Imaging modality
Radiation dose
Key measurements
Common applications
Echocardiography (2D, 3D, exercise stress, dobutamine stress, transesophageal)
None
Wall thickness, cardiac chamber size in systole or diastole, valvular anatomy and function, intracardiac hemodynamics
Serial assessments in heart disease. Assessment of pharmacologic interventions
Carotid ultrasound
None
Intima-media thickness
Carotid atherosclerosis
Intravascular ultrasound
None
Plaque volume and characteristics within the vessel wall and degree of luminal stenosis
Coronary arteries and peripheral vascular arteries
Optical coherence tomography
None
Can differentiate between lipid, calcium, and fibrosis
Plaque characterization, vessel sizing
Cardiac CT angiography
Moderate
Still images of the heart and blood vessels, coronary calcium content
Coronary artery disease
Cardiac MRI
None
Structure and function of the myocardium
Cardiac function
Coronary angiography
Mild
Anatomy of the coronary vessels
Coronary artery disease, ventricular function, valvular assessment
SPECT
Moderate
Perfusion defects
Cardiac ischemia and function
Ischemic Heart Disease
Athlerosclerotic plaques begin to appear in the vasculature during childhood and progressively increase over time. A diagnosis of ischemic heart disease (in the absence of congenital cardiac anomalies) is made when the atherosclerotic process has advanced to the point of causing ischemic symptoms either at rest or under stress conditions. The diagnosis of coronary artery disease may also be made anatomically by demonstrating vascular stenosis >50 %. Thus, the significance of an individual’s heart disease is defined by its quantity and location. The diagnosis of ischemic heart disease or coronary artery disease may involve several imaging modalities. The initial approach to patients with suspected ischemic heart disease is to perform myocardial stress testing. Therefore, we will begin with a discussion of imaging techniques commonly employed in conjunction with stress testing.
Nuclear Medicine
Nuclear medicine encompasses both diagnostic imaging and treatment of disease and may also be referred to as molecular medicine or molecular imaging and therapeutics. Nuclear medicine uses certain properties of isotopes and the energetic particles emitted from radioactive material to diagnose or treat various pathology. Different from the typical concept of anatomic radiology, nuclear medicine enables assessment of physiology. This function-based approach to medical evaluation has useful applications in most subspecialties, notably oncology, neurology, and cardiology. Gamma cameras are used in, for example, scintigraphy, SPECT, and PET to detect regions of biologic activity that may be associated with disease. A relatively short-lived isotope, such as sestamibi, is administered to the patient. Isotopes are often preferentially absorbed by biologically active tissue in the body and can be used to identify myocardial perfusion and function. Images are acquired after collimated photons are detected by a crystal that gives off a light signal, which is in turn amplified and converted into count data.
Scintigraphy (scint) is a form of diagnostic test wherein radioisotopes are taken internally, for example, intravenously or orally. Then, gamma camera captures and forms two-dimensional images from the radiation emitted by the radiopharmaceuticals.
SPECT is a 3D tomographic technique that uses gamma camera data from many projections and can be reconstructed in different planes. A dual-detector head gamma camera combined with a CT scanner, which provides localization of functional SPECT data, is termed a SPECT/CT camera and has shown utility in advancing the field of molecular imaging.
Positron emission tomography (PET) uses coincidence detection to image functional processes. Short-lived positron emitting isotope, such as 18F, is incorporated with an organic substance such as glucose, creating F18-fluorodeoxyglucose, which can be used as a marker of metabolic utilization. This is more fully described in Chap. 1. Utilization of PET imaging allows a comparison of myocardial functional status with perfusion imaging. Areas of underperfused but functionally viable myocardium can be noninvasively identified to determine patient eligibility for invasive percutaneous or surgical revascularization strategies.
PET images can be viewed in comparison to computed tomography scans to determine an anatomic correlate. Modern scanners combine PET with a CT, or even MRI, to optimize the image reconstruction involved with positron imaging. This is performed on the same equipment without physically moving the patient off of the gantry. The resultant hybrid of functional and anatomic imaging information is a useful tool in noninvasive diagnosis and patient management.
Myocardial Stress Testing
Most exercise stress testing involves the BRUCE protocol where an individual starts exercising on a treadmill and is progressed through increasing levels of difficulty that elicit increases in heart rate due to the increased oxygen demands. In patients who are unable to exercise, pharmacologic stress can be induced with intravenous coronary vasodilating agents including adenosine and Lexiscan. When these oxygen demands are not met, there may be associated ST segment changes on the ECG, patient symptoms, or areas of underperfusion that can be detected through imaging. Monovalent cations are known to be transported into myocardial cells. Therefore, cations such as potassium, thallium, rubidium, and ammonia have been used with single-photon emission computed tomography (SPECT) imaging. Superior imaging quality has been established with technetium-99m-labeled isonitriles (sestamibi and tetrofosmin) which are the current standard of tracer for SPECT. These isotopes are injected intravenously at baseline and again at the time of peak exercise. See Fig. 9.1 for an example image using technetium-99m. They are rapidly taken up into the myocardium. Areas where the tracer is not taken up represent either abnormal regional blood flow or previous infarction. By comparing the baseline scan to the subsequent scan at peak load, one can distinguish between the two. Stenotic arteries are not able to vasodilate to the same degree as normal vessels which lead to relative differences in myocardial perfusion.
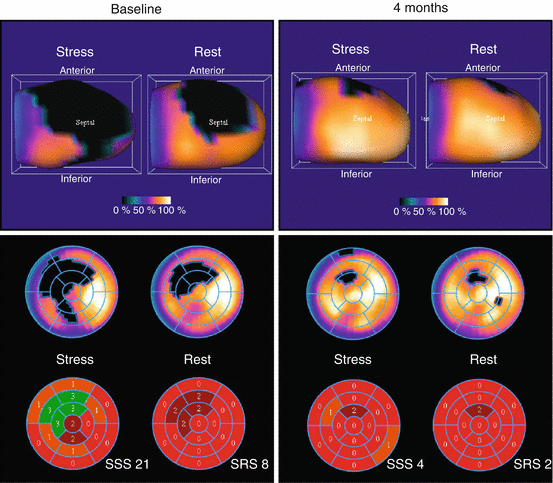
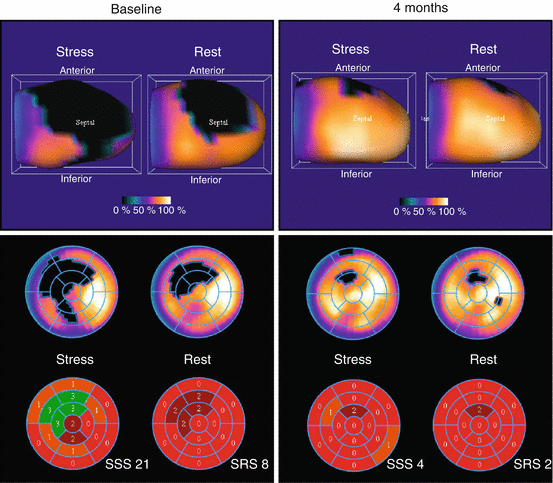
Fig. 9.1
Cardiac SPECT imaging. Technetium-99m sestamibi single-photon emission computed tomography (SPECT) scintigraphy looking at myocardial perfusion. Patient with acute anterior MI presented an important perfusion defect of the anteroseptal and apical territories (top left). The bull’s-eye image (bottom left) combining the 17 segments revealed an important ischemic territory with summed stress score (SSS) of 21 and a summed rest score (SRS) of 8. The summed difference (SDS = SSS–SRS) was 13 (not shown), indicating a significant redistribution (reversible perfusion defect). Four months later (rights), perfusion defect and ischemia were significantly reduced as revealed by stress and rest SPECT (Used with kind permission of Springer Science + Business Media from Mansour et al. [6])
A normal exercise SPECT is associated with a rate of MI or cardiac death of 0.65 % annually, while an abnormal test is associated with a 4.30 % annual rate. Normal pharmacologic nuclear myocardial perfusion scans are associated with a 1.78 % annual risk of MI or cardiac death, while an abnormal test yields a 9.98 % annual rate [1]. These differences are attributed to greater comorbidities and the associated increased atherosclerotic disease burden in the pharmacologically diagnosed group. While the rates of MI/cardiac death with a negative SPECT are relatively low, due to the large numbers of these tests being performed with approximately ten million being performed annually in the United States, the event rate per million negative procedures is 6,500 for exercise SPECT and 17,800 for pharmacologic SPECT. One reason for this is that nonobstructive plaques vulnerable to rupture will not be detected.
If one is interested in performing a clinical trial for patients at risk for cardiac events due to coronary artery disease, is a positive exercise or pharmacologic SPECT a useful inclusion criterion? A meta-analysis comparing SPECT to coronary angiography where coronary artery stenosis >50 % was used to define CAD, the sensitivity, specificity, and diagnostic accuracy for SPECT was 82, 76, and 83 %, respectively [2]. PET yielded the most favorable results with sensitivity of 91 %, specificity of 89 %, and diagnostic accuracy of 89 % making it a preferred noninvasive test for the diagnosis of coronary artery disease.
Cardiac Positron Emission Tomography (PET)
Cardiac positron emission tomography (PET) is a form of diagnostic imaging in which patients are evaluated using a PET scanner after intravenous injection with a radioisotope. Although several isotopes have been used for cardiac PET imaging, the most widely employed in clinical practice are rubidium-82 and nitrogen-13 ammonia.
The requirements to perform cardiac PET imaging include:
Facility: taking into consideration clinical workflow, as well as regulatory requirements such as requisite shielding from radiation exposure.
Capital equipment: PET or PET/CT scanner.
Radiopharmaceutical: rubidium-82 generator system or close access to cyclotron produced isotopes such as nitrogen-13 ammonia.
Personnel: including specialty trained physician, radiation safety, physics, nursing, and technologist support.
Operations: stress test monitoring, as well as emergency response equipment, processing and review workstations, and administrative and support personnel are additional considerations.
This form of diagnostic imaging has traditionally been perceived as cost prohibitive in comparison to general nuclear medicine cardiac stress testing using single-photon emission computed tomography (SPECT). However, due to significant gains in access to scanners, related to the widely accepted role of PET/CT in clinical oncology, cardiac PET is likely to become more widely available, particularly given various clinical and technical advantages that might make this a potential test of choice in the diagnosis of coronary artery/heart disease. An example set of images are shown in Fig. 9.2.
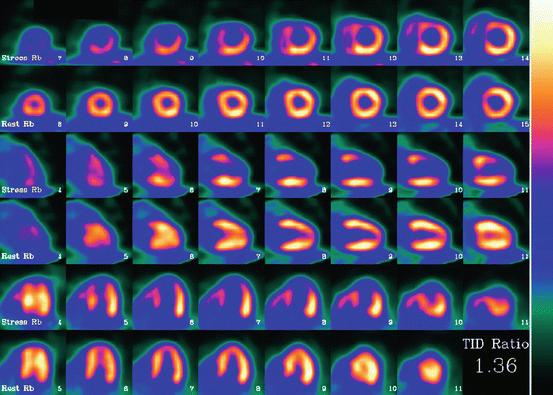
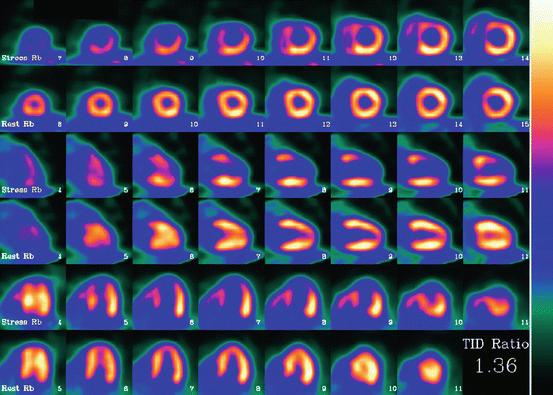
Fig. 9.2
Cardiac positron emission tomography. Abnormal rubidium-82 PET myocardial perfusion scan at stress and rest. Short axis (top rows), vertical long axis (middle rows), and horizontal long axis (bottom rows) slices demonstrate severe and extensive ischemia involving the anterior, apical, and septal walls (Used with kind permission of Springer Science + Business Media from Fox and Strauss [7])
Computed Tomography Angiography (CTA)
CT combines the use of X-rays with computerized analysis of the images. Beams of X-rays are passed from a rotating device through the area of interest in the patient’s body from several different angles to obtain projection images, which then are assembled by computer into a three-dimensional picture of the area being studied. See Chap. 1 for more details. Contrast material is injected in order to better visualize the coronary vessels. With the advent of subsecond rotation combined with multi-slice CT (up to 128-slice), high resolution and high speed can be obtained at the same time, allowing excellent imaging of the coronary arteries (cardiac CT angiography). Images with an even higher temporal resolution can be formed using retrospective ECG gating. In this technique, each portion of the heart is imaged more than once while an ECG trace is recorded. The ECG is then used to correlate the CT data with their corresponding phases of cardiac contraction. Once this correlation is complete, all data that were recorded while the heart was in motion (systole) can be ignored, and images can be made from the remaining data that happened to be acquired while the heart was at rest (diastole). In this way, individual frames in a cardiac CT investigation have a better temporal resolution than the shortest tube rotation time.
Because the heart is effectively imaged more than once (as described previously), cardiac CT angiography results in a relatively high radiation exposure around 12 millisieverts (mSV). Newer prospective gating techniques and imaging protocols in combination with the development of larger imaging scanner 128-slice and higher are allowing significant reduction in radiation exposure. Methods are available to decrease this exposure, however, such as prospectively decreasing radiation output based on the concurrently acquired ECG (also known as tube current modulation). This can result in a significant decrease in radiation exposure, at the risk of compromising image quality if there is any arrhythmia during the acquisition. The significance of radiation doses in the diagnostic imaging range has not been proven, although the possibility of inducing an increased cancer risk across a population is a source of significant concern. This potential risk must be weighed against the competing risk of not performing a test and potentially not diagnosing a significant health problem such as coronary artery disease.
Currently, it appears that the greatest utility of cardiac CT lies in excluding significant coronary artery disease rather than confirming its presence. This is because the test has a high sensitivity (greater than 90 %) and thus a negative test result means that a patient is very unlikely to have coronary artery disease and can be worked up for other causes of their chest symptoms. This is termed a high negative predictive value. A positive result is less conclusive and often will be confirmed (and possibly treated) with subsequent invasive angiography. The positive predictive value of cardiac CTA is estimated at approximately 82 % and the negative predictive value is around 93 %. A meta-analysis at the patient level comparing computed tomography angiography to invasive coronary angiography in the diagnosis of arterial stenosis between years 2006 and 2009 determined a sensitivity of 98.2 % and specificity of 81.6 %. The median positive predictive value was 90.5 % and negative predicted value was 99.0 % [3]. A retrospective study compared males and females at low (defined as risk <30 %) and intermediate (defined as risk of 30–90 %) risk of CAD assessed CTA against invasive coronary angiography. Women at low and intermediate risk had a sensitivity of 97 and 99 %, a specificity of 79 and 72 %, a positive predictive value of 80 and 83 %, and a negative predictive value of 97 and 98 %, respectively. Values for men were comparable with sensitivity of 100 and 99 % and negative predictive values of 100 and 99 %, respectively [4]. Thus, a negative CTA is a very good noninvasive manner to detected coronary artery disease. The diagnostic accuracy of the modality is now accepted and the field has shifted towards reducing the radiation dose associated with the procedure.
Dual-source CT scanners, introduced in 2005, allow higher temporal resolution by acquiring a full CT slice in only half a rotation, thus reducing motion blurring at high heart rates and potentially allowing for shorter breath-hold time. This is particularly useful for ill patients who have difficulty holding their breath or who are unable to take heart-rate-lowering medication.
The speed advantages of 64-slice MSCT have rapidly established it as the minimum standard for newly installed CT scanners intended for cardiac scanning. Manufacturers are now actively developing 256-slice and true “volumetric” scanners, primarily for their improved cardiac scanning performance.
The latest MSCT scanners acquire images only at 70–80 % of the R-R interval (late diastole). This prospective gating can reduce effective dose from 10 to 15 mSv to as little as 1.2 mSv in follow-up patients acquiring at 75 % of the R-R interval. Effective doses at a center with well-trained staff doing coronary imaging can average less than the doses for conventional coronary angiography.
CT Angiography of Vascular Structures
CT angiography remains the mainstay in imaging of the thoracic and abdominal aorta. This technique provides an accurate measurement of segmental regions of the aorta. Three-dimensional reconstruction can also be performed with automated software. Image analysis allows precise measurement for procedure planning, device measurement for interventional placement, and endovascular graft placement for thoracic and abdominal aneurysm.
Serial CT angiograms of the aorta allow an effective method for monitoring progression of disease targeting timing of interventional therapies. In addition, serial studies are included in protocols to monitor the short- and long-term effectiveness of therapies and post-procedural complications. Examples include stent graft endoleaks and graft migration.
In addition, quantitative analysis of vascular plaque presence and its extent have been utilized as surrogate endpoints in the effectiveness of pharmacotherapy in clinical trials.
Prognostic Implications of an Abnormal Coronary CT Angiogram (CCTA)
The clinical outcomes published to date for coronary CT angiogram (CCTA) are comparable in trial size, patient population, and median follow-up to those with SPECT. A CCTA indicating no coronary artery disease is associated with a 0.15 % annual risk of overall mortality. In the same study there were no deaths related to coronary artery disease during the follow-up period [5] (The annualized mortality rate for nonobstructive CAD was approximately 1 % and for obstructive CAD was approximately 4 %.) Thus, CCTA enables one to predict three distinct levels of risk and importantly identifies a population that is at very low risk of a cardiac event. Thus, for the purpose of identifying individuals at risk for a first MI, CCTA is the imaging modality to identify the burden of coronary artery disease. See Fig. 9.3 for plaque identification with CCTA.
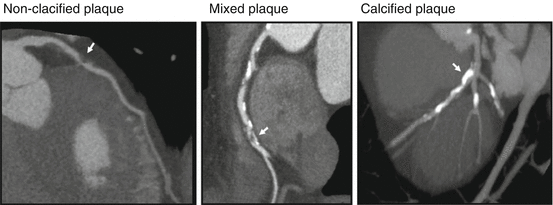
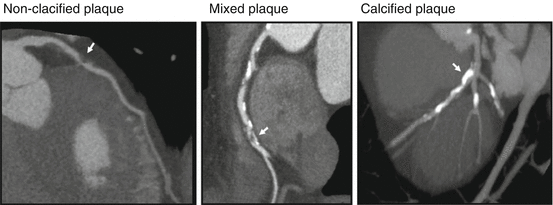
Fig. 9.3
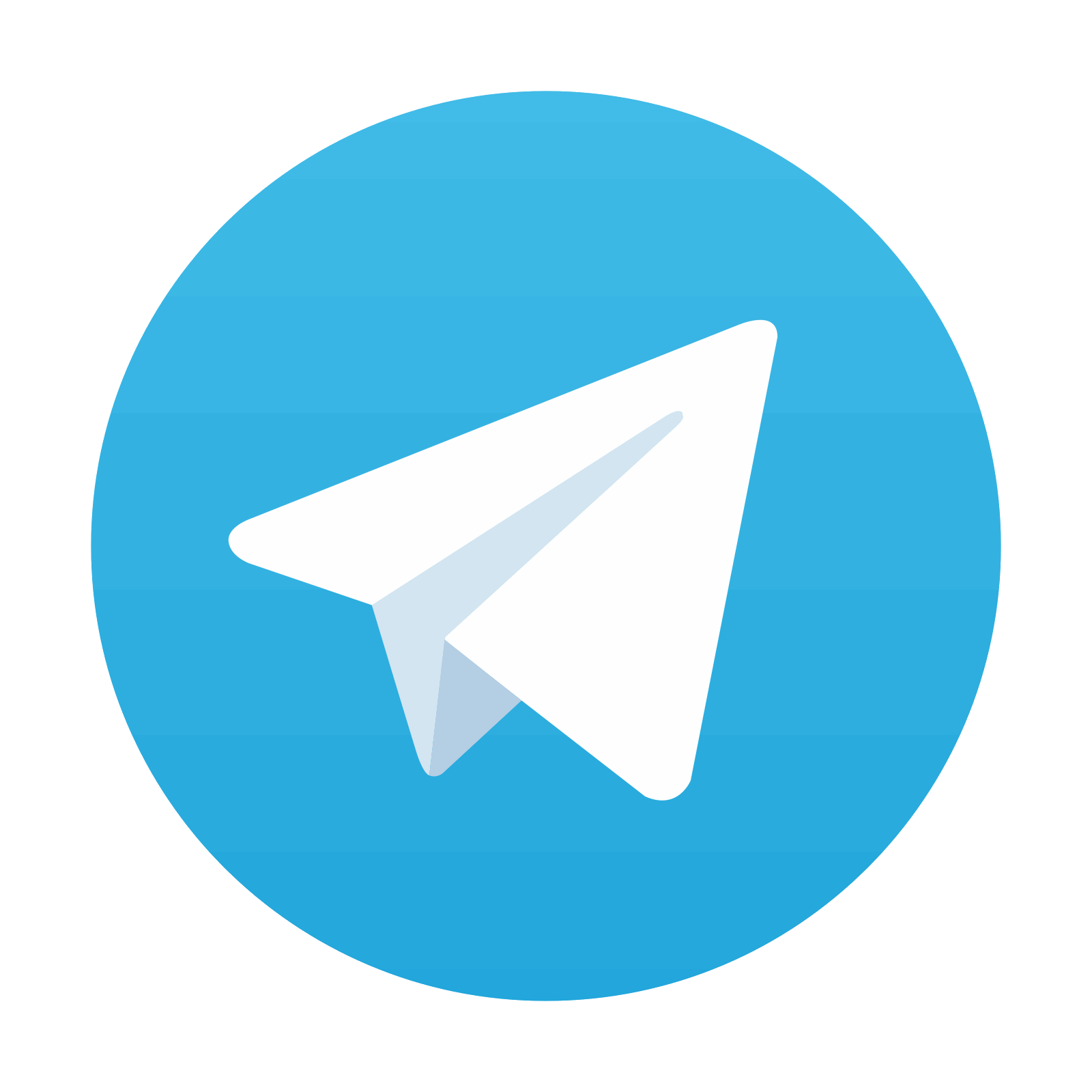
Computed tomography angiography. Plaque composition assessed with CTA. Curved multi-planar reconstructions showing three distinct plaque characteristics observed on CTA with noncalcified plaque (arrow, left panel), mixed plaque (arrow, middle panel), and calcified plaque (arrow, right panel) (Used with kind permission of Springer Science + Business Media from van Werkhoven et al. [8])
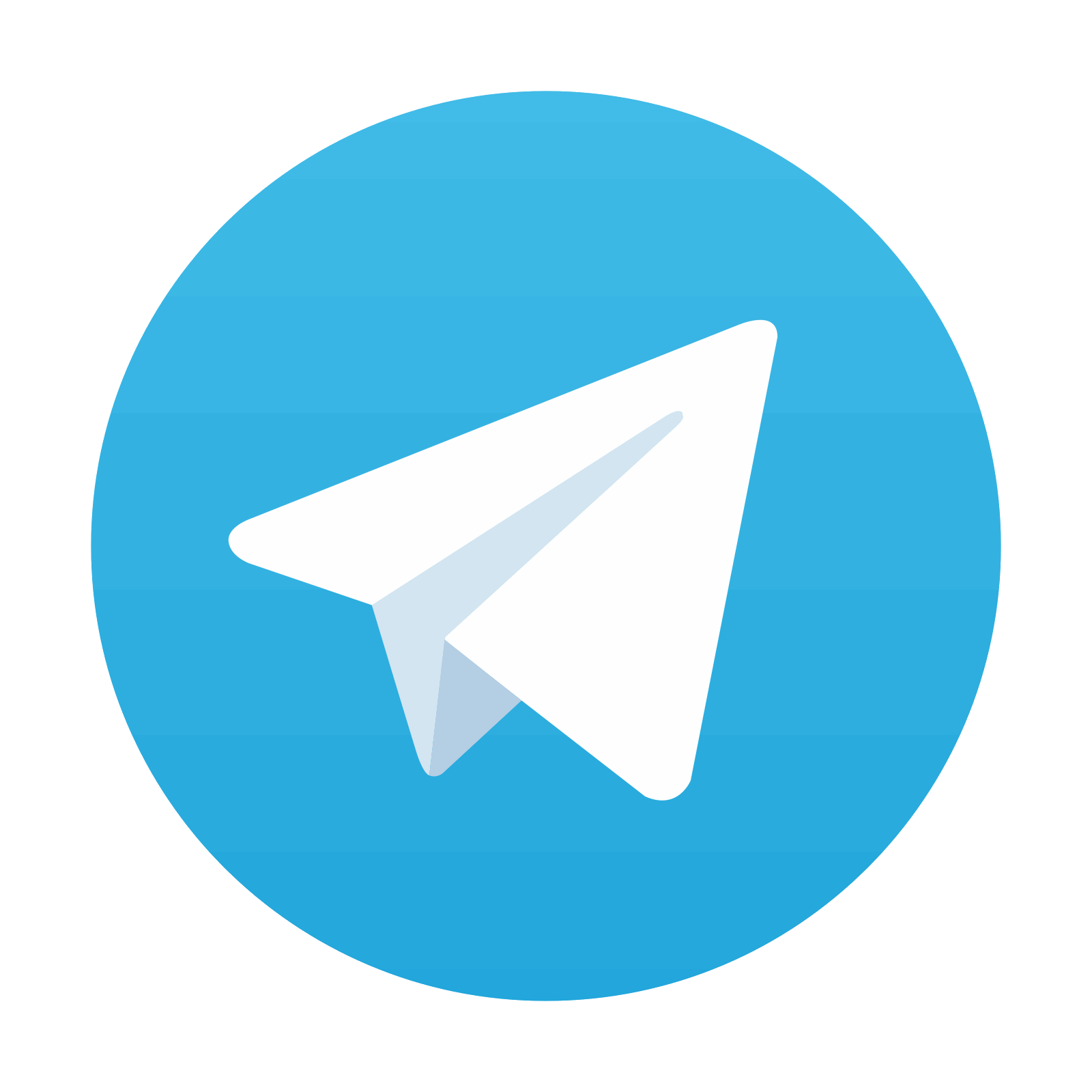
Stay updated, free articles. Join our Telegram channel

Full access? Get Clinical Tree
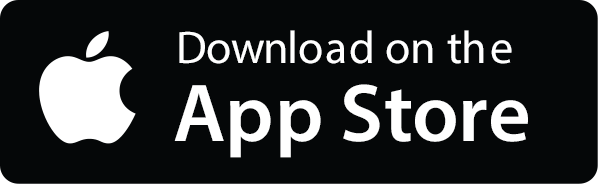
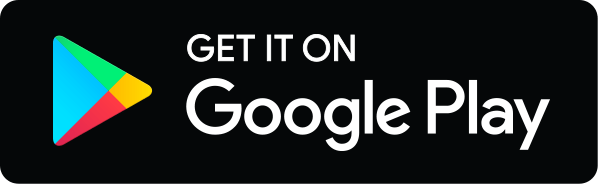
