Fig. 5.1
Axial views (upper panel) and volume rendering reconstructions (lower panel) of aortic computed tomography angiograms (CTA) with conventional single energy computed tomography (SECT) will full iodine volume load and dual energy computed tomography (DECT) imaging at different levels of iodine volume reduction. Panel (a) Conventional SECT CTA with 100 % iodine volume load. The mean signal density was 301.0 ± 38.9 HU. Panel (b) DECT CTA with 50 % iodine volume load, at 60 keV reconstruction. The mean signal density was 301.7 ± 28.3 HU. Panel (c) DECT CTA with 40 % iodine volume load, at 50 keV reconstruction. The mean signal density was 389.6 ± 35.4 HU. Panel (d) DECT CTA with 30 % iodine volume load, at 50 keV reconstruction. The mean signal density was 339.3 ± 43.8 HU
Image artifacts also can be reduced or avoided with the use of DECT applying the basic analysis that DECT uses monochromatic evaluation and material decomposition.
One of the major problems CTA faces in the calcium deposits in the walls of arteries that can generate two types of artifacts on the traditional CT images: the beam-hardening and blooming artifacts, originated by the polychromatic nature of x-rays and the energy dependency of x-ray attenuation.
Calcified plaques are overestimated in size and volume in single energy CT (SECT) hampering the assessability of luminal stenosis when they are severe. In these situations, inaccurate stenosis quantification can be achieved [24, 25]. These artifacts could be reduced with the generation of synthesized monochromatic image reconstruction, but especially with the ability of DECT to discriminate between two materials (calcium and iodine), that relies primarily on the difference between the dual energy ratio of each materials, determined by the separation between the high- and low-energy spectra and the difference between the effective atomic numbers of the evaluated materials (Fig. 5.2) [26–28].
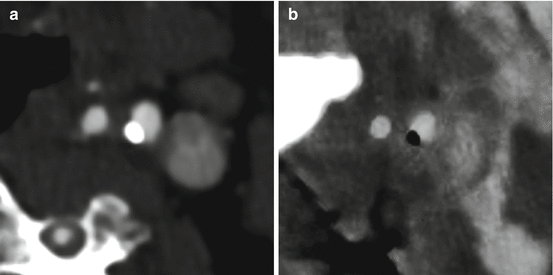
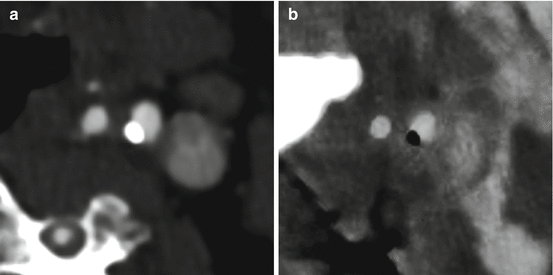
Fig. 5.2
Axial views of dual energy computed tomography (DECT) internal carotid angiograms. Panel (a) DECT 70-keV image (equivalent to a 120 kVp single energy CT image). Panel (b) DECT iodine (calcium) image. The material decomposition image allows a better and more reliable size assessment of calcified plaques
Calcified plaques can be better identified in real size using high energetic levels that reduce the overestimation of the plaque volume in general using 80 kev or higher. On the other hand using material decomposition, calcium can be removed from the vessel walls and allow to perform a more precise stenosis measurement. It is important to mention that this technique works better in larger vessels such as aorta and main branches (Fig. 5.3). However it has some limitations in smaller vessels such as lower extremities or coronaries.
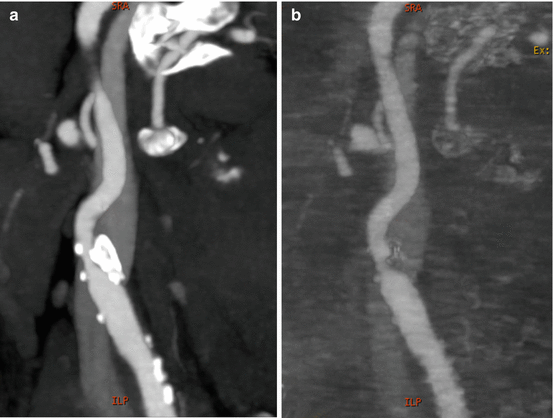
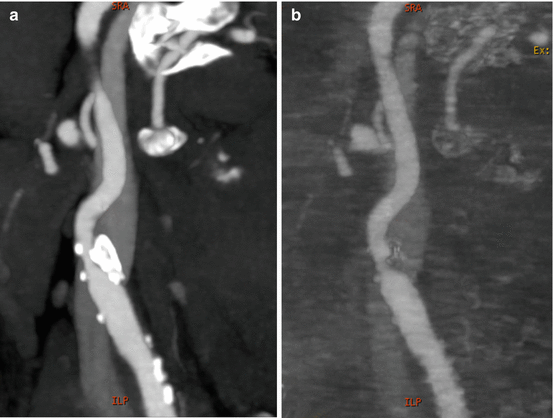
Fig. 5.3
Sagittal multiplanar views of dual energy computed tomography (DECT) internal carotid angiograms. Panel (a) DECT 70-keV image (equivalent to a 120 kVp single energy CT image). Panel (b) DECT iodine (hydroxyapatite) image. The material decomposition image allows calcium plaques removal and a more precise evaluation of the arterial lumen
DECT Technical Considerations
DECT has the ability to distinguish between materials, and based on that, it is appreciated as being potentially more accurate than single energy CT for determining several different contents such as calcium, bone, iron or fat [29–31].
Imaging at multiple energies provides redundant information on only two physical quantities, the density of the two basis materials. The X-ray attenuation on CT imaging has two physical mechanisms, the Compton scattering and the photoelectric absorption, that depend on the X-ray energy (single or dual) but also on the object. The two properties that establish the difference in attenuation between two different materials (such as calcium and soft tissue) are the effective atomic number (Zeff) and the electron density [32, 33].
On DECT systems, an object is imaged with X-rays of two different effective energies with a sufficiently large separation spectrum and in a very short time between both, to avoid errors resulting from motion or contrast uptake/washout become excessive. It will depend on the type of DECT scanner used. The more developed and most mature is the dual-source CT technology. These dual-source CT scanners use two X-ray tubes, mounted at 90° in the z-axis plane, each with their corresponding detector array [17, 34]. Nevertheless, the approach to dual kVp is the most competitive. The CT system rapidly switches between low and high kVp as the source-detector pair rotates about the object. The difference in time between successive high and low energy projections is very small, originating motion free and well-registered projection pairs, and a sufficient number of projections equally spaced in angle are acquired with each spectrum [35].
DECT Intravascular Contrast Considerations
One of the main advantages of dual energy CTA is the possibility to reduce the volume of contrast load. The major challenge of this contrast volume reduction is to maintain an adequate opacification for a given individual and vascular territory.
The use of iodine-based contrast media is related to the properties of iodine to absorb X-rays, and this ability is particularly increased on DECT when analyzing low-kiloelectron voltage mono-energetic images. Vascular enhancement depends on the amount of iodine molecules injected per unit of time. This contrast administration rate can be increased either by increasing the iodine concentration of the selected contrast media and/or the injection flow rate. A determined enhancement response is proportional to the iodine flux, or iodine administration rate. Nevertheless, the degree of vascular enhancement is highly variable between patients and physiologic and anatomic parameters like the cardiac output or the body weight must be taken in consideration. The optimal arterial enhancement in CTA exams is between 250 and 300 HU. It was suggested that levels arterial enhancement greater than 350 HU should be avoided because of difficulties in differentiating contrast from vascular calcifications [36, 37].
The intravenous contrast media administration is the main component of CTA. In daily clinical practice, a large cubital or antecubital vein is the most favorable injection site, and a 16–20-gauge peripheral catheter is selected for iodine contrast infusion on dedicated CTA studies. Especially using DECT, it is important to understand the influence of user-controllable injection parameters on arterial enhancement. Based on conventional single-energy CTA, it is established that the iodine flux is proportional to the injection flow rate and has a direct, proportional effect on arterial enhancement. For example, a 20 % increase in the contrast media concentration will determined a 20 % stronger enhancement; similarly, a 40 % increase in the injection rate (with the same duration of the contrast injection) will increase in 40 % the vascular enhancement, but using a 40 % more contrast media. Other important issue is that the injection duration has also impact on the arterial enhancement and there is minimum injection duration (around 10–12 s) to raise arterial enhancement to an appropriate level of opacification necessary to CTA scans [36].
As a maximum arterial opacification is of critical importance in these studies, the injection-to-scan delay needs to be timed relative to each patient contrast media transit time, which can be determined using either a test-bolus injection or a bolus-triggering technique. As mentioned earlier, one of the challenges of DECT vascular imaging is a reduction in the total contrast media volume. This volume reduction affects both the injection rate and the injection duration, and an adaptation of the injection parameters is necessary for obtain an adequate level of uniform arterial enhancement, with the minimum contrast-bolus related artifacts. Variable contrast media reductions between 50 % and 70 % of the theoretical patient-weight-based total contrast volume are proposed, maintaining on low-kiloelectron voltage images an appropriated arterial opacification (around 250 UH), without hampering of image quality or diagnostic consistency. A multiphasic injection protocol is an alternative solution, taking into account that the typical arterial enhancement pattern resulting from a prolonged injection is a continuous increase in the arterial enhancement. Table 5.1 shows a comparison of injection protocols between conventional and dual energy CTA.
Table 5.1
Comparison of contrast medium injection parameters between single- and dual-energy abdomen/pelvis CTA for an 80-Kg patient
Single-energy CTA | Non-coronary dual-energy CTA | Coronary dual-energy CTA | |
---|---|---|---|
CM concentration | ≥350 mg/mL | ≥350 mg/mL | ≥350 mg/mL |
Injection duration | Greater than 20 s | Greater than 20 s | Greater than 20 s |
Total CM volume/patient weight | 1 mL/Kg | 0.5 mL/Kg | 0.5 mL/Kg |
Total CM volume | 80 mL of CM | 40 mL of CM | 40 mL of CM |
Phase I volume | 80 mL of CM | 20 mL of CM | 25 mL CM + 25 mL saline |
Phase I injection rate | 4.5–5 mL/s | 3–3.5 mL/s | 3–3.5 mL/s |
Phase II volume | – | 20 mL CM + 20 mL saline | 15 mL CM + 35 mL saline |
Phase II injection rate | Same flow rate as phase I | Same flow rate as phase I | |
Saline flushing volumen | 40 mL | 40 mL | 40 mL |
Saline flushing injection rate | Same flow rate as phase I | Same flow rate as phase I | Same flow rate as phase I |
Image Processing Techniques
CTA image processing involves image-reformatting operations such as multiplanar reformats in coronal, sagittal, oblique and also curved planes, and more specific projectional methods, including the maximum intensity projection (MIP). Surface and volume rendering images utilize algorithms that create three-dimensional (3D) views by integrating a series of axial CT images. This 3D models represents a necessary tool for realistically analyze volume data, depicting complex vascular anatomy and also contributing to understand pathological changes [38, 39].
Several CT image acquisition parameters have a direct incidence on the quality of image processing. Thin isotropic sections and no reconstruction spacing reduce partial volume averaging effects on the z-axis, allowing better processing results. X-ray tube kilovoltage and current settings are also main factors that influence the image quality and also the iodine attenuation on CTA images. Low-peak kilovoltage (e.g. 80 kV) increase proportions of photons undergoing photoelectric absorptive interactions, with the consequent increase in iodine attenuation. However, the usage of low-peak kilovoltage imaging and traditional filtered back projection (FBP) reconstruction algorithms are associated with greater image noise that negatively influences the 3D image quality and the diagnostic image interpretation.
These potential impediments have been overcome during the last decade. The high levels of noise present in CT projection data of low-peak kilovoltage imaging result in an image with significant artifacts and high quantum mottle and electronic noise. These drawbacks of FBP reconstruction algorithm were resolve with the implementation of iterative reconstruction (IR) techniques. This model-based IR algorithm, focus on noise in projection space and attempt to create image reconstruction preserving the image texture and appearance with a better noise removal efficiency. And this properties are particular useful when DECT vascular imaging is utilized, remarking that one of the major benefits is the resultant iodine attenuation increase on low-energy DECT images [40].
Vascular DECT Workflow
DECT imaging permits a wide spectrum of image reconstructions that facilitate lesion visualization and characterization. Both the creation of low- and high-energy image datasets from the same imaging acquisition and the material-specific reconstructions with the ability to differentiate iodine and calcium has numerous potential clinical applications, particularly in vascular imaging [21, 42].
On the one hand, low-energy (e.g., 40 keV) DECT images, as occurred with low-peak kilovoltage images, enhance the conspicuity of iodinated contrast allowing an adequate degree of vascular enhancement despite a large reduction in iodine intravascular load. The other side of the monochromatic imaging spectrum, the high-energy (e.g., 140 keV) DECT images, contribute to metal artifact reduction, better assessment of stenosis quantification in severe calcified vessels and potentially may play a role in stent evaluation. On the other hand, material-specific applications in vascular imaging include the generation of virtual unenhanced (water-iodine) images, iodine-selective (iodine-water) images, bone- and calcium plaque-subtracted (calcium-iodine) angiographic images.
Monoenergetic Dataset Applications
Increased Vascular Attenuation
It is well established that low-peak kilovoltage scans have a large number of photons with low energies undergoing photoelectric absorptive interactions, especially around the K-edge of iodine (33 keV). This generates an increase in iodine vascular attenuation that can be translated into reduced contrast utilization. However, the limitation of low-peak kilovoltage CTA is the greater image noise that can reduce diagnostic information. New advances in CT technology with the development of iterative reconstruction algorithms and the introduction of DECT scanners in the clinical setting gave possible solutions to the above mentioned limitations. Until now iterative reconstruction can be used up to 60 keV improving image noise in that level.
DECT imaging clearly demonstrates the improved vascular attenuation properties of low energies images. Simulated monoenergetic datasets at low kiloelectron voltage (e.g., 40 keV) have a marked increase in enhanced vascular attenuation, with also higher noise (Fig. 5.4). It is important to consider the best peak-kilovoltage dose assignment between low- and high-peak kilovoltage spectra to achieve the best combination between higher vascular attenuation with acceptable image noise. Currently, iterative reconstruction algorithms are available to all the spectra of simulated monoenergetic datasets, including low-peak kilovoltage images (e.g., up to 60 keV), giving a definitive solution to the image noise problem [40–42]. In the near future iterative reconstruction could be applied to lower energy levels.
