Abstract
This chapter describes the emerging role of cardiovascular magnetic resonance (CMR) in the assessment of neonatal hemodynamics. While at the time of publication this approach remains in its infancy, the technique has begun to demonstrate its value in providing highly detailed insights into the pathophysiology of the neonatal circulation.
CMR assessments in adults are now acknowledged as the gold standard clinical assessments of ventricular function, cardiomyopathy, myocarditis, and complex congenital heart disease. The use of brain magnetic resonance imaging (MRI) is similarly the standard of care for the assessment of newborns with encephalopathy.
CMR assessments of neonatal hemodynamic function are neither simple nor inexpensive, but they can be highly accurate and entirely noninvasive. This chapter summarizes areas of neonatal hemodynamics where there is significant residual uncertainty over pathophysiology and management; provides a background to application of CMR in adult and newborns; discusses the current and emerging CMR techniques; and describes areas where CMR has already advanced understanding of developmental cardiovascular physiology and may in the future contribute to changes in the care of critically ill preterm and term neonates with cardiovascular compromise.
Our hope is that as co-location of MRI technology with neonatal intensive care units becomes more common, CMR will fulfill its significant promise to advance clinical research and support improvements in mortality and morbidity in the vulnerable newborn through enhanced hemodynamic monitoring and support.
Keywords
blood flow, cardiac output, echocardiography, magnetic resonance imaging, neonate
- •
Cardiac magnetic resonance (CMR) imaging techniques provide noninvasive assessments of the newborn circulation with high accuracy and repeatability.
- •
Cine CMR produces three-dimensional assessments of chamber volumes and myocardial mass.
- •
Phase contrast CMR can quantify blood flow in any major vessel.
- •
CMR techniques have provided normative ranges for neonatal left and right ventricular development, quantification of patent ductus arteriosus (PDA) shunt volume, and guided optimization of ultrasound techniques.
Acknowledgments
We are grateful to Prof. David Edwards, Prof. Reza Razavi, Prof. Jo Hajnal, Miss Giuliana Durighel, Dr. Kathryn Broadhouse, Dr. Anna Finnemore, Dr. David Cox, and the staff of Queen Charlotte’s and Chelsea Hospital and St. Thomas’s Hospital Neonatal Units for their assistance with the project.
This chapter describes the emerging role of cardiovascular magnetic resonance (CMR) in the assessment of neonatal hemodynamics. While at the time of publication this approach remains in its infancy, the technique has begun to demonstrate its value in providing highly detailed insight into the pathophysiology of the neonatal circulation. CMR is also increasingly utilized for the assessment of structural congenital heart disease in infancy, but the reader is directed to other excellent reviews for discussions of this topic.
CMR in adults is now acknowledged as the gold standard clinical assessment of ventricular function, cardiomyopathy, myocarditis, and complex congenital heart disease. The use of magnetic resonance imaging (MRI) in the brain is similarly the standard of care for assessment in newborns with encephalopathy, pathology of the premature brain, and developmental and genetic abnormalities.
CMR assessments of neonatal hemodynamic function are neither simple nor inexpensive, but they can be highly accurate and noninvasive. This chapter summarizes areas of neonatal hemodynamics where there is significant residual uncertainty over pathophysiology and management; provides a background to application of CMR in adult and newborns; discusses the current and emerging CMR techniques; and describes areas where CMR has already advanced understanding of developmental cardiovascular physiology, and where in the future it may contribute to changes in the care of critically ill preterm and term neonates with cardiovascular compromise.
Our hope is that as co-location of MRI technology with neonatal intensive care units (NICUs) becomes more common, CMR will fulfill its significant promise to advance clinical research and support improvements in mortality and morbidity in the vulnerable newborn through enhanced hemodynamic monitoring and support.
Current Understanding of Neonatal Hemodynamics
As discussed in a number of chapters in this book, the most prematurely born infants remain at high risk of death and disability. While in the past respiratory disease was the primary cause of death, high quality research has improved preterm respiratory care, such that an increasing proportion of deaths occur due to episodes of sepsis or necrotizing enterocolitis, where impaired myocardial function and failing peripheral vascular control often make circulatory failure the final mechanism of death.
Circulatory failure is also central to the pathophysiology of the key morbidities of premature birth. The long-term sequelae of preterm birth carry an estimated annual socioeconomic burden of greater than £3 billion in the UK and greater than $26 billion in the United States. Failing cardiac function causes cerebral hypoperfusion, and episodes of low cerebral blood flow are central to the pathophysiology of preterm brain injury, which causes long-term disability (see Chapter 7, Chapter 26 ). Limitations in clinicians’ ability to adequately assess circulatory function impair both the ability to tailor care in individual infants and the conduct of adequate trials of the impact of potential therapies. Improving circulatory management is therefore a research priority in preterm infants.
Circulatory Physiology and Assessment
All circulatory function relies on the interplay of preload, contractility, and afterload (see Chapter 1 ). The preterm transitional circulation is further complicated by the persistence of fetal shunt pathways (foramen ovale and ductus arteriosus), which may significantly alter circulatory dynamics. A robust assessment of the newborn circulation therefore needs to quantify preload, contractility, afterload, and systemic and organ perfusion.
Unfortunately, the current methods fall short of this ideal; in particular, routine clinical monitoring of circulatory status in the neonatal unit still relies heavily on arterial blood pressure. However, systemic arterial blood pressure is the product of systemic blood flow and systemic vascular resistance and cannot itself distinguish between the two. While clinicians presumably feel that monitoring systemic blood pressure is a screening tool for low systemic perfusion, in fact blood pressure in itself is at best weakly predictive of volume of blood flow, and some studies have suggested no or even an inverse relationship between blood pressure and flow in newborn preterm infants. Other clinical assessments, such as capillary refill time or volume of urine output, also have limited value in indicating circulatory health.
A range of technologies are now entering “prime time” for hemodynamic assessment in the neonatal unit. Point of care cardiac ultrasound (see Chapter 10, Chapter 11, Chapter 12, Chapter 13 ) leads the way and has produced important advances in the understanding of circulatory physiology, with an increasing role in the assessment of circulatory status at the bedside. Established techniques are being improved upon, and newer modalities are emerging and undergoing optimization. Cardiac ultrasound currently provides only snapshots of hemodynamic status, so continuous monitoring techniques such as impedance monitoring (see Chapter 14 ) and near-infrared spectroscopy (see Chapter 17, Chapter 18 ) are also reaching the point of clinical utility. Finally, the evolving systems capable of real-time, comprehensive, and mostly noninvasive, continuous cardiorespiratory and neurocritical care monitoring and data acquisition at the bedside provide an approach, with the potential of addressing many of the presently unanswered questions and unresolved controversies (see Chapter 21 ). CMR will again play a key role in helping improve our understanding of the interplay among important hemodynamic factors during transition and in pathologic conditions, in addition to assessing the response to various treatment approaches in neonates with hemodynamic compromise.
A key step for all new modalities is robust validation in the population of interest. In the current absence of a gold standard assessment of cardiac function in the premature infant, CMR has a role as a validation tool—its accuracy and repeatability in newborns are consistently outperforming that of cardiac ultrasound, near-infrared spectroscopy, and impedance monitoring. Outside the early newborn period (where transfer of a sick newborn to an MRI scanner, even one located on the NICU, is challenging), the accuracy of CMR in quantifying volume of blood flow and myocardial mass is providing valuable insights into circulatory physiology and cardiac development.
Cardiac Magnetic Resonance in Adults and Newborns
A comprehensive discussion of magnetic resonance (MR) physics is beyond the scope of this textbook, but a few basic principles may be pertinent. The underlying principle of MR relies upon the fact that certain atomic nuclei possess an intrinsic magnetic moment, if they contain an odd number of protons or neutrons. This is also identified as nuclei having the property of nonzero spin. When placed in a strong magnetic field, these nuclei align either with, or against, the field; a small proportion align with the field, leading to a net magnetization. The effect is proportional to the field strength and ultimately leads to the level of signal available in MRI—hence the drive for increasing the field strength of magnets in clinical imaging. The resulting net magnetization rotates or “precesses” around the axis of the magnetic field at a set rate depending on the field strength and the gyromagnetic ratio of the nuclei. This rate of precession (or resonance) is known as the Larmor frequency.
The aligned equilibrium magnetization can be manipulated by radiofrequency (RF) pulses applied at the resonant frequency; subsequently, the perturbed magnetization will then emit its own RF signal as it rotates in the magnetic field. This is measured using closely coupled receiver coils. Images are produced by localizing the abundance of spins by the use of magnetic field gradients. Hydrogen atoms consisting of a single proton, provide the strongest signal of all relevant atoms and are also the most abundant in the body. Image contrast may be based on the concentrations of protons or how they interact with their magnetic environment, which is detected by changes in their relaxation rate. In-flow effects can provide additional contrast; this is particularly useful to CMR, where blood flow provides “fresh” magnetization compared with saturated signals of more static tissue. Alternatively, in phase contrast (PC) MR, spins are “labeled” according to their velocity by applying additional encoding magnetic field gradients, producing a signal dependent on their velocity through the direction of the applied field, analogous to that achieved with Doppler ultrasound.
Cmr In Adults
Cardiac MR techniques have significantly advanced our understanding of cardiovascular physiology and pathophysiology in adults and are now considered the gold standard functional assessment tool. These noninvasive assessments of cardiac health are now being gained faster, in more detail, and with greater sophistication than ever before. The range of techniques available have been summarized in a number of recent reviews. The key benefits of CMR in the adult population are the ability to quantify the component factors of circulatory function and the improved repeatability over echocardiography for the assessment of quantitative measures. Critically, improved repeatability also translates into a reduction in the patient numbers required to prove a hypothesis in research studies.
Cmr In Newborns
It is clear that functional CMR will not become a routine clinical assessment tool for the sick newborn infant in the foreseeable future; imaging acutely ill children and infants remains challenging. It is more feasible, especially for more stable newborns, when an MR scanner is located within the neonatal unit. A growing number of centers are installing either dedicated neonatal or full-size adult scanners, bringing “point of care MRI” closer to reality. Our group’s patient care system for very low birth weight infants undergoing MRI has been described, demonstrating that MR scans can safely be performed in the newborn population while maintaining respiratory, circulatory, and thermal stability. We have now performed cardiac MR examinations in more than 350 newborn preterm and term infants without any adverse events. Functional CMR images have been successfully obtained in infants weighing less than 600 g and at 25 weeks’ corrected gestation. For the research data presented that follows, all infants were studied purely for research purposes, with signed parental consent and ethics committee or institutional review board approval. Scans were carried out using dedicated neonatal scanners installed within the Neonatal Intensive Care Units at Hammersmith and St. Thomas’s Hospitals (Philips 3.0 Tesla Achieva systems [Best, Netherlands]).
In all cases, cardiac MR images are obtained after infants have been allowed to fall into a natural sleep after a feeding, with careful swaddling. Neither sedative medication nor anesthesia is used. Infants are scanned with oxygen saturation, heart rate, and continuous temperature monitoring; a pediatrician/neonatologist or trained neonatal nurse should be in attendance throughout each scan. Protection from acoustic noise is achieved by applying moldable dental putty to the ears and covering them with neonatal ear muffs (Natus Minimuffs, Natus Medical Inc., San Carlos, California). The application of an acoustic hood can further reduce disturbance from scanner noise. Scans can be performed free-breathing or with the provision of mechanical ventilation, nasal continuous positive airway pressure, or high flow or low flow oxygen therapy, as clinically indicated. In older subjects, respiratory motion causes significant image degradation requiring breath-holds, the use of image navigators to coordinate acquisition with diaphragmatic movement, or advanced postprocessing to account for respiratory motion. However, in neonates, high quality cardiac images are obtainable without the need for sedation/anesthesia or respiratory navigation, presumably due to the relatively small degrees of diaphragmatic excursion. Performing scans free-breathing both improves the acceptability of the technique and limits scan acquisition times. The gating of scan acquisitions to the infant’s cardiac cycle remains vital for adequate imaging. This is best achieved using a four-lead vector cardiogram (VCG), as a standard electrocardiogram (ECG) can be significantly degraded by the magnetic fields in the MRI environment. VCG gating can be carried out prospectively or retrospectively. In general, retrospective gating is preferred, as this technique allows imaging throughout the entire cardiac cycle and gives additional flexibility to adapt to the variable heart rate seen in the newborn.
Adult CMR studies are increasingly performed at higher (3.0 Tesla) field strengths. Discussion on the specific challenges faced when imaging at higher field strengths is outside the scope of this chapter. However, in brief, the main drive to higher field systems comes from the increased signal offered as field strength increases, which may confer a substantial benefit for imaging small neonates. The major challenges faced in performing CMR in neonates arise from the need to significantly increase image resolution, both spatially, due to the size of the heart, and temporally, due to the rapid heart rates. These requirements place significant increased demands on the scanner hardware. In addition, the standard imaging protocols applied to adults may not be directly transferable, and thus require modification to be used for scanning small neonates. The initial process of image optimization that we have undergone to improve image quality has previously been described.
Current and Emerging Cardiovascular Magnetic Resonance Techniques
Cine Cmr
Cine CMR plays a central role in any CMR assessment by producing time-resolved images of the heart that can be used to analyze cardiac function. Typically, in adults, an MR acquisition method known as steady-state free procession (SSFP) is used because it provides images with excellent contrast between blood and muscle while also having very high signal-to-noise efficiency. The technique applies rapid repetitive RF pulses and subsequently acquires data, referenced relative to the VCG trace to allow retrospective reordering, that can produce dynamic images of the beating heart. However, applying this technique at higher fields (3.0 T and above) is challenging and in particular requires the maintenance of a very uniform background magnetic field through careful shimming of field inhomogeneities. Cine images acquired in the newborn infant typically have a temporal resolution of around 10 to 20 milliseconds, a spatial (in-plane) resolution of 1 mm, a slice thickness of 4 to 5 mm, and can be acquired in around 30 seconds per slice, with multiple averages. Scans can be acquired in any imaging plane, with example four-chamber and short-axis views shown ( Fig. 15.1 ).
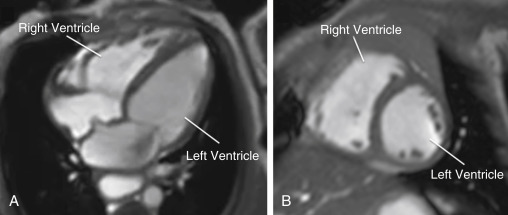
However, the key utility of cine CMR comes from acquiring a stack of contiguous short axis images to encompass the entire volume of the left and right ventricles. Endocardial and epicardial borders can be traced at end-diastole and end-systole at each level of the stack of images to reconstruct three-dimensional (3-D) models of ventricular function using freely available software packages (e.g., Segment, http://medviso.com/products/segment/; Fig. 15.2 ). These models are constructed directly from imaging of the whole heart without the assumptions on ventricular geometry, which weaken the equivalent two-dimensional echocardiographic estimations.
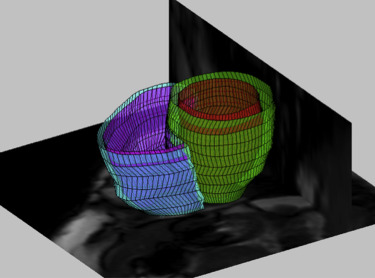
Cine CMR techniques can therefore provide data on cardiac preload (end-diastolic volume), cardiac contractility (ejection fraction), cardiac output (stroke volume), and myocardial volume from these 3-D models. In addition to enabling assessments of cardiac preload and ejection fraction, which have not previously been readily assessed by echocardiography, our data demonstrate that these quantitative CMR measures have significantly improved repeatability compared with traditional echocardiographic methods (discussed later).
Pc Cmr
PC CMR techniques require additional flow encoding gradients alongside a reference acquisition collected in quick succession. Any static tissue will have the same phase between acquisitions. Moving objects experience a different magnetic field between the flow encoded and reference acquisition, and therefore accumulate a phase directly (and quantifiably) proportional to the velocity of tissue or (more often) blood. Utilizing this technique with VCG-synchronized cine (time-resolved) acquisition means that the flow of blood can be directly measured throughout the cardiac cycle. PC slices can be again placed in any orientation, allowing the quantification of volume of flow in any large blood vessel. PC images acquired in the newborn infant have a temporal resolution of 10 to 20 milliseconds, and with sequence optimization and dedicated receiver coil technology, images can now be acquired with a spatial (in-plane) resolution of 0.4 to 0.6 mm and a slice thickness of 4 mm in around 90 seconds.
The particular value of PC imaging in the neonate lies in quantification of flow at multiple points in the circulation. The persistence of fetal shunt pathways in the preterm neonate means that neither left nor right ventricular output represents true systemic or pulmonary perfusion. Cardiac MR allows quantification of flow in the superior vena cava (SVC) and descending aorta (DAo), both of which are considered to be markers of true systemic perfusion in the preterm neonate. In addition, flow can be assessed in the internal carotid and basilar arteries, the sum of which equates to total brain blood flow.
PC MRI is a highly validated technique in the adult. Our data also demonstrate that quantification of SVC and DAo flow with PC CMR has significantly improved repeatability compared with prior echocardiographic cohorts (discussed later). This may be because echocardiographic techniques measure diameter, which then has to be squared to estimate the area and multiplied by the measured velocity time integral of blood flow, producing a potential multiplication of errors. In contrast, PC MRI techniques measure velocity in each voxel across the vessel area, and total flow is estimated by averaging the signal over each of these voxels, so potentially smoothing out errors. The sum of SVC and DAo flow volumes correlates closely with left ventricular (LV) output when the ductus is closed, providing further validation of the techniques and supporting the notion that both SVC flow and DAo flow are reasonable surrogates for systemic perfusion.
Three-Dimensional Pc Cmr
While two-dimensional (2-D) PC techniques allow quantification of flow within a single blood vessel, 3-D techniques allow for the visualization of flow in entire regions of the body. Specialist postprocessing software is commercially available, which allows the user to trace the path of a notional bolus of blood from within any vessel, throughout the cardiac cycle. We have applied these techniques in newborn infants and utilized MRI flow software (GTFlow, GyroTools LLC, Zurich, Switzerland) to visualize flow in the aortic arch and pulmonary arteries ( Fig. 15.3 ).
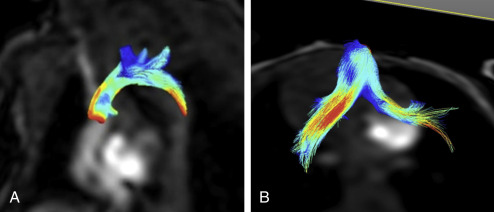
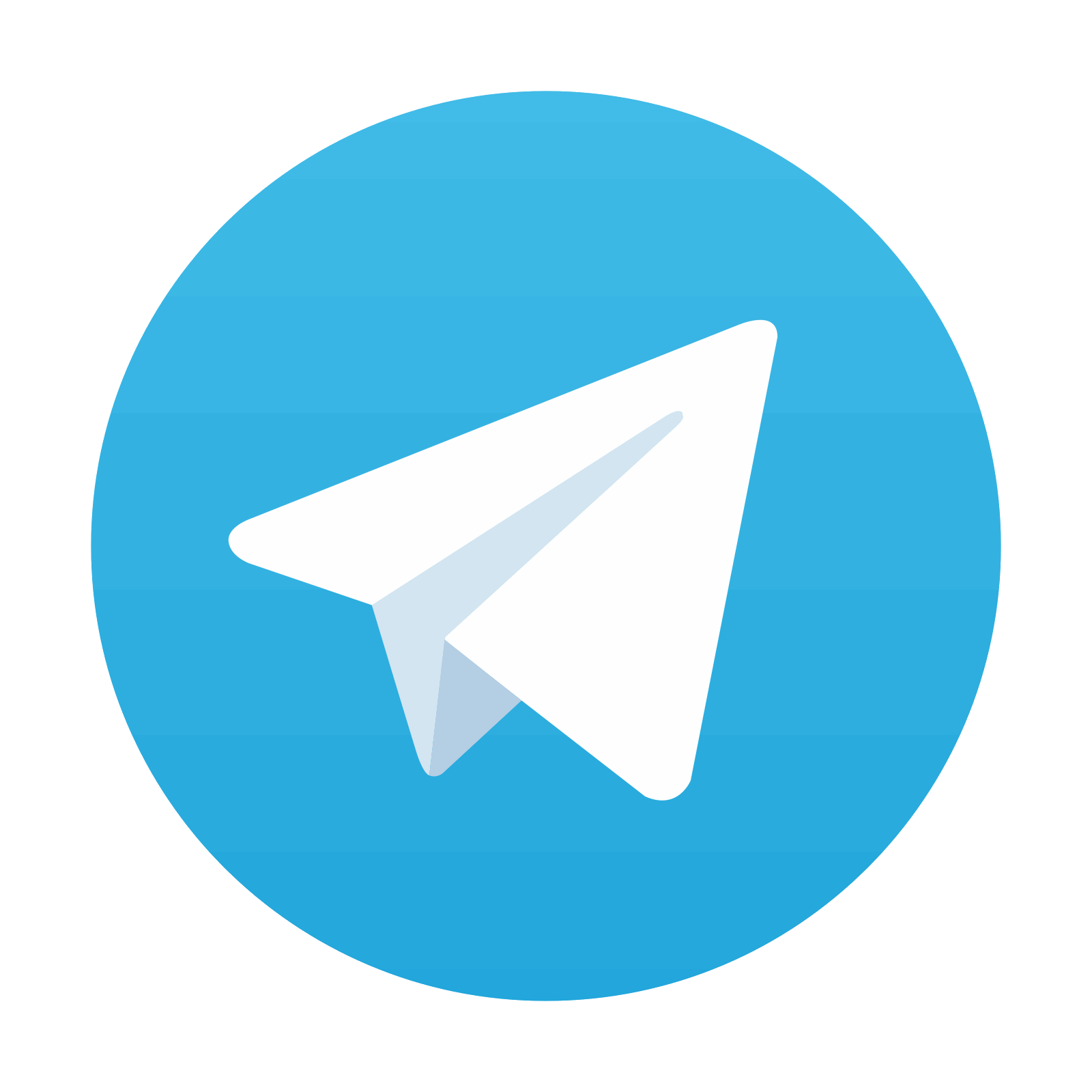
Stay updated, free articles. Join our Telegram channel

Full access? Get Clinical Tree
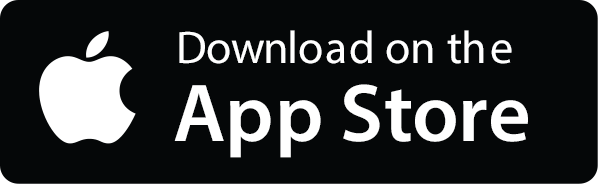
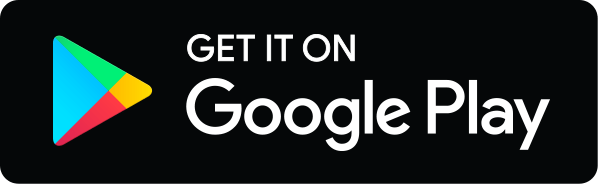
