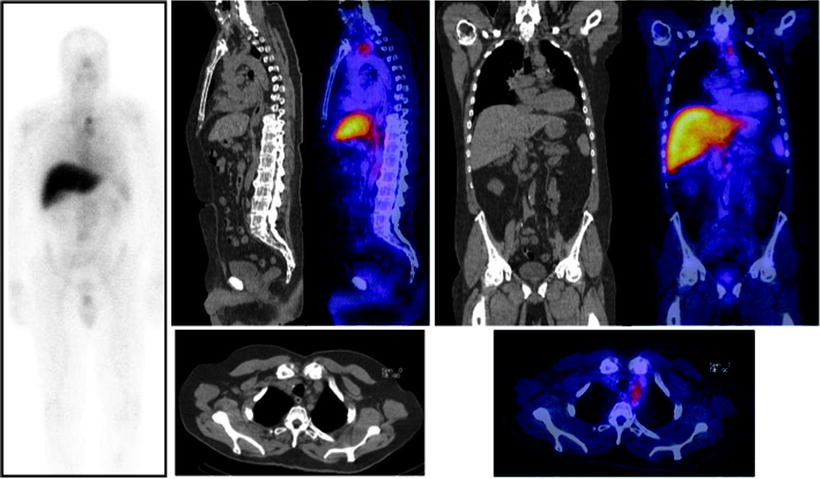
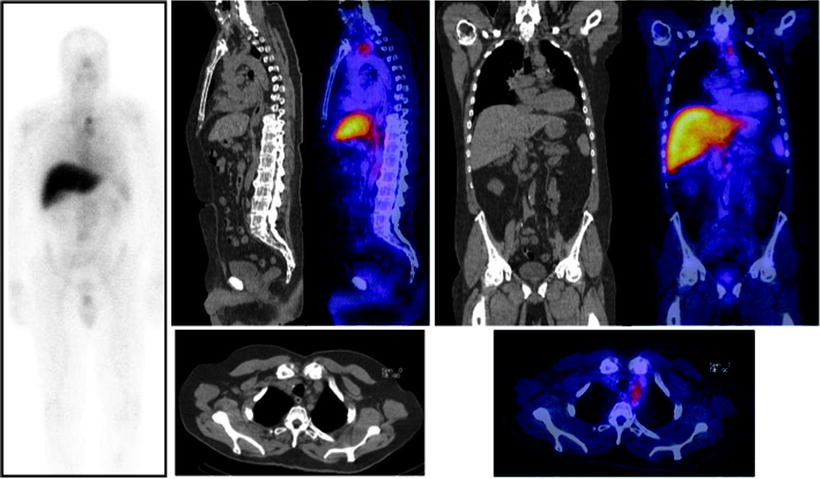
Fig. 21.5
Immunoscintigraphy with 111In-capromab pendetide performed because of rising serum PSA post-prostatectomy. Planar whole-body scan shows abnormal radioactivity accumulation in the upper mediastinum (left panel). SPECT/CT images allow localizing metastatic lesions to a group of lymph nodes between left subclavian vein and left internal jugular vein (sagittal CT and fused sections in center panel, coronal CT and fused sections in right panel, transaxial CT and fused sections in bottom panels) (Courtesy of the Cleveland Clinic Foundation, Cleveland, OH, USA)
PET
Over the last decade, several studies were performed with different PET tracers for restaging purposes in patients with PCa.
[18F]FDG
Initial PET studies with [18F]FDG in PCa patients were disappointing [107, 108]. This is due to the fact that PCa is a slowly growing tumor that does not utilize glucose as its major source of energy. The majority of PCa tumors are well differentiated, a feature typically associated with poor [18F]FDG trapping. Studies comparing [18F]FDG versus radiolabeled choline showed a lower number of pathological uptake sites and lower uptake intensity with [18F]FDG compared to radiolabeled choline [108]. This finding was true for both lymph nodes and for the skeleton. Moreover, in tumors with predominantly sclerotic features, [18F]FDG is less accurate for the assessment of skeletal involvement, and such lesions show lower tracer uptake than lytic metastases as assessed by standardized uptake value.
There was no difference between the [18F]FDG uptake of benign prostate hyperplasia, prostate carcinoma, postoperative scar, or local recurrence after radical prostatectomy. Therefore, [18F]FDG was reported not to be useful to distinguish between postoperative scar and local recurrence after radical prostatectomy [109–112].
Patients with metastases/recurrences, high Gleason scores and fast PSA kinetics often display high [18F]FDG uptake [110, 113]. These findings are thought to be due to loss of cellular differentiation accompanying disease progression. However, as the disease progresses and the probability of a positive [18F]FDG scan increases, the chance of a positive bone scan also increases so that the relative benefit of the PET examination decreases.
In patients with metastatic PCa, [18F]FDG was able to discriminate active from quiescent osseous lesions [113]. Disease progression during chemotherapy was correctly identified by [18F]FDG PET in 91% of these cases.
[11C]Choline
In patients treated with radical prostatectomy that experienced biochemical failure (i.e., PSA > 0.2 ng/mL), tracer uptake was seen in metastatic lesions in 30–80% of the patients [108, 114]. Pathological uptake sites were identified in the prostatectomy bed as well as at distance (Fig. 21.6). Subsequent studies performed in samples that included also patients with locally confined disease indicated that PSA is the single, most powerful predictor of a positive [11C]choline PET/CT [108, 115–117].
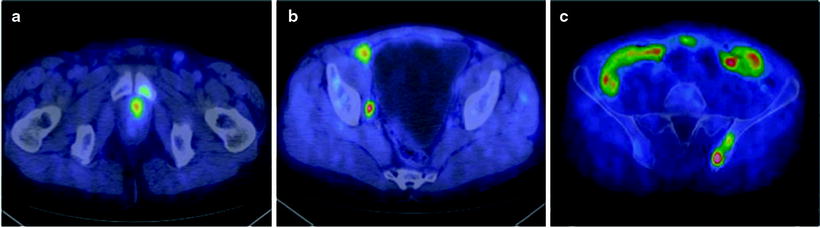
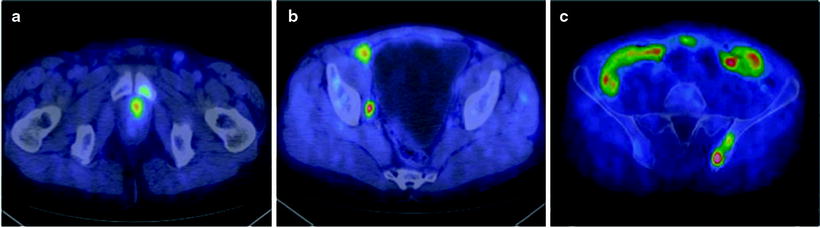
Fig. 21.6
[11C]choline PET/CT fused images of local recurrence (a), metastases to right external and internal iliac lymph nodes (b), and metastases to the left iliac bone (c) in a 67-year-old patient with PSA levels of 0.93 ng/mL during therapy with enantone. The Gleason score was 4 + 3 and stage was pT2bpN1. After PET/CT, paclitaxel was added, and a partial, transitory reduction of PSA levels was obtained (From [117], with permission)
In large populations, the pattern of regional localization of [11C]choline uptake is consistent with the natural spread of the disease. Lymph nodes are the most frequent anatomical sites of increased [11C]choline uptake (30%). Pelvic lymph nodes are involved twice as often as retroperitoneal lymph nodes, while the skeleton is affected in about 20% of patients [117]. Finally, local recurrence can be detected only in 15% of patients, indicating an overall low sensitivity to assess the prostatectomy bed. In presence of multiple risk factors, skeletal metastases were found also in patients with low PSA values (PSA <1 ng/mL) [117]. The fraction of patients with positive [11C]choline PET/CT ranges from 20% for PSA <1 ng/mL, to 46% for PSA between 1 and 3 ng/mL, and to 82% for PSA higher than 3 ng/mL (Fig. 21.7).
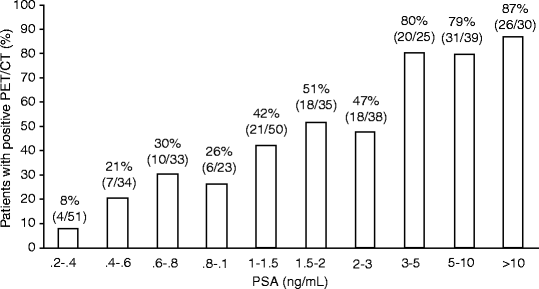
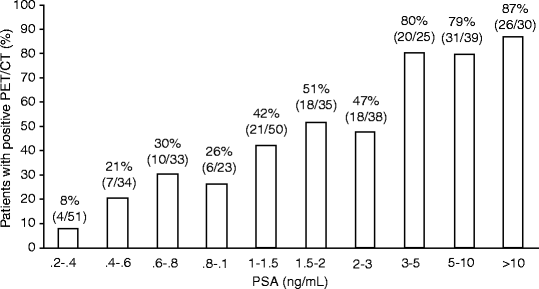
Fig. 21.7
Percent fractions of patients with positive [11C]choline PET/CT over different intervals of serum PSA levels (From [117], with permission)
PSADT also predicts [11C]choline PET/CT findings. Patients with very short PSADT (<3 months) are known for an increased risk of PCa-specific mortality. In such patients, the number of pathological skeletal [11C]choline uptake sites is highest, while it is the number of cases with pathological [11C]choline uptake in the prostatectomy bed which is lowest (Fig. 21.8) [118, 119]. This finding parallels previous results obtained with bone scintigraphy and CT [120–122], indicating that short PSADT is associated with distant disease and long PSADT is associated with local recurrence. Clinical outcome is worse in patients with short PSADT compared to patients with long PSADT [19, 123].
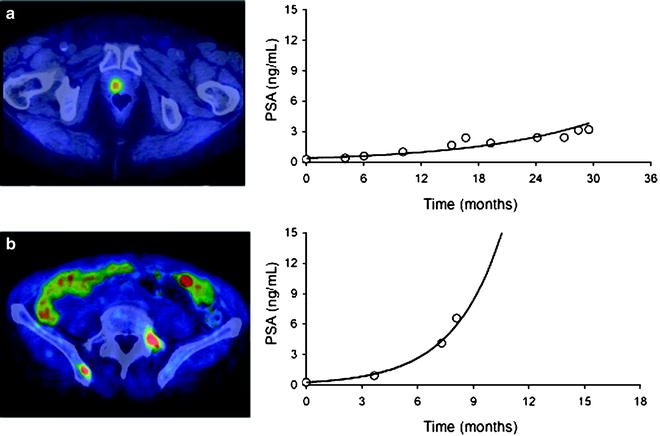
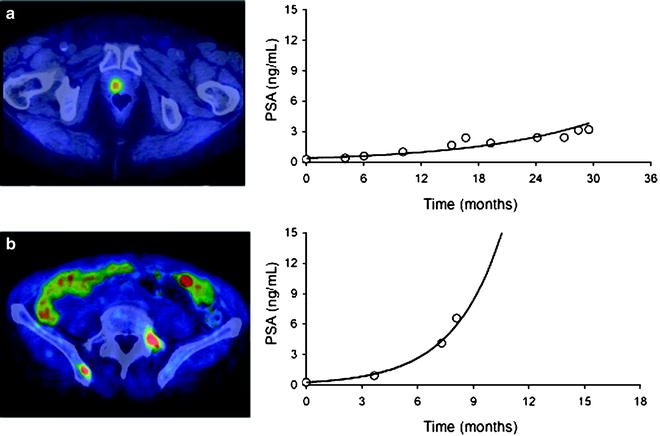
Fig. 21.8
(a) Patient with abnormal [11C]choline uptake in the prostatectomy bed. There were no other sites of pathological [11C]choline uptake; PSADT was 8.9 months. (b) Patient with abnormal [11C]choline uptake in the left transverse process of L5 and in the right iliac bone; PSADT was 1.9 months (From [118], with permission)
In highly selected patients with biochemical recurrence and no evidence of disease on conventional imaging, [11C]choline PET/CT did show focal uptake suggestive of disease in about 10% of cases. This finding highlights the sensitivity of the technique compared to conventional imaging and suggests that [11C]choline PET/CT can be used for early detection of recurrence [124].
The technique has intrinsic limitations. False negatives are particularly frequent in the prostatectomy bed [108, 125]. The reasons for this finding are not clear, but it could be related to small tumor volume and limited spatial resolution of present-day PET tomographs. In particular, micrometastases (that are especially frequent in lymph nodes) are not detectable by the technique. False positives are also encountered; in fact, increased [11C]choline uptake is not specific for carcinogenesis, as focal tracer uptake may occur in inflammatory and degenerative diseases as well [126–128].
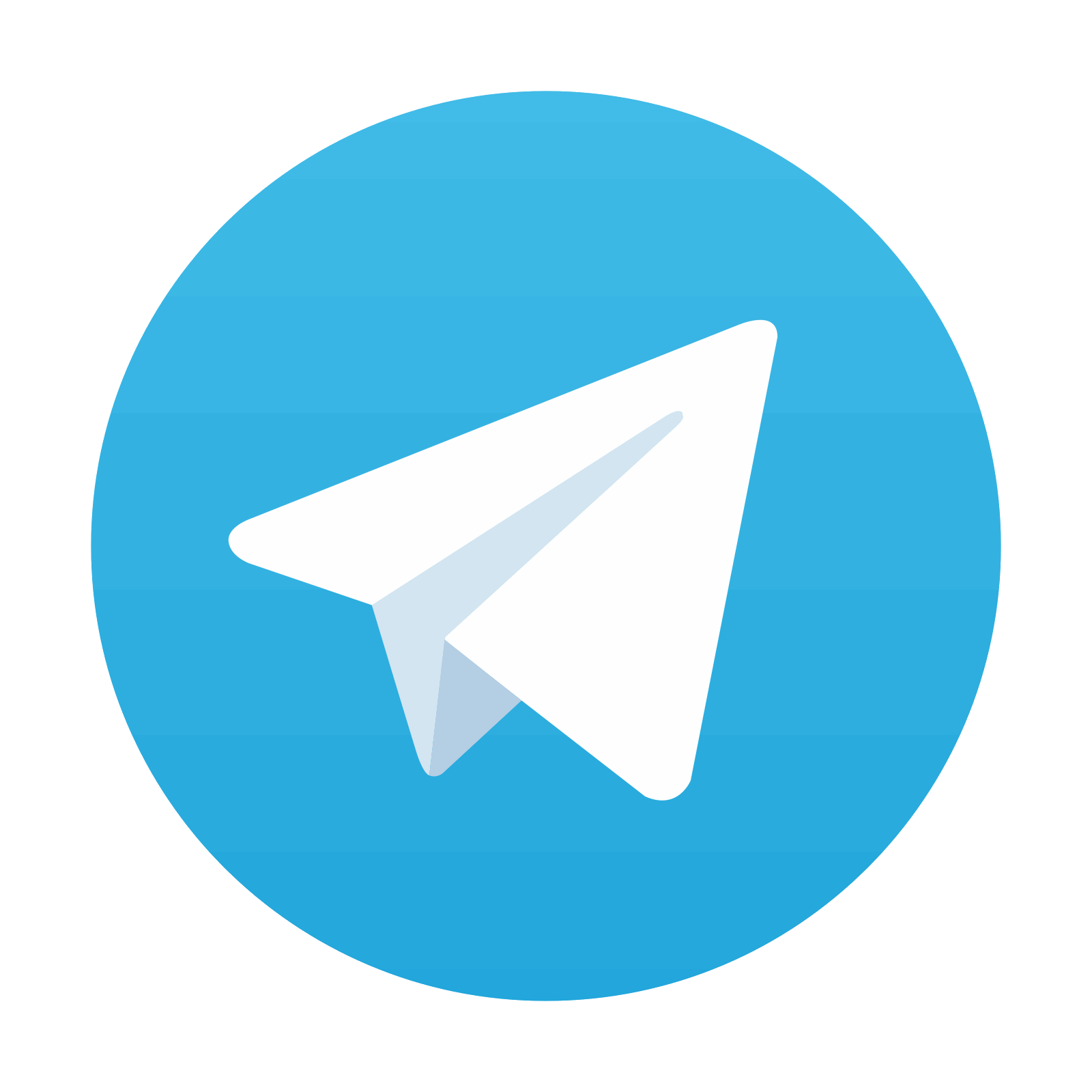
Stay updated, free articles. Join our Telegram channel

Full access? Get Clinical Tree
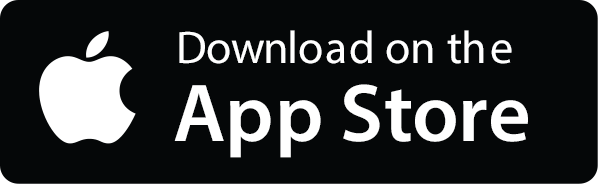
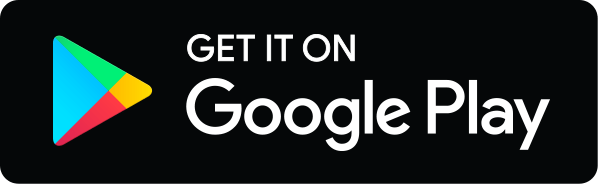