Fig. 30.1
Patient with Hodgkin’s lymphoma treated with bleomycin. Diffuse bilateral uptake of 67Ga-citrate reflects pulmonary toxicity
Recently, pulmonary [18F]FDG uptake has been reported in patients with BIP and absence of pulmonary symptoms [11, 12]. In a study done by Butchler et al. [13], the PET scan performed within 2 months of onset of BIP demonstrated [18F]FDG uptake in the lungs which resolved after corticosteroid treatment. Conventional CT scanning was not able to distinguish between residual changes and active inflammation. This data suggests that [18F]FDG-PET may provide a tool to indicate the resolution of disease activity, even in the presence of residual pulmonary scarring [13]. Along the same line, Connerotte et al. [12] have shown that PET abnormalities can resolve after cessation of bleomycin as the only intervention, suggesting that [18F]FDG-PET could be useful to detect early preclinical BIP at a stage that is fully reversible upon cessation of bleomycin. Therefore, [18F]FDG-PET may be used in the detection of BIP before clinical symptoms, and possibly before significant lung function or conventional radiology alterations take place.
Pulmonary Permeability in Chemotherapy
In chemotherapy-induced lung injury, pulmonary permeability is altered because of alveolar epithelial disruption with fluid and protein leakage into the alveolar spaces and focal necrosis of type I pneumocytes and fibrosis. Permeability can be assessed using 99mTc-DTPA aerosols. The inhalated aerosol is deposited in the alveoli, permitting the acquisition of scintigraphic images that show different patterns of ventilation. In the noninflammed lung there is minimal permeability of the DTPA from the air space to the vasculature, hence minimal activity in the systemic circulation. The inflammed lung has greater permeability, allowing passage of the tracer from the air space to the vasculature. The clearance time decreases as epithelial permeability [14, 15] increases. In untreated patients, permeability was reduced, possibly because of tumoral infiltration, while it was increased in treated patients, suggesting early injury [16, 17].
Radiation Pneumonitis
In radiation therapy of the thorax, radiation pneumonitis can appear 2–8 months after treatment. Radiation lung injury occurs in proportion to the amount of lung that is exposed to doses of >20 Gy. Symptoms vary from none, when radiation is delivered to a small region of the lung, to systemic signs and symptoms of fever, dyspnea, cough, and respiratory distress syndrome, when radiation is delivered to >20% of the total lung volume. Following lung radiation there are two phases of cytokine expression: one occurring hours to days or weeks after radiation, typically returning to normal after ∼2 weeks and a second phase at 6–8 weeks, leading to pulmonary parenchymal and vascular damage [18], which may progress for 6–24 months following radiation exposure. Within 1–3 months of exposure, acute radiation lung injury can occur, manifested by dyspnea, cough, and hypoxia. Six to twenty-four months after radiation lung fibrosis can occur, leading to progressive impairment of pulmonary function. Early detection of radiation pneumonitis enables adequate treatment with a reasonable chance to prevent or limit late sequelae [19]. Clinical manifestations, chest radiographs and pulmonary function tests may help to establish an early diagnosis, but scintigraphic abnormalities can be demonstrated in the acute phase often before radiologic changes become apparent. 67Ga-citrate is also a sensitive method for the early detection of radiation pneumonitis [20], although the delineation of lung injury may be hampered due to the normal 67Ga uptake in sternum and thoracic spine.
Pentetreotide, a synthetic somatostatin analog with strong affinity for the somatostatin receptor subtypes 2 (sst2) and 5, can be labeled with 111In. Somatostatin has been shown to modulate fibroblast activation and to have antifibrotic properties also [21], although the exact mechanisms of such antifibrotic action of octreotide still remains unclear. 111In-pentetreotide may have a role in the differential diagnosis of patients with complaints after radiotherapy and in the monitoring of the response to corticosteroid therapy [19]. Some false-positive studies have been reported with increased pulmonary uptake in patients with neuroendocrine tumors which eventually was due to pulmonary fibrosis (Fig. 30.2) [22, 23]. [18F]FDG-PET has also been recently proposed to detect radiation pneumonitis [24] and may be used use as a predictor for acute radiation pneumonitis [25] (Fig. 30.3).
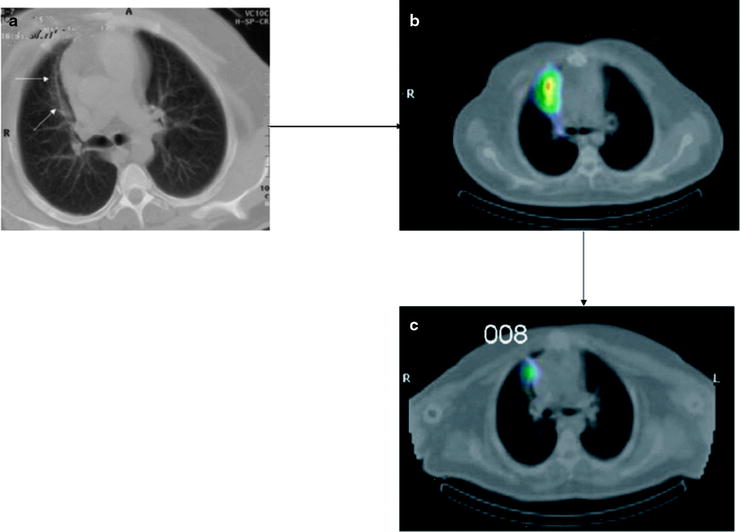
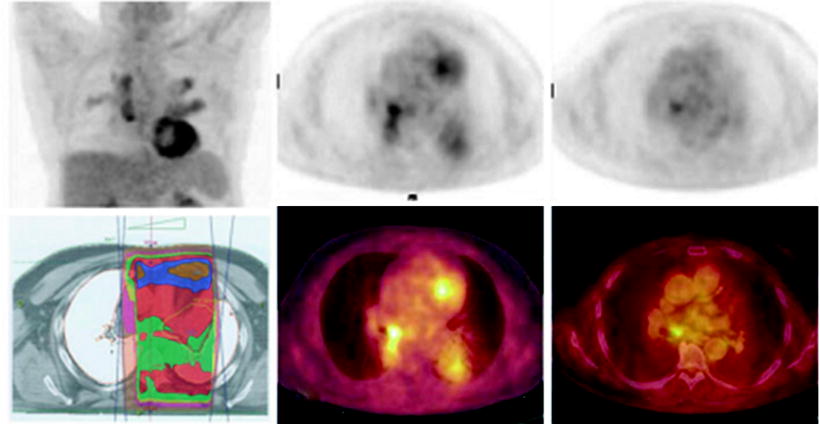
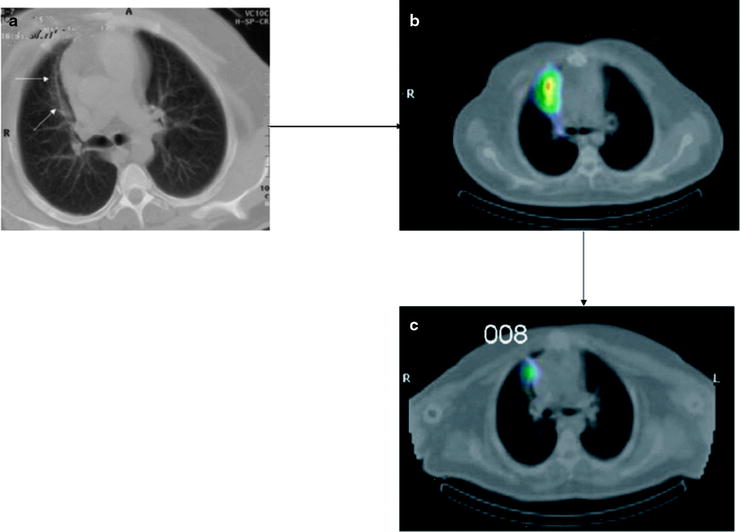
Fig. 30.2
Patient with a neuroendocrine tumor studied 10 months after radiotherapy. (a) CT. (b) Pulmonary fibrosis in the right paramediastinum shows 111In-DTPA-OC uptake on the SPECT image. (c) Decreased radiollabeled octreotide uptake 9 months later (from [22], with permission)
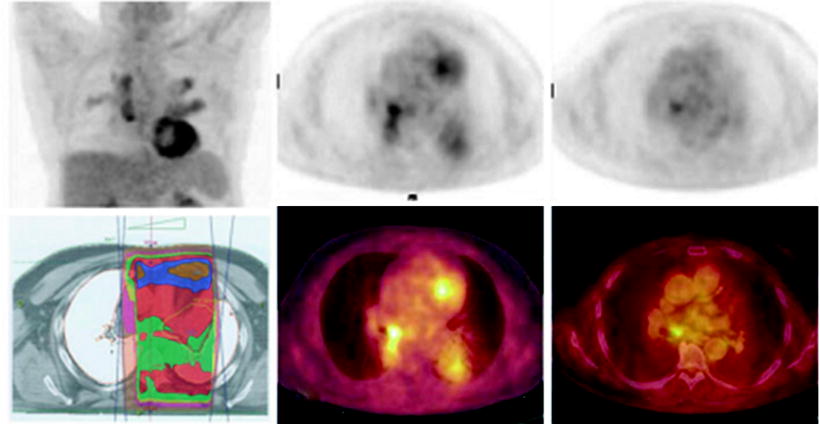
Fig. 30.3
Post-radiotherapy pneumonitis in areas of the radiotherapy treatment field (left and central panels) with resolution on the [18F]FDG-PET scan done 2 months later (right panel)
There may be significant [18F]FDG uptake in the irradiated region up to about 2 months following therapy. Persistence of significant focal activity beyond 8 weeks raises the likelihood of persistence of disease within the irradiated region.
Ventilation/Perfusion Changes Induced by Thoracic Irradiation
Radiation-induced lung damage may lead to decreased lung perfusion, lung ventilation, and diffusion of gases in the irradiated region. A decrease in pulmonary blood flow in the irradiated areas has been reported in breast cancer patients receiving radiotherapy [26]. Lung scintigraphy with 99mTc-DTPA aerosol or 99mTc-MAA (macroaggregates of albumin) have been performed to assess abnormal ventilation/perfusion patterns. A reduction in both ventilation and perfusion was observed 3 months after radiotherapy. Other radiotracers used in different studies include 133Xe, 81mKr gas, or 131I-MAA with reduced perfusion in the irradiated areas as the common finding [26–28].
Kidneys
Chemotherapeutic drugs (cisplatin and ifosfamide) can cause renal damage, while abdominal radiation in the treatment of abdominal malignancies can lead to renal nephropathy. Several radionuclide techniques have been proposed to evaluate the effect of these treatments over the kidneys.
Chemotherapy-Induced Tubular Injury
The use of nephrotoxic chemotherapeutic agents can lead to both acute and chronic toxicity, including life-threatening acute adverse effects and long-term effects that lead to progressive deterioration of the kidney function. Cisplatin, a frequently used chemotherapeutic agent in treatment of cancer, may cause renal toxicity in about one-third of patients, usually manifest as a decrease in glomerular filtration rate (GFR). The process is initiated by proximal tubular damage followed by alterations in the GFR and in the effective plasma renal flow [29]. The decrease in GFR is closely related to the dose. Cisplatin can be given in four cycles of 20 mg/m2 for 5 days without kidney damage, but a treatment dose of 40 mg/m2 daily for 5 days, results in the reduction of GFR and tubular function [30]. The strategies to prevent cisplatin nephrotoxicity are based on early detection and reduction of toxic effects using diuretics (mannitol and furosemide) or on the use of other platinum derivatives such as carboplatin and iroplatin which have less kidney toxicity. On the other hand, ifosfamide can induce Fanconi’s syndrome, a disorder of the proximal tubule of the kidney, with increasing risk at doses exceeding 50 mg/m2 [31].
Chemotherapy-induced nephropathy can be identified with quantitative measurement of GFR. Although the gold standard for this measurement utilized intravenous injection of 51Cr-labeled EDTA, 99mTc-DTPA has become the preferred radiopharmaceutical because of availability and cost. Typically three blood samples are obtained over 2–3 h and tracer clearance is calculated.
Methods proposed to evaluate tubular function include the determination of the fractional excretion of electrolytes, the renal threshold for phosphate, aminoaciduria follow-up and determination of low-molecular-weight proteins (beta-2 microglobulin). Results of such methods should be interpreted with caution before assuming proximal tubular damage because they are affected by excessive secretion of hormones, dependence on plasma concentration of proteins and urine-plasma measurement. 99mTc-DMSA scintigraphy can be used to establish tubular dysfunction [32]. It binds to proximal tubular cells. Diseases affecting the proximal tubules, such as tubular acidosis, Fanconi’s syndrome, or tubular dysfunction due to nephrotoxic drugs, inhibit 99mTc-DMSA uptake. In patients treated with ifosfamide, a significant correlation has been found between 99mTc-DMSA uptake and cumulative doses of ifosfamide (p < 0.01). Anninga et al. found that 99mTc-DMSA scintigraphy is more consistent than urine levels of beta-2 microglobulin in detecting ifosfamide-induced tubular dysfunction and concluded that the test is also able to detect subclinical injury. Other studies show that ifosfamide-induced tubular injury can be detected with 99mTc-DMSA scintigraphy [33], even before chemotherapy-associated nephrotoxicity is observed by other laboratory measurements [33–35].
Radiation Nephropathy
Radiation therapy can cause damage to the endothelial cells of the glomeruli, the parenchymal vessels, or the renal tubules leading to the radiation nephropathy [36]. Lesion progress is typically slow but resulting in complete atrophy of the irradiated renal tissue with a total loss of function. Nuclear medicine techniques offer the possibility to follow the clinical evolution of radiation nephropathy. Bone scans, using 99mTc-MDP, show abnormal uptake in the kidney of patients with radiation fields including kidneys, as for vertebral or rib malignancies. Early after radiation (over about 6–9 months) 99mTc-MDP uptake is increased. Over the succeeding 6–12 months the uptake decreases to normal or below normal levels, associated with the gradual loss of renal parenchymal function [37].
Renography with 99mTc-DTPA can be performed to assess radiation nephropathy. 99mTc-DTPA is cleared through glomerular filtration providing information about glomerular function. Simultaneous 99mTc-DTPA scans to study glomerular function and 99mTc-DMSA imaging to study tubular function show a decline in renal function at about 6 months after irradiation. Scintigraphic studies with 99mTc-DTPA and 99mTc-DMSA have been found to be more sensitive than biochemical tests to assess radiation injury. Renal toxicity has been demonstrated during treatment at radiation doses over 18 Gy. In these patients, radionuclide studies performed at regular intervals are useful for appropriate assessment of renal toxicity [37].
Some studies have described a relation between small vessel injury due to radiation and hypertension. Using 99mTc-DTPA renography with and without Captopril in patients with hypertension, it has been shown that small-vessel injury appears in hypertensive patients due to increased release of renin [38]. Other studies with 99mTc-DMSA in unilateral kidney irradiated patients have evaluated the function of each kidney. After a follow-up of 5 years, results showed that the increase of volume in the contralateral kidney was less accentuated than the increase in uptake. This suggests that the mechanism to compensate the radiation nephropathy of the contralateral kidney would be hyperfunction rather than hypertrophy [39]. These findings underline the need to perform follow-up function studies in unilateral kidney disease (Fig. 30.4).
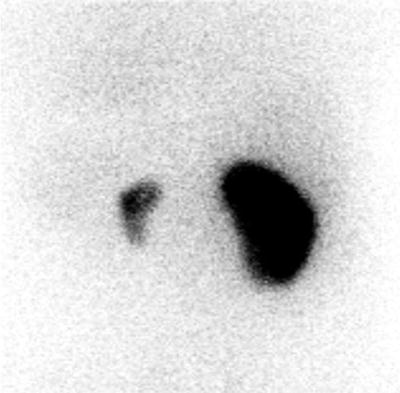
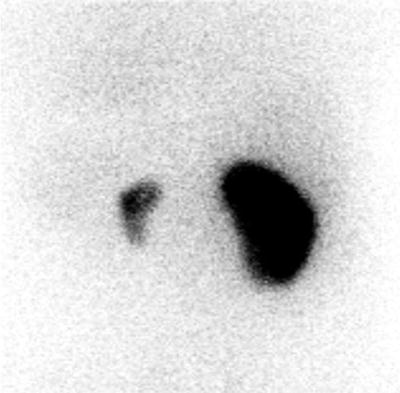
Fig. 30.4
99mTc-DMSA scintigraphy in a patient who received radiotherapy involving the left kidney. Right kidney remains normal
Digestive Tract
Nausea, vomiting, diarrhea, and enteritis often occur due to radiotherapy and chemotherapy. Nuclear medicine examinations can play a role in the detection of radiation-induced late injury on the small bowel.
Radiation Damage on Small Bowel
In cancers of the cervix, endometrium, ovary, prostate, bladder, or rectum, radiation therapy is often required. In the course of radiation therapy, rectal toxicity of grade 1–2 (Radiation Therapy Oncology Group definition = grade 1 = minor symptoms requiring no treatment; grade 2 = symptoms that respond to simple management) occurs in ∼70% of patients. The occurrence of radiation proctitis is related to the volume of the rectum exposed to doses >60 Gy. Radiation proctitis is usually self-limiting and resolves within a month after the conclusion of therapy. Damage of the small bowel is seen in 0.5–15% of the patients [40] and it is characterized by progressive cell depletion, collagen fibrosis, and obliterative vascular injury [41].
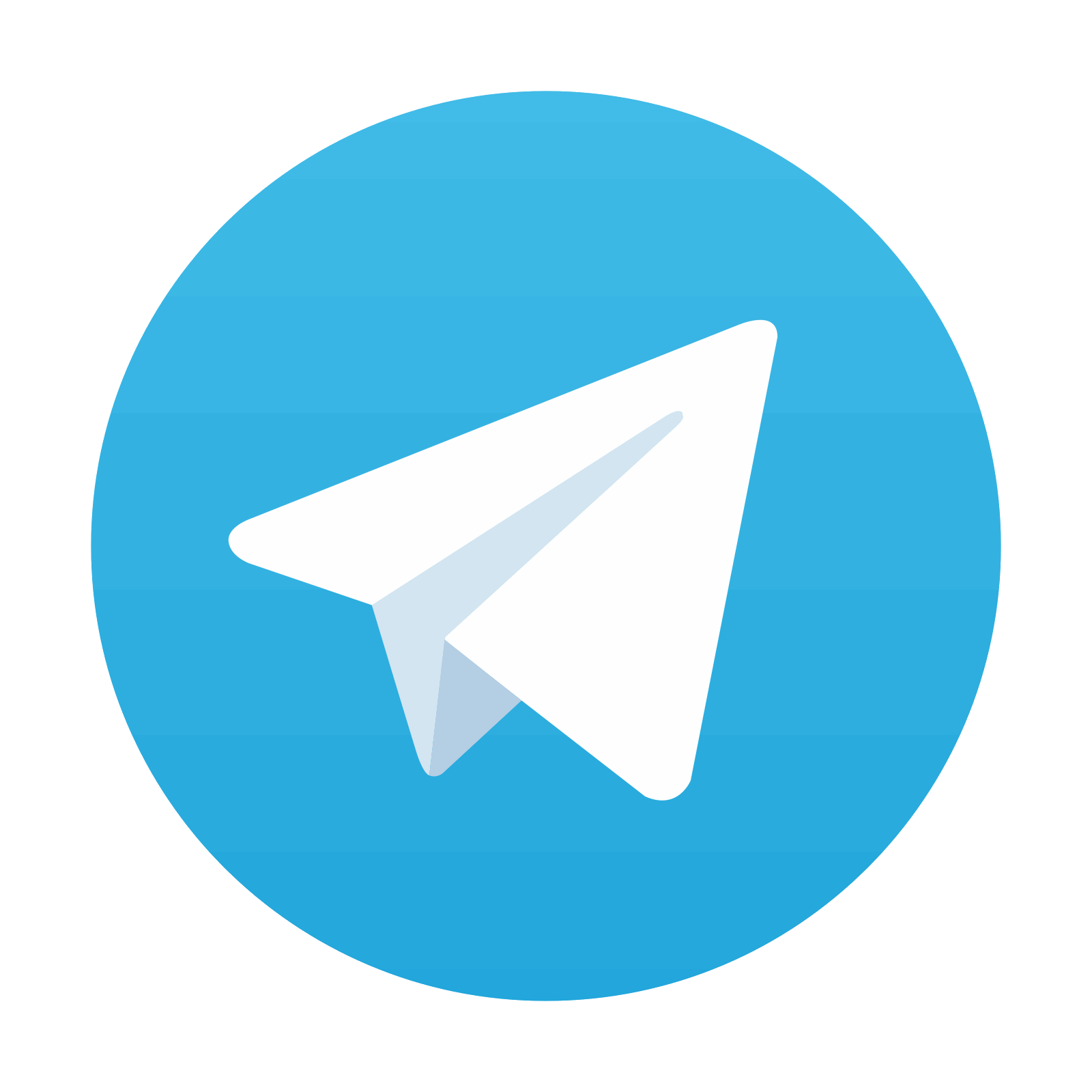
Stay updated, free articles. Join our Telegram channel

Full access? Get Clinical Tree
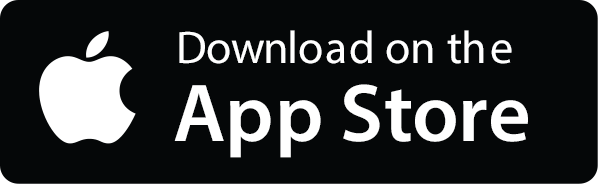
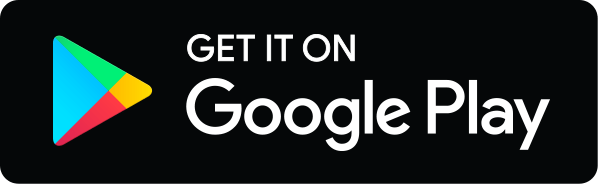