Imaging Techniques and Indications
Conventional Radiography
Diagnostic imaging plays an important role in the evaluation of the upper airway and chest in children. Chest radiographs comprise about 40% of all pediatric imaging, in large part because congenital and acquired respiratory disorders are so common.
The most common indication for evaluation of the upper airway is stridor, or noisy breathing caused by airway obstruction. Stridor can be inspiratory, expiratory, or both, and characterization of stridor helps to formulate a practical differential diagnosis and determine the imaging indicated to diagnose the level of obstruction. When stridor is primarily expiratory, the pathology is almost always below the thoracic inlet, and chest radiography with frontal and lateral views is typically performed. When stridor is primarily inspiratory, anteroposterior (AP) and lateral views of the neck soft tissues are performed in addition to chest radiography to evaluate the upper airway. When children have stridor and are suspected to have airway obstruction, they are imaged in a position where they are most comfortable so that symptoms are not exacerbated.
In addition to inspiratory stridor, other indications for upper airway imaging include nasal obstruction, epistaxis, suspected foreign body, evaluation of nasopharyngeal lymphoid tissue, unexplained pulmonary hypertension, hoarseness or abnormal cry, trauma, caustic ingestion, and neck masses. The lateral radiograph of the neck is essential for identifying retained foreign bodies, evaluating for upper airway masses, and diagnosing epiglottitis. An AP view is added for the evaluation for croup or subglottic obstruction.
Indications for chest radiography in children include expiratory stridor, wheezing, tachypnea, chest pain, suspected infectious or inflammatory respiratory illness, trauma, known or suspected heart disease, and evaluation for metastatic or primary tumors. Chest radiography is generally not performed in children for routine hospital admissions or before sedation or anesthesia unless there are concerning symptoms and/or a history of recent respiratory illness.
Obtaining AP or posteroanterior (PA) and lateral views of the chest in children can be challenging, especially when they are younger than 4 years. A variety of immobilization techniques can be used. A pacifier may be helpful to obtain the chest radiograph during quiet inspiration. Supine AP films are usually easiest to obtain, and there is no appreciable difference in magnification between the supine AP and the upright AP or PA view in small children [ Fig. 2.1A ]. At age older than 4 years, PA and lateral views of the chest are performed with the patient standing or sitting upright.
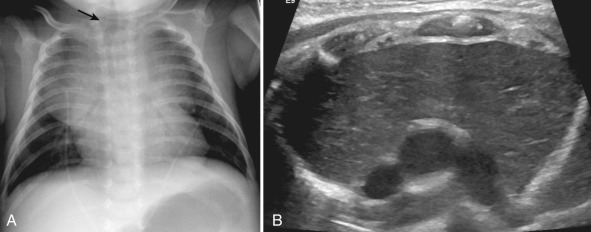
A supplemental view of the chest such as an expiratory image can be helpful to evaluate for and determine the size of a pneumothorax or detect dependent air trapping if there is concern for an aspirated foreign body. Decubitus views are useful to evaluate for layering of a pleural effusion when there is concern for possible loculation. Small amounts of pleural fluid (up to 2 mm thick) can be normal in children.
Ultrasound
Ultrasonography provides valuable diagnostic information to determine the cystic versus solid nature of neck masses and lesions in the superior mediastinum. Ultrasound can be used to distinguish between the normal, homogeneous-appearing thymus and a concerning mediastinal mass [ Fig. 2.1B ]. Cardiac structure and function can be exquisitely assessed by US without the use of ionizing radiation or contrast administration. Echocardiography is primarily performed by pediatric cardiologists. Ultrasound can be used to assess the size and complexity of pleural effusions. It is particularly useful in the setting of complete hemithorax opacification, allowing the detection of pleural fluid associated with collapse or consolidation of an entire lung. Ultrasound can provide an assessment of diaphragmatic excursion during inspiration and expiration in the evaluation for diaphragmatic paresis. Doppler US is useful in the evaluation of intravascular access and the assessment of vessel patency and/or thrombus formation. Ultrasound has the important benefit of portability, allowing for bedside evaluation of patients who are very ill and/or unable to travel.
Computed Tomography
Modern-day multidetector computed tomography (CT) scanning provides excellent image quality, particularly in the less than cooperative patient. There is a relatively low need for sedation or anesthesia, now used in less than 2% of patients younger than 4 years. The tracheobronchial tree, pulmonary congenital malformations, metastatic and interstitial lung disease, mediastinal pathology, and subpleural and chest wall lesions can now be assessed without breath-holding technique in small children using the rapid scanning techniques of currently available dual-source and volumetric CT scanners. High-resolution computed tomography (HRCT) provides excellent spatial resolution for the depiction of interstitial lung disease. Computed tomography angiography (CTA) has an established role in the evaluation of congenital cardiac anomalies (see Chapter 3 ) and the assessment of extracardiac thoracic vascular anomalies.
Magnetic Resonance Imaging
Magnetic resonance imaging (MRI) is a useful modality for the evaluation of mediastinal masses. It is particularly useful for demonstrating the extent of posterior mediastinal lesions. MRI and magnetic resonance angiography (MRA) are becoming more widely used in the evaluation of the mediastinal vasculature, congenital cardiac lesions, and anomalies of the great vessels. Both modalities are useful for the assessment and characterization of the vascular supply of congenital bronchopulmonary foregut malformations and vascular lesions of the lung. More recently, rapid MR sequences have allowed improved depiction of the pulmonary interstitium and ventilation and perfusion abnormalities, reducing the cumulative dose from surveillance CT scans. The morphology of congenital cardiac and vascular lesions and related MRI applications are discussed in detail in Chapter 3 .
Development of Airway and Lungs
Structural Development
In the fourth week of gestation, a ventral diverticulum arises from the developing foregut. This is called the laryngotracheal tube. The proximal portion of this develops into the larynx and trachea, and becomes continuous with the pharynx. The distal portion enlarges and divides into three right and two left bronchial buds, which will eventually form the right and left lungs. With continued growth and division, the terminal bronchioles and respiratory bronchioles develop. The process of lung development is complete by 28 weeks’ gestation. When the respiratory bronchioles become invested with capillaries, they are called terminal sacs or primitive alveoli. The primitive alveoli continue to mature until birth. During the last 2 months of prenatal life and up until the age of 8 years, the number of alveoli continues to increase. Growth of the lungs after birth is primarily due to an increase in the number of respiratory bronchioles and alveoli. Alveolar epithelial cells (types I and II) line the terminal sacs. Type II pneumocytes develop by 23 to 24 weeks’ gestation and are responsible for pulmonary surfactant production. Respiration is possible at 24 weeks’ gestation, when sufficient terminal sacs exist to allow survival of the premature infant with respiratory support in the intensive care unit.
Development of the pulmonary vasculature begins concurrently in the fourth week of gestation. A primitive pulmonary artery arises from the ventral aspect of the right and left sixth aortic arches, extending caudally toward the developing tubular lung bud. This is eventually incorporated into the mesenchymal tissue around the primitive trachea and bronchial buds. A pulmonary artery accompanies each developing bronchial bud. The venous return develops from the mesenchyme and the cardiac wall, and rather than following the bronchial tree, runs between the bronchopulmonary segments and drains to the left atrium.
Tracheal cartilage first begins to differentiate during the fourth week of gestation. Distinct rings of cartilage are present along the trachea and mainstem bronchi by 11 weeks’ gestation. The development of cartilage lags behind the branching of the airways; as such, cartilage does not extend into the more peripheral pulmonary bronchi. Any disturbance of this orderly sequence of events may result in a predictable set of developmental aberrations. These are referred to as bronchopulmonary foregut malformations, and they include congenital lobar emphysema, bronchial atresia, and sequestration. These entities are discussed in depth later in this chapter. The submucosal mucous-secreting glands of the bronchi and bronchioles arise from the epithelial cells and migrate into the submucosa. These glands are even slower to develop than the cartilage.
In children, insults (e.g., viruses) to the lungs primarily affect the terminal and respiratory bronchioles. In contrast, in adults such insults primarily affect the interstitium or the airspaces.
Functional Development
Successful lung maturation and function require the completion of both structural development and biochemical development of the surfactant system. Incomplete structural development and/or premature birth before development of the surfactant system will lead to respiratory compromise or insufficiency in the newborn.
Surfactant is a mixture of phospholipids and hydrophobic proteins produced by type II pneumocytes and secreted into the alveolar spaces. The production of surfactant is independent of lung growth. Surfactant decreases the surface tension within alveoli and prevents alveolar collapse during expiration. Surfactant production gradually increases with advancing gestational age, with full maturation by 36 weeks in most fetuses. Therefore birth at any time before 36 weeks’ gestation may be associated with respiratory compromise or failure as a result of surfactant deficiency.
In infants, the balance among central airway compliance, peripheral airway resistance, and lung recoil differs compared with that of older children and adults, resulting in different patterns of airflow. Any adverse influences during this period may diminish airway and/or alveolar growth, affecting lung and airway size ultimately attained. Such potentially harmful factors include allergens, viruses, and air pollutants.
Physiologically, the respiratory cycles of a healthy infant are markedly different from those of an infant suffering from peripheral airway disease. Diffuse peripheral airway inflammation is referred to as bronchiolitis. In children with bronchiolitis, the tidal volume of the lungs is small and the residual volume is significantly higher than normal. This leads to air trapping. In severe cases, the degree of air trapping may approach total lung capacity.
On radiographs, hyperinflation is seen in association with thickened (visible) bronchial walls and areas of atelectasis. Peribronchial thickening or “cuffing” is best seen on the lateral radiograph. Other radiographic findings include flattening of the hemidiaphragms, anterior bowing of the sternum, and a more “horizontal” orientation of the ribs. These radiographic findings reflect the physiologic changes and may vary over time. Atelectasis is relatively frequent in children compared with adults because collateral air circulation through the pores of Kohn and canals of Lambert is less efficient [ Fig. 2.2 ].
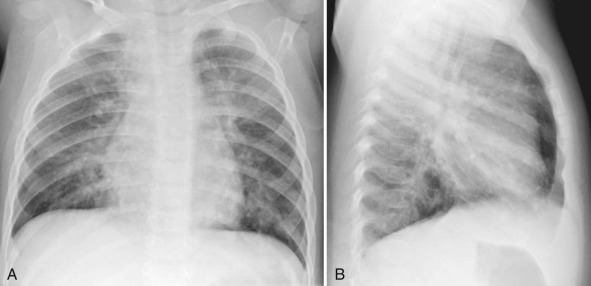
Normal Anatomy and Variants
Pharynx
The pharynx is divided into the nasopharynx, oropharynx, and hypopharynx. An important anatomic structure is the Waldeyer ring, composed of the adenoids superiorly, the palatine tonsils laterally, and the lingual tonsils inferiorly. On a lateral radiograph of the neck soft tissues, the retropharyngeal soft tissues extend from the adenoids, which are visible by 3 to 6 months of age, to the origin of the esophagus at the level of C4-C5. Prominent adenoids become pathologic when they encroach on the nasopharyngeal airway [ Fig. 2.3 ]. The palatine tonsils are outlined by air only with marked distention of the hypopharynx. The lingual tonsils are occasionally visible radiographically at the base of the tongue. Measurements of the adenoid tissues and tonsils are notably neither reliable nor useful.
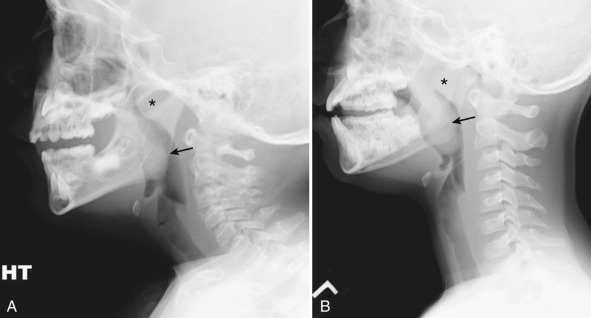
A quantitative assessment of retropharyngeal soft tissue thickness is important. A useful ratio is that of the retropharyngeal soft tissue thickness to the C2 vertebral body width. On a lateral view of the neck obtained in inspiration, this ratio changes from approximately 1.0 until almost 1 year of age to 0.5 by 6 years of age. On average, the retropharyngeal soft tissue width above the level of C4 should not exceed 50% of the vertebral body width [ Fig. 2.4 ]. Notably, the retropharyngeal soft tissues may become quite thick on radiographs obtained in expiration or without full neck extension. This can lead to a false-positive diagnosis of retropharyngeal abscess. If an examination is equivocal, a repeat view with full inspiration and neck extension should be obtained.
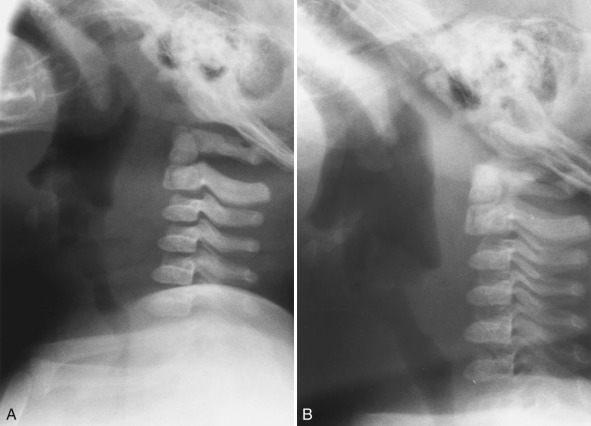
Larynx
The larynx extends from the base of the tongue to the trachea. It is composed of three major cartilaginous structures—the epiglottis, the thyroid cartilage, and the cricoid cartilage—and three small paired cartilaginous structures—the arytenoid, cuneiform, and corniculate cartilages. A practical anatomic division of the larynx consists of three regions: (1) a supraglottic region containing the epiglottis, aryepiglottic folds, and false vocal cords; (2) a glottic region containing the laryngeal ventricle and the true vocal cords; and (3) a subglottic region extending from the inferior aspect of the true vocal cords to the lower cricoid cartilage. Other anatomic landmarks of the larynx include the hyoid bone, body, and horns, which may be ossified at birth. The horns of the hyoid are oriented in such a way that they “point” to the epiglottis on a conventional lateral radiograph of the neck [ Fig. 2.5 ]. Calcification of respiratory cartilage is notably rare in children and is pathologic. It is seen in such conditions as chondrodysplasia punctata and relapsing polychondritis.
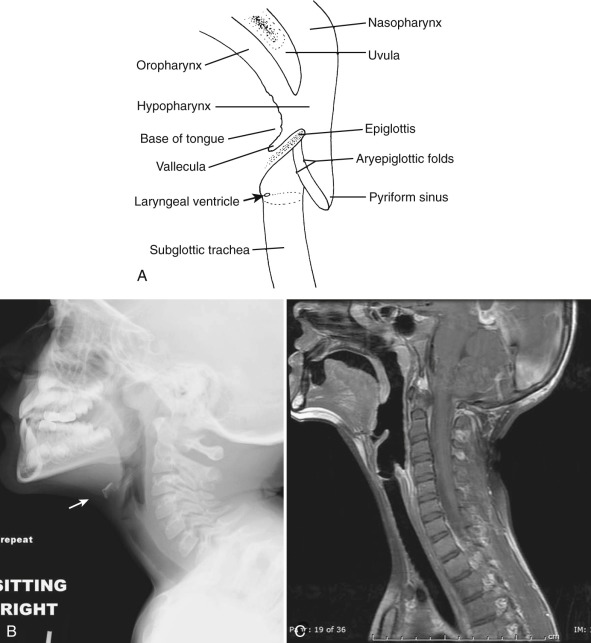
Mediastinum
The variable size and shape of the normal mediastinum in children can result in challenges in the interpretation of chest radiographs. Compartmentalization of the mediastinum represents an arbitrary classification to facilitate description of the location of disease and determine pathology. Classically the mediastinum has been divided into a superior portion and an inferior portion by a line drawn from the manubrial sternal junction to the T4-T5 intervertebral disk space. The inferior portion is typically further divided into anterior, middle, and posterior portions by classification systems that vary to some degree. This system is somewhat more difficult to apply to the mediastinum of infants and children.
Using a simplified classification system, the trachea, esophagus, heart, vasculature (including the aorta, superior vena cava (SVC), inferior vena cava (IVC), and central pulmonary arteries and veins), vagus and phrenic nerves, and lymph nodes comprise the middle mediastinum (M). The thymus occupies much of the anterior mediastinum (A), also referred to as the prevascular space. The posterior mediastinum (P) consists of the structures posterior to a line drawn along the anterior edge of the vertebral bodies, and includes the paraspinal region [ Fig. 2.6 ]. This compartmentalization is easily applied to cross-sectional imaging modalities such as CT and MRI [ Fig. 2.7 ]. When an anterior or middle mediastinal lesion is suspected on conventional chest radiographs, both CT and MRI are appropriate modalities for further evaluation. When a posterior mediastinal lesion is suspected, MRI is preferred to assess for possible intraspinal extension.
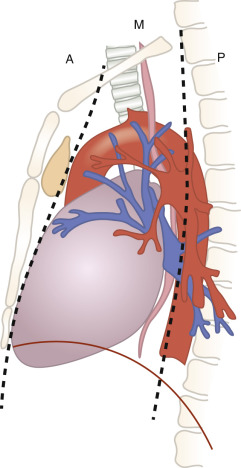
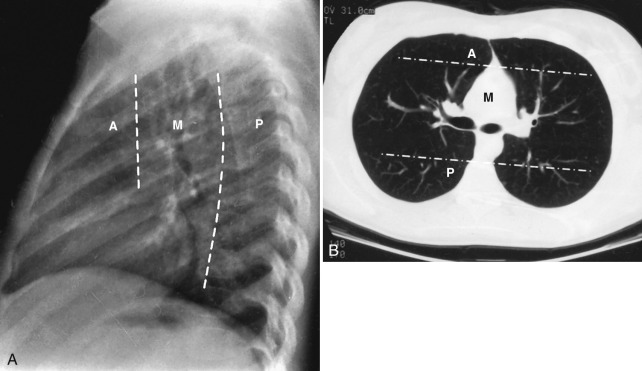
The mediastinum is usually prominent on chest radiographs in children due to the relatively large size of the thymus. The thymus is often visible up to 3 years of age, and may be seen until the age of 8 or 9 years. Maximal thymic size is reached during the first few months of life. The thymus then becomes relatively smaller with further growth of the rest of the body. It has a quadrilateral shape in infancy and gradually becomes triangular-shaped in later childhood and adolescence as it begins to involute. The normal thymus has a soft consistency. It does not compress adjacent structures; instead, it adapts its contour to surround structures of the middle mediastinum. The thymus does not displace the trachea or mediastinal vascular structures.
On chest radiography, the thymus has a similar density to the heart and vascular structures. Thus clear delineation of the borders of these structures may not be possible in the setting of a prominent thymus. Because of its anterior location, the margins of the thymus may be indented by the anterior ribs (“thymic wave” sign) [ Fig. 2.8 ]. The right lobe of the thymus can insinuate into the minor fissure, creating a sail-like contour (“thymic sail” sign) [ Fig. 2.8 ]. The thymus can change shape during respiration. It can elongate and narrow on inspiration, with apparent increase in size on expiration. Occasionally a notch in the mediastinal contour can be seen at the junction of the thymus and the heart. This is called the cardiothymic incisure.
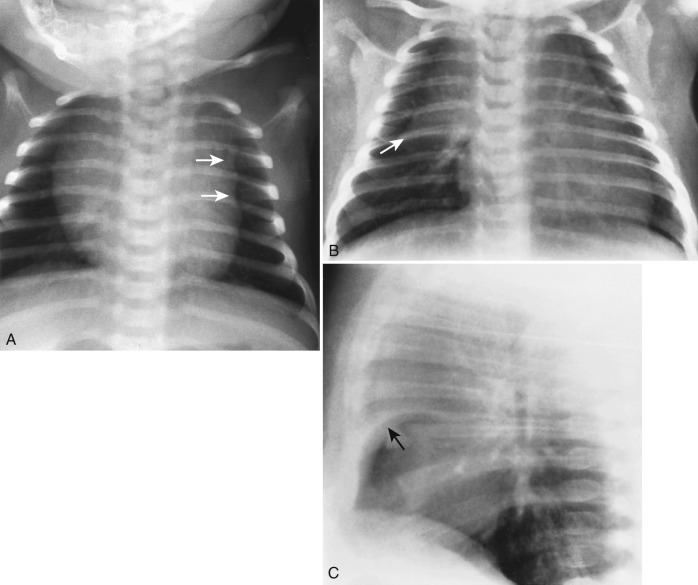
The thymus can dramatically decrease in size when a child is under stress. This may occur in the setting of an acute respiratory illness, surgery, steroid treatment, and radiation and/or chemotherapy. The thymus will regenerate when the stress resolves or when therapy is discontinued. Regeneration of the thymus or “thymic rebound” may result in a larger organ than existed before the insult in up to 25% of children. However, in the majority of cases the thymus grows up to 50% of its original size.
The variable appearance of the mediastinum on chest radiographs in children can often be a result of the normal variation in thymic contour. When questions arise, ultrasound is the modality of choice to evaluate for the presence of a normal thymus versus a mediastinal mass [ Fig. 2.9 ]. On CT and MRI, normal residual thymic tissue may be visualized until the early teens. It may be seen insinuating between the vessels of the mediastinum. On CT, the thymus appears homogeneous throughout childhood, with attenuation similar to or slightly greater than that of the adjacent chest wall musculature on unenhanced studies [ Fig. 2.10A ]. On MRI, the thymus is slightly hyperintense to skeletal muscle on T1-weighted images and slightly hypointense to fat on T2-weighted images [ Fig. 2.10B and C ].
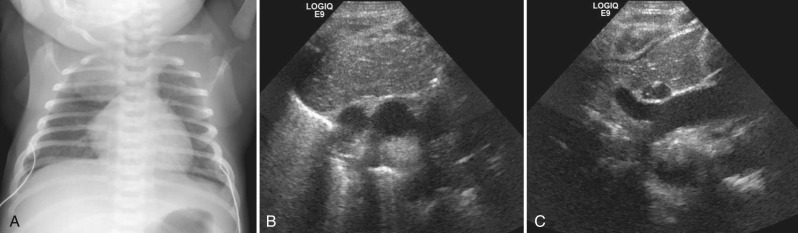
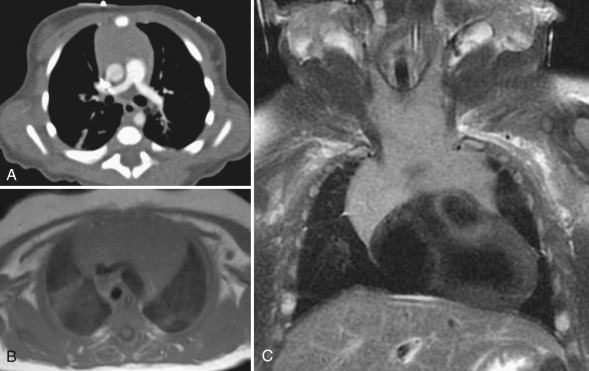
On chest radiographs, the contour and size of the heart should be evaluated in the context of positioning of the child when the radiograph was obtained. The heart typically appears more prominent when the child is imaged supine as opposed to upright. Regardless of position, the chest radiograph should be obtained with adequate pulmonary inflation. On an expiratory chest radiograph, a misdiagnosis of cardiomediastinal enlargement may be made.
The tracheal air column should be carefully evaluated on both frontal and lateral chest radiographs. On the frontal view, the normal subglottic trachea has a bilaterally symmetric convex appearance, typically described as “shouldering” [ Fig. 2.11 ]. As the trachea courses inferiorly into the chest, the diameter should remain uniform to the level of the carina except for a mild, smooth indentation at the level of the aortic arch. The trachea is normally positioned to the right of the midline as it courses by a left aortic arch. When the trachea is positioned in the midline or leftward within the mediastinum, mass effect caused by a right aortic arch or mediastinal mass should be considered. Careful inspection for associated tracheal narrowing is important to detect, particularly in the setting of stridor. The trachea is relatively more flexible in children younger than 5 years. On expiratory radiographs, the normal trachea may buckle or angle to the side opposite the aortic arch, typically to the right [ Fig. 2.1A ]. This finding is most pronounced in infants. A normal trachea can decrease in diameter up to 50% during expiration, especially in the neonatal period. Collapse of the trachea greater than 50% raises the possibility of tracheomalacia.
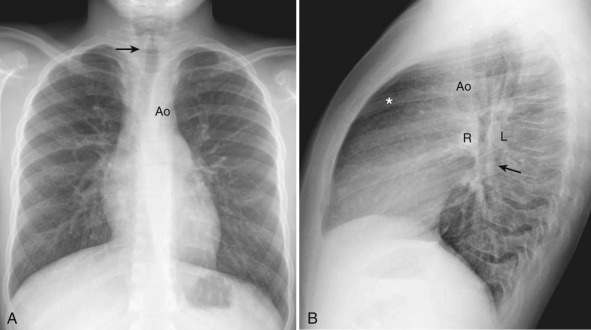
Lungs
Chest radiography is most often performed in children for evaluation of the lung parenchyma. Evaluation should begin with an assessment of technique, including patient rotation and the degree of inspiration.
Support Lines and Tubes in Intensive Care Patients
The evaluation of chest radiographs of neonates in the intensive care unit usually begins with an assessment of support lines and tubes. Incorrect positioning of umbilical venous catheters (UVCs), umbilical arterial catheters (UACs), endotracheal tubes (ETTs), and enteric tubes is important to recognize. Malpositioning of these devices can lead to a prolonged hospital stay, with significant associated morbidity and mortality.
Umbilical venous and arterial catheters enter through the umbilicus, which can be seen as a well-defined, rounded density outlined by air on an abdominal radiograph. Correct positioning of a UVC follows along the expected course of the umbilical vein from the umbilicus into the liver, usually to the right of midline. Blood from the placenta enters the umbilical vein. The umbilical vein then joins the left portal vein, continues to the ductus venosus, then subsequently to the middle or left hepatic vein, the IVC, and the right atrium. Optimal positioning of the UVC is within the suprahepatic IVC just below the right atrium, usually at the level of the right hemidiaphragm. A UVC should take a fairly straight course superiorly to reach the suprahepatic IVC. It should never loop as it courses into the liver [ Fig. 2.12A ]. A UVC may have an abnormal course through the liver parenchyma, terminating in the right or left portal vein. The UVC may also extend into the main portal vein, and subsequently to the superior mesenteric or splenic veins [ Fig. 2.12B ]. Abnormal positioning of the UVC within a portal vein may result in portal vein thrombosis, liver damage, and even portal vein perforation. If the UVC is advanced too far, it may terminate in the right atrium or extend farther superiorly to enter the SVC. It may cross a patent foramen ovale to terminate in the left atrium. Malpositioning of the UVC within the heart can be associated with arrhythmia or even perforation.
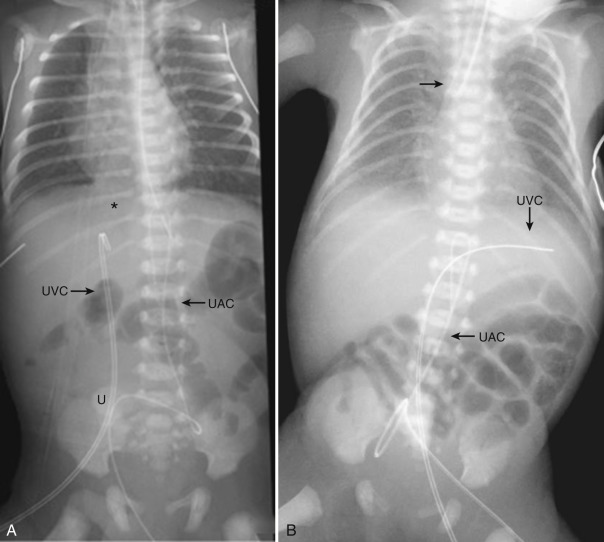
A correctly placed UAC follows the course of one of the paired umbilical arteries through the umbilicus. The umbilical arteries return blood to the placenta from the internal iliac arteries. The UAC takes an inferior course from the umbilicus to enter into one of the umbilical arteries, then turns superiorly to course into the common iliac artery and subsequently into the aorta. The UAC ideally terminates within the aorta superior to the origin of the celiac artery and well below the branches from the thoracic aortic arch. This is generally between the T6 and T10 vertebral bodies. This is commonly referred to as a “high” UAC. A “low” UAC is positioned with the tip below the renal arteries or the level of the L3 vertebral body. This positioning is acceptable but may be associated with more complications. A malpositioned UAC may loop in the region of the aorta [ Fig. 2.12 ] or take an aberrant course into a branch of the abdominal aorta.
Percutaneously inserted central catheters (PICC) are now being used for long-term access in neonates to avoid the higher complication rates associated with umbilical catheters. The tip of a PICC inserted from an upper extremity peripheral vein should terminate in the SVC, whereas a PICC inserted from a lower extremity approach should terminate in the IVC inferior to the right atrium.
An ETT should be positioned in the intrathoracic trachea above the level of the carina. High positioning of the ETT within the cervical trachea carries a risk for accidental extubation. Low positioning within a mainstem bronchus may cause hyperinflation of the ipsilateral lung and collapse of the contralateral lung.
Extracorporeal membrane oxygenation (ECMO) provides support to neonates with severe respiratory distress related to conditions such as congenital diaphragmatic hernia (CDH), meconium aspiration, and primary pulmonary hypertension. Venous blood is diverted to an extracorporeal membrane for oxygenation, then returned to the patient via the arterial circulation for combined pulmonary and cardiac support. This is referred to as venoarterial (VA) ECMO. Blood can be returned to the venous circulation alone when only pulmonary support is needed. This is referred to as venovenous (VV) ECMO.
VA ECMO involves separate venous and arterial cannulas, whereas VV ECMO can be performed with a single double-lumen venous cannula [ Fig. 2.13 ]. The arterial cannula is often placed via the right common carotid artery, with the tip terminating in the brachiocephalic artery. It can also be placed in the femoral artery. The venous cannula is most often placed via the internal jugular vein, with the tip terminating in the right atrium. Bedside echocardiography is now routinely used for the initial evaluation of cannula placement. Subsequently, follow-up chest radiographs are used to document stability of positioning.
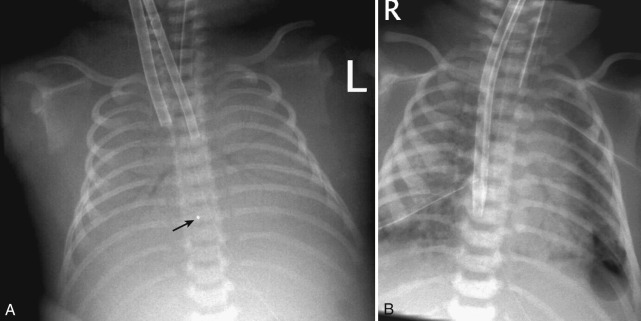
Upper Airway
Supraglottic Abnormalities
Laryngomalacia
Congenital laryngomalacia is the most common cause of stridor in the newborn. It is characterized by an infolding of the aryepiglottic folds during inspiration, leading to collapse and obstruction of the airway. Infants typically present with inspiratory stridor that worsens with feeding, excitement, agitation, crying, and supine positioning.
The diagnosis is usually made by laryngoscopy, although airway fluoroscopy may first be performed to establish the diagnosis. On imaging, downward and posterior displacement of the epiglottis and anterior buckling of the aryepiglottic folds can be seen.
Symptoms are usually self-limiting, with resolution by 12 to 18 months of age. At this point, the arytenoid tissues strengthen and become more firmly attached to the underlying cartilage. Before resolution, serious complications such as airway obstruction and sudden death can occur.
Acute Epiglottitis
Acute epiglottitis is a life-threatening condition caused by infection and inflammation of the epiglottis and aryepiglottic folds, potentially leading to acute airway obstruction. The peak incidence of epiglottitis is between 3 and 6 years of age; however, this entity can also occur in adults. Historically, the most common causative agent was Haemophilus influenzae type B (HIB). Since the widespread administration of the HIB vaccine, infection with group A β-hemolytic streptococcus has become more frequent. Routine administration of the HIB vaccine in infancy has led to a dramatic decrease in the incidence of epiglottitis in young children. It is now more commonly diagnosed in the older pediatric population.
The clinical presentation can be striking. The affected child assumes a bold upright position, with the head held forward in respiratory distress. In severely affected patients, immediate treatment of acute epiglottitis involves intubation with direct laryngoscopy to secure the airway.
If imaging is obtained, a lateral radiograph of the neck soft tissues should be performed. The patient should be kept in an upright position, and there should be minimal to no manipulation of the neck. The classic radiographic findings of epiglottitis are marked enlargement and edema of the epiglottis and aryepiglottic folds, well-profiled on a lateral view of the neck with the characteristic “thumbprint sign” [ Fig. 2.14 ]. The hypopharynx may be overdistended . Subglottic edema, as seen in croup, will also be present in about 25% of patients with epiglottitis.
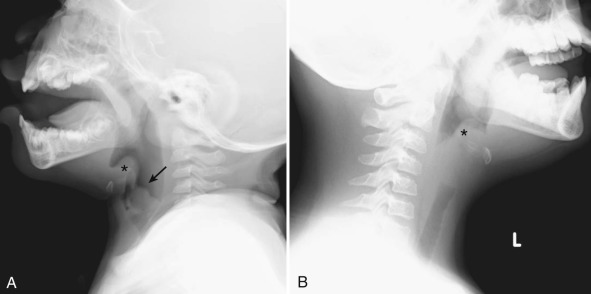
In addition to airway management, patients with epiglottitis require antimicrobial therapy. The role of steroids is controversial. They may reduce airway inflammation, resulting in improved airway patency.
Glottic Abnormalities
Laryngeal Atresia
Laryngeal atresia is a rare anomaly. It may be characterized by agenesis of the glottis, agenesis of the larynx, or both. The pathogenesis involves maldevelopment of the sixth branchial arch, resulting in failure of the larynx and trachea to recanalize. This entity may occur in isolation or as part of a number of syndromes. It can be associated with tracheoesophageal fistula (TEF), esophageal atresia (EA), urinary tract abnormalities, and limb anomalies.
Prenatal ultrasound may show signs of congenital high airway obstruction syndrome. These include dilated airways distal to the obstruction, bilaterally enlarged and echogenic lungs, and diaphragmatic flattening and/or inversion. Associated fetal ascites, hydrops fetalis, and polyhydramnios may be seen . Fetal MRI can assist in the prenatal diagnosis of this entity and provide a more accurate evaluation of the level of obstruction [ Fig. 2.15 ].
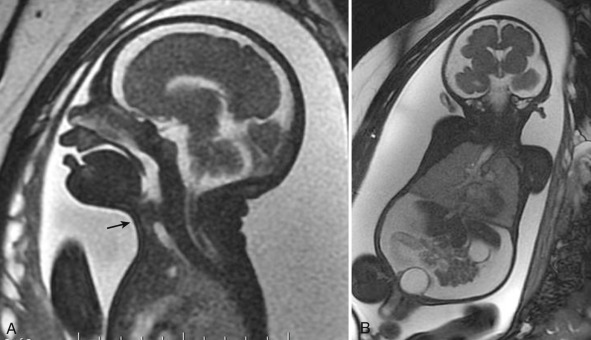
Patients typically present with asphyxia at the time of birth, which may require an emergent tracheotomy soon after delivery. If laryngeal atresia is detected in utero, an ex utero intrapartum treatment (EXIT) procedure with tracheotomy can be performed, which may be lifesaving.
Subglottic Abnormalities
Congenital Subglottic Stenosis
Subglottic stenosis is the third most common cause of congenital stridor in the neonate behind laryngomalacia and vocal cord paralysis. It involves narrowing of the subglottic lumen caused by incomplete recanalization during embryogenesis. There are two types of congenital subglottic stenosis: membranous and cartilaginous. Patients with congenital subglottic stenosis may be asymptomatic until an upper respiratory infection causes further narrowing and compromise of the airway. The affected patient may present with stridor and a barking cough. With severe disease, patients present with dyspnea and marked chest retractions.
Although subglottic narrowing may be appreciated on AP and lateral radiographs, congenital subglottic stenosis is a clinical endoscopic diagnosis. CT and MRI can aid in the assessment of the precise location and length of the stenosis. These modalities are also useful for evaluation of the airway distal to the level of narrowing [ Fig. 2.16 ].
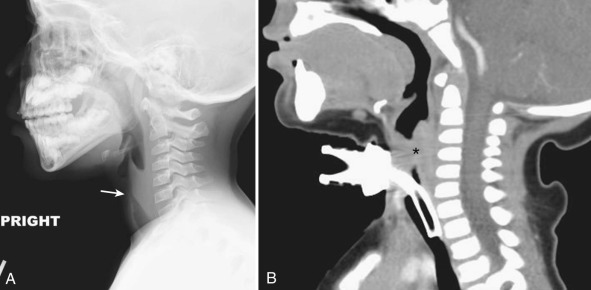
The management of congenital subglottic stenosis involves supportive care in times of airway compromise. Ultimately surgical reconstruction is needed to provide the patient with an adequate airway for normal activity without the need for tracheostomy.
Croup
Croup, also known as acute laryngotracheobronchitis, is a self-limited viral inflammatory disease of the upper airway. It is caused by parainfluenza or respiratory syncytial virus, and results in symmetric subglottic edema. It is the most common cause of upper respiratory distress in infants and young children, with a peak incidence between 3 and 6 months of age. Acute clinical symptoms include a barky cough, inspiratory stridor, and hoarseness. Symptoms may be preceded by a prodrome of low-grade fever, mild cough, and rhinorrhea.
Imaging is not routinely indicated because the diagnosis is often made clinically. Radiographs are sometimes obtained to exclude more serious causes of stridor such as epiglottitis, a retained foreign body, or a neck mass. On frontal radiographs, subglottic edema is visualized as loss of the normal shouldering (lateral convexities) of the subglottic trachea, creating a “steeple sign” [ Fig. 2.17 ]. Lateral radiographs of the upper airway demonstrate a normal epiglottis with narrowing of the subglottic region. Overdistention of the hypopharynx can be seen on both views.
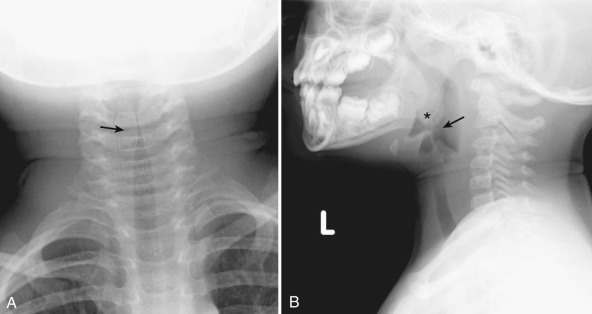
Croup usually has a self-limited course and resolves within a few days. Therefore supportive care is often sufficient. Oral or inhaled corticosteroids may be administered to decrease the severity of symptoms and potentially avoid hospital admission. Epinephrine nebulizer treatments may be helpful in some cases. Intubation is necessary when disease is severe. In children with atypical, prolonged, or recurrent symptoms refractory to medical therapy, bronchoscopy may be helpful for further evaluation.
Bacterial Tracheitis
Bacterial tracheitis is a bacterial infection of the trachea, with common causative agents including Staphylococcus aureus, Streptococcus pneumoniae, and Haemophilus influenzae. This infection is associated with a purulent exudate that can cause acute, life-threatening obstruction of the upper airway in rare circumstances. Preschool and early school-aged children are most frequently affected. The clinical features of bacterial tracheitis are similar to those of viral croup and epiglottitis. Children initially present with sore throat, rhinorrhea, cough, and fever, which may escalate to severe upper airway obstruction, high fever, and toxicity.
On a lateral view of the neck soft tissues, linear soft tissue filling defects may be seen along the airway. Irregular plaques along the anterior wall of the trachea may also be seen. This is known as the “candle-dripping sign” [ Fig. 2.18 ]. On an AP radiograph of the neck, narrowing of the subglottic airway may be visualized. Notably, concomitant pneumonia can be seen on chest radiographs in approximately half of patients. The most definitive way to diagnose bacterial tracheitis is via direct visualization with bronchoscopy.
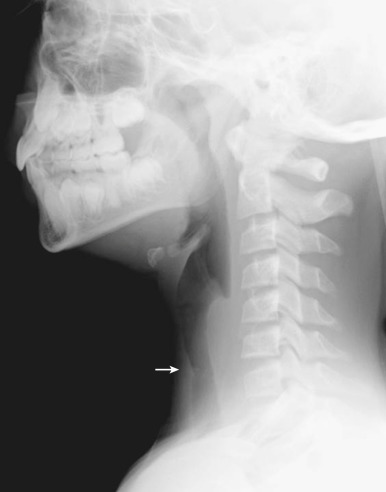
Because bacterial tracheitis can result in airway obstruction leading to respiratory failure, treatment is aggressive. Broad-spectrum intravenous antibiotics are initiated as soon as the clinical diagnosis of bacterial tracheitis has been made. If membranes are visualized within the trachea, rigid bronchoscopy can be performed for “stripping” of the airway.
Retropharyngeal Cellulitis/Abscess
Retropharyngeal cellulitis/abscess is a potentially life-threatening infection involving the retropharyngeal space. Retropharyngeal infection can be secondary to pharyngeal trauma from a penetrating foreign body, endoscopy, an intubation attempt, or a dental procedure. It may also occur in association with infectious pharyngitis, vertebral body osteomyelitis, and petrositis. Retropharyngeal infections can range from cellulitis to a mature abscess. The most common causative organisms are S. aureus, H. influenzae, and Streptococcus. This infection occurs most commonly between the ages of 2 and 4 years.
Children with retropharyngeal abscess generally appear very ill, presenting with sore throat, dysphagia, poor oral intake, dehydration, fever, chills, and an elevated white blood cell count and erythrocyte sedimentation rate. The affected patient may appear toxic, with marked neck pain and limited range of motion. Airway compromise may be seen at initial presentation.
Imaging evaluation includes lateral radiographs of the neck soft tissues and/or CT scan of the neck with intravenous contrast administration. On lateral radiographs, there may be widening of the retropharyngeal soft tissues with anterior displacement of the airway [ Fig. 2.19A ]. As indicated previously, the normal thickness of the retropharyngeal soft tissues from C1-C4 should be about equal to half of the vertebral body width in children. Rarely, gas may be seen in the retropharyngeal soft tissues in the setting of an abscess.
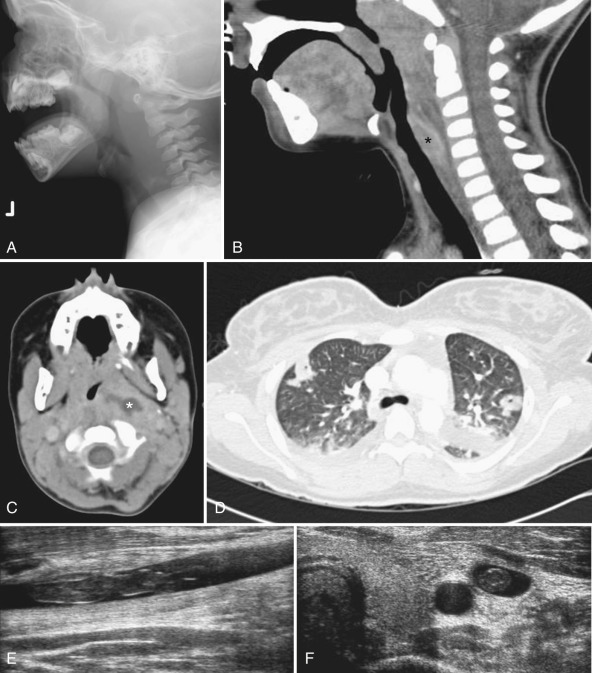
Contrast-enhanced CT scan is ideal to assess the craniocaudal extent of disease. The retropharyngeal space may be distended by a rim-enhancing fluid collection [ Fig. 2.19B and C ]. Sepsis may develop, with septic emboli to the lungs [ Fig. 2.19D ]. Vascular complications including jugular vein thrombosis or thrombophlebitis can be seen in the setting of Lemierre syndrome [ Fig. 2.19E and F ]. Narrowing of the internal carotid artery may develop, or rarely, an internal carotid artery pseudoaneurysm and/or rupture may occur.
Traditionally, management of a retropharyngeal abscess has involved surgical drainage. This is evolving, and some cases are now managed with antibiotics alone. This is particularly true for small collections.
Other Causes of Upper Airway Disease
Tonsillar Enlargement/Adenoidal Hypertrophy
Enlargement of the tonsils and adenoids in children may be related to normal development of the immune system. It is usually asymptomatic. However, when there is excessive adenotonsillar enlargement associated with sore throat and/or recurrent ear or sinus infections, the affected child may experience difficulty breathing or swallowing. Tonsillar inflammation is typically bilateral and can affect children and young adults. Obstructive sleep apnea may also result from adenotonsillar hypertrophy.
A lateral radiograph of the neck soft tissues can be obtained to evaluate size of the tonsils and adenoids [ Fig. 2.3 ]. In the case of tonsillar inflammation with persistent symptoms, a contrast-enhanced CT scan is useful to distinguish between acute tonsillitis and tonsillar/peritonsillar abscess. This distinction is important because these symptoms may progress to lockjaw (trismus) refractory to antibiotic treatment without appropriate management. In the setting of tonsillitis, CT shows bilateral tonsillar enlargement with variable attenuation and a striated pattern of parenchymal enhancement [ Fig. 2.20 ].
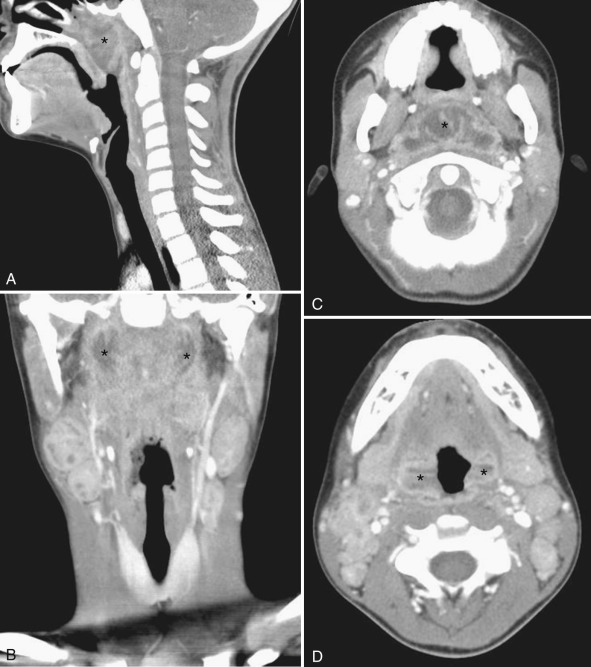
MR sleep studies are useful to detect both anatomic and dynamic motion abnormalities in children with obstructive sleep apnea related to tonsillar enlargement. These studies include a combination of static T1-weighted imaging and both static and dynamic cine T2-weighted imaging.
Excision of the tonsils and adenoids is indicated in the setting of obstruction and in cases of recurrent acute or chronic tonsillitis.
Foreign Body
Foreign bodies lodged in the larynx can lead to significant morbidity and mortality in children. The majority of patients who present are younger than 4 years, and peanuts are the most common offending agent. Children who ingest or aspirate foreign bodies may have a delayed presentation of acute respiratory distress, days or even months after the aspiration event. This is particularly true if the foreign body aspiration goes unwitnessed. Typically there is a suggestive history marked by an acute episode of paroxysmal cough and stridor. Other common symptoms include cyanosis, choking, and dyspnea.
Radiologic evaluation should begin with frontal and lateral views of the neck and chest, which can be helpful in localizing radiopaque foreign bodies such as coins, batteries, lead, mineral fragments, and some pills [ Fig. 2.21 ]. Coin ingestion is very common in children. Most coins cause no harm and pass through the gastrointestinal (GI) tract in a few days. However, if a coin becomes impacted at the thoracic inlet or enters the airway, interventional therapy is warranted. Disk (button) batteries are also commonly ingested by children [ Fig. 2.21D ]. These batteries contain a variety of caustic and corrosive agents. Perforation and systemic toxicity related to heavy metal poisoning may occur if their containers are compromised.
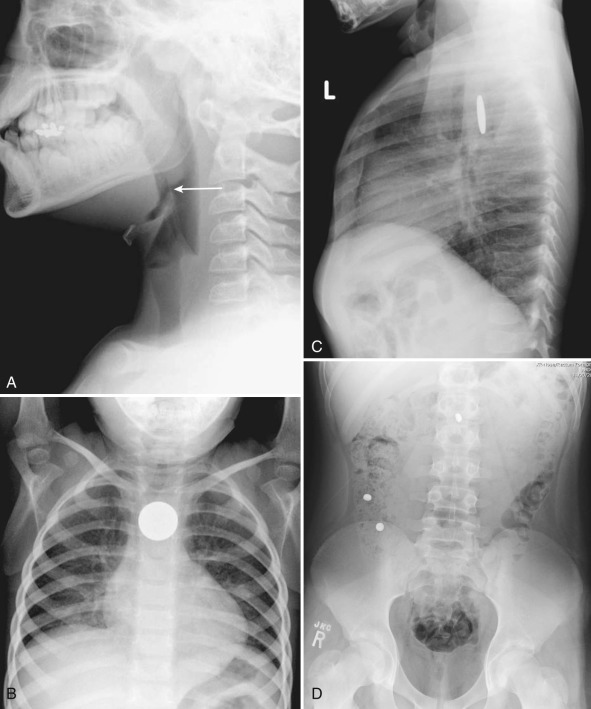
In a child with upper respiratory symptoms and suspected foreign body ingestion, radiographic findings may be normal in about one third of cases. When a radiopaque foreign body is not seen, an indirect sign of airway obstruction is differential hyperinflation of the affected lung related to air trapping. This can be diagnosed with expiratory radiographs, or alternatively bilateral decubitus views for young children who cannot hold their breath on command [ Fig. 2.22 ]. Other indirect radiographic findings of a foreign body aspiration include regional hyperinflation of a lung lobe and peripheral opacities reflecting atelectasis or consolidation distal to the site of obstruction.
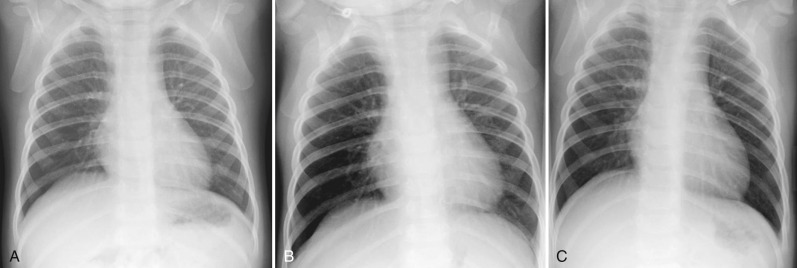
Pharyngeal and laryngeal foreign bodies can lead to airway obstruction and respiratory distress at the time of aspiration. Thus once a foreign body has been identified, flexible or rigid bronchoscopy is indicated to retrieve the aspirated object.
Neoplasms
Subglottic Hemangioma.
Subglottic hemangioma is a rare, benign vascular neoplasm characterized by a proliferative phase of rapid growth during the first 6 to 18 months of life followed by spontaneous involution. Patients are typically symptomatic by 6 months of age due to progressive airway narrowing during the proliferative phase. Symptoms resolve after involution. Presenting symptoms include hoarseness and an abnormal cry. In about 50% of cases, associated cutaneous hemangiomas are seen. Subglottic hemangiomas can be seen as a component of the PHACES syndrome ( p osterior fossa brain malformations, h emangiomas, a rterial anomalies, c ardiac defects, e ye abnormalities, s ternal clefts and s upraumbilical raphe).
Inspiratory AP and lateral radiographs of the neck demonstrate asymmetric subglottic tracheal narrowing [ Fig. 2.23 ]. The modalities of choice for complete assessment of a clinically suspected subglottic hemangioma are contrast-enhanced CT and MRI. Cross-sectional studies will show an enhancing submucosal mass that may be circumferential, bilateral, or unilateral, with posterolateral positioning most common [ Fig. 2.23 ]. Definitive diagnosis is made with direct laryngoscopy or bronchoscopy.
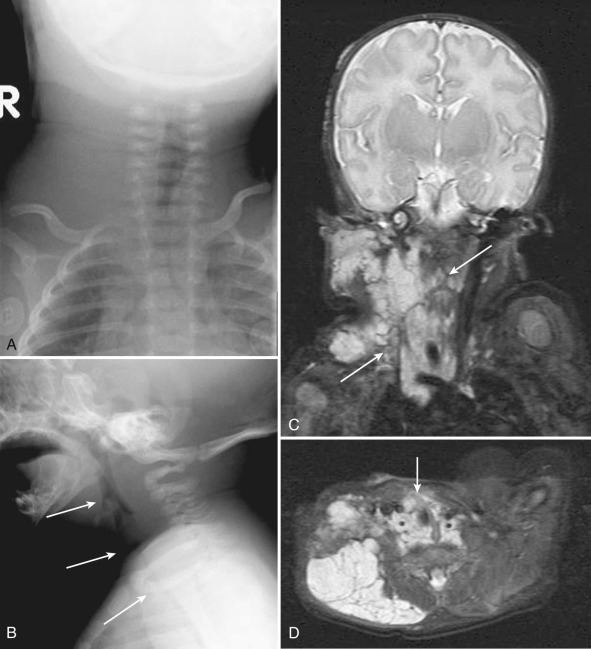
Management varies depending on severity of symptoms. Patients without respiratory or feeding difficulties may be managed conservatively. Symptomatic patients can be treated with systemic and intralesional corticosteroids, CO 2 laser therapy, a trial of interferon, vincristine, or propranolol, or direct excision.
Laryngeal Papilloma.
Laryngeal papilloma is the most common benign neoplasm of the larynx in children. In the majority of cases, multiple papillomas are seen in the trachea. Because of the high recurrence rate, this entity is also called recurrent respiratory papillomatosis (RRP). About half of affected patients have a maternal history of condyloma acuminatum. This condition is most often caused by the human papilloma virus types 6 and 11 and is characterized by the proliferation of benign squamous papillomas throughout the aerodigestive tract. Although it is benign, RRP can have an aggressive clinical course in children. It can be fatal because of its tendency to recur and spread throughout the aerodigestive tract.
Children usually present around age 2 or 3 years with worsening hoarseness and stridor. Less commonly, patients can present with chronic cough, recurrent pneumonia, failure to thrive, dyspnea, dysphagia, or acute respiratory distress associated with an upper respiratory tract infection.
RRP can be seen as an irregular filling defect in the glottis on lateral radiographs of the neck soft tissues [ Fig. 2.24 ]. Chest radiographs are often normal, particularly if lesions are confined to the larynx. With extension into the subglottic region and seeding of the respiratory tract, pulmonary nodules may develop. Nodules may be solid or cavitary. When papillomas result in airway obstruction, atelectasis, bronchiectasis, and mucous plugging can be seen. On CT, thin-walled cysts with adjacent nodules are visualized. Diagnosis is confirmed with direct laryngoscopy and biopsy.
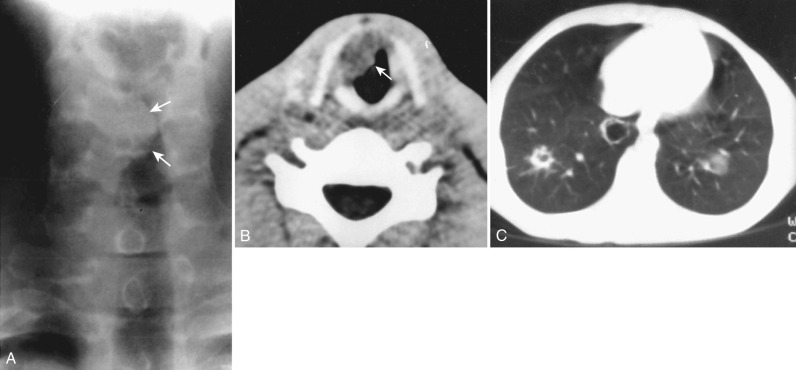
Laser ablation of laryngeal or airway lesions can be performed for debulking. Repeated procedures may be needed because of the frequency of recurrence of these tumors. Interferon and antiviral agents may slow growth but are not curative. There is a small risk for malignant degeneration into squamous cell carcinoma.
Lower Airway
Tracheobronchomalacia
Tracheobronchomalacia (TBM) is characterized by abnormal collapse of the airway in expiration. It results from abnormal, weakened cartilaginous support of the airway and/or the membranous portion of the posterior tracheal wall. It may be congenital (primary) or acquired (secondary), and it most commonly occurs in young infants in the first year of life because of tracheal flaccidity. Primary TBM results from incomplete development of the tracheal cartilage, and it can involve the entire length of the airway. Secondary TBM may be related to a history of infection, prior surgery, extrinsic compression from cardiovascular structures, or neck and mediastinal tumors. TBM related to tracheal underdevelopment or inflammation is often seen in children with EA and TEF. Children with TBM characteristically present with expiratory wheezing that increases with crying or feeding, cough, stridor, and/or recurrent respiratory infections.
In complex or severe cases of secondary TBM, dedicated expiratory-phase CT imaging can be performed to detect excessive (>50%) collapsibility of the trachea and bronchi, diagnostic of this condition [ Fig. 2.25 ]. The most common findings during dynamic expiration are tracheal collapse and crescentic bowing of the posterior membranous trachea. In older patients, a cine CT can be combined with a coughing maneuver. This is the most sensitive method for eliciting tracheal collapse.
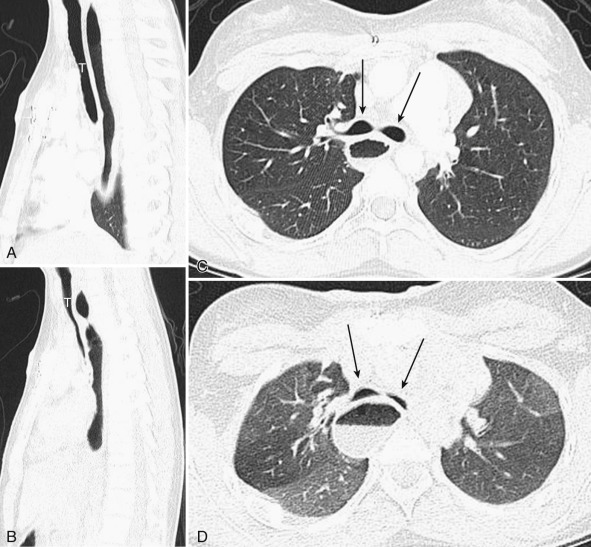
For children with mild-to-moderate TBM, conservative management is preferred because symptoms often resolve by 1 to 2 years of age with maturation and strengthening of the tracheal cartilage. For children with more severe symptoms, more aggressive treatment options may be needed. These can include continuous positive airway pressure (CPAP), tracheostomy placement, stent placement, or surgical intervention.
Tracheoesophageal Fistula
TEF results from incomplete or abnormal division of the developing trachea from the ventral foregut during embryogenesis. There are five major anatomic variations of esophageal atresia-tracheoesophageal fistula (EA-TEF) [ Fig. 2.26 ]. The level of the fistula varies, but it is most commonly near the carina. There is an increased incidence of TEF in children with Down syndrome. It is also seen with increased incidence in children with VACTERL ( v ertebral anomalies, a nal atresia, c ardiac abnormalities, T EF and/or E A, r enal agenesis and dysplasia, and l imb defects).
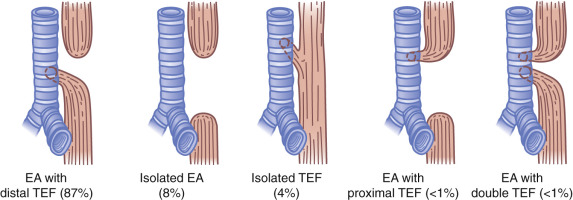
Infants with most forms of EA-TEF present shortly after birth. The exception is the H-type TEF, which may go undiagnosed until late childhood. Symptoms typically include coughing, gagging, cyanosis, vomiting, copious oral secretions, and/or respiratory distress.
Imaging findings depend on the type of EA-TEF. The diagnosis may be suggested on prenatal ultrasound as early as 24 weeks’ gestation. Polyhydramnios, absence of a fluid-filled stomach, a small abdomen, lower-than-expected fetal weight, and a fluid-filled, distended esophageal pouch may be seen. Fetal MRI can be obtained to confirm the diagnosis. Postnatally, EA is suggested by the presence of an air-filled, distended upper esophageal pouch on chest radiographs. Clinically, failure to pass a nasogastric tube occurs, with coiling of the tube in the esophageal pouch [ Fig. 2.27 ].
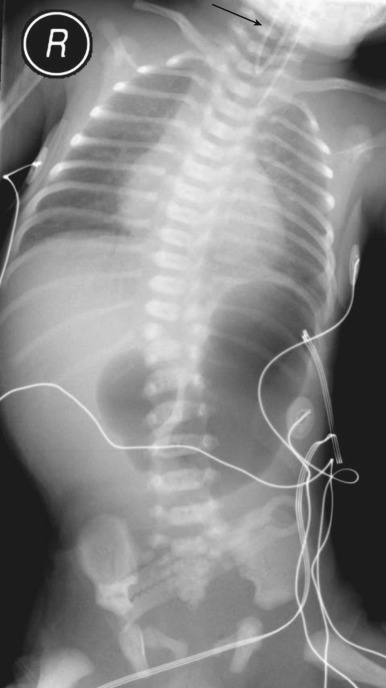
Initial postnatal intervention is aimed at minimizing the risk for aspiration pneumonia. Surgical repair consists of closing the fistula, with primary anastomosis of the proximal and distal ends of the esophagus if there is a short-segment atresia. When there is a long-segment atresia or EA without a TEF (type 2), a colonic interposition may be performed. This procedure has varied success rates.
Long-gap EA has also been managed successfully with growth induction via the Foker procedure. In this procedure, sutures are applied to the proximal and distal ends of the esophagus, with a tag on each end. The sutures are externalized and attached to traction devices outside the patient’s body. With the patient paralyzed, increasing traction is applied over time, creating stress and leading to natural tissue growth. When growth is determined to be adequate, the proximal and distal ends of the esophagus are connected with a primary anastomosis. After EA-TEF repair, an esophagram is often performed to assess for surgical complications such as anastomotic leak or stricture, recurrent TEF, or esophageal dysmotility.
Asthma
Asthma is characterized by acute, subacute, or chronic paroxysmal airway inflammation caused by hyperreactivity. It is at least partially reversible. It primarily involves the medium-sized and small bronchi, which are thickened due to edema, hyperplasia of the bronchial wall smooth muscle, and an increase in the size of the airway mucosal glands. Airway hypersensitivity can be related to a number of factors, including viral illnesses, allergens, exercise, medications, and environmental conditions. Symptoms include wheeze, cough, chest tightness, and shortness of breath. Approximately 50% of children suffering from reactive airways disease develop symptoms before the age of 2 years, with 80% to 90% diagnosed by 5 years of age.
Chest radiographs may demonstrate pulmonary hyperinflation, with peribronchial thickening and areas of segmental atelectasis, similar to the findings seen in bronchiolitis [ Fig. 2.2 ]. However, the chest radiograph of a child with an asthma exacerbation is most often normal. Radiographs are important as part of the initial diagnostic workup to exclude complications of asthma, which may include pneumomediastinum and pneumothorax. CT can be performed to diagnose associated conditions that may occur, such as allergic bronchopulmonary aspergillosis. In this condition, CT demonstrates central multifocal bronchiectasis with areas of mucoid impaction (“finger-in-glove” appearance), centrilobular nodules, mosaic perfusion caused by air trapping, areas of consolidation and ground-glass opacification, and atelectasis related to bronchial obstruction [ Fig. 2.28 ].
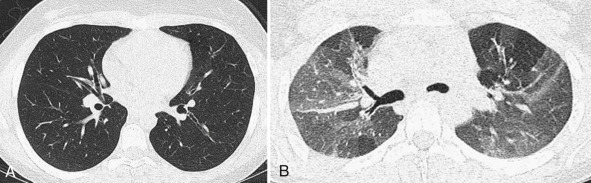
With appropriate management of asthma, prognosis is usually excellent. Treatment includes avoidance of known triggers and exacerbating factors, inhaled beta-agonists for bronchospasm, corticosteroids, and inhaled mast cell stabilizers.
Rings and Slings
Vascular rings and pulmonary artery sling are congenital anomalies of the great vessels that can cause stridor in infancy and childhood. They are caused by abnormal development of the embryonic aortic arches. A vascular ring completely encircles the trachea and/or esophagus. A pulmonary artery sling encircles the trachea only.
The embryonic development of the normal aortic arch system was first described by Edwards in 1948. Paired right and left dorsal aortae arising from the truncus arteriosus are present by approximately 21 days gestational age. They are connected by six primitive aortic arches that correspond to the six branchial arches. Early on in development, the first, second, and fifth primitive aortic arches regress. The third arches become the carotid arteries, and the left sixth arch becomes the ductus arteriosus. The right sixth arch will normally regress. The fourth arch forms the proximal portion of the subclavian artery on the right and the major transverse aortic arch segment on the left. The paired dorsal aortae and six primitive arches undergo a structured process that results in a left aortic arch with the normal branching order (the innominate artery followed by the left common carotid and subclavian arteries) and a descending thoracic aorta to the left of the spine. Atresia of segments that should normally persist or persistence of segments that should normally regress can result in complete encircling of the trachea, which may lead to compressive airway obstruction.
In the setting of a vascular ring, there may be displacement or compression of the tracheal air column. This can be detected on radiographs of the neck and chest. When extrinsic compression of the esophagus is detected on a barium swallow or upper GI series, follow-up CTA or MRA of the chest is warranted to delineate the vasculature causing compression of the esophagus and possibly also the airway. Cross-sectional studies provide valuable information necessary to guide surgical management.
In general, respiratory symptoms predominate in the initial presentation of patients with a vascular ring or sling. Stridor is present in almost all cases and is more pronounced with feeding or activity. The three most important vascular causes of stridor are double aortic arch, right aortic arch with aberrant left subclavian artery, and pulmonary sling. Vascular rings may present with dysphagia when there is relatively more compression of the esophagus than the trachea, although this is much less common.
Double Aortic Arch
The most common symptomatic vascular ring is a double aortic arch. This results from persistence of both the right and the left dorsal aortae arising from the ascending aorta. The right and left aortic arches pass to either side of the trachea and esophagus, and join posteriorly to form a single descending thoracic aorta. The right arch is larger than the left in about 75% of cases and is typically also more superior in position.
On a frontal view of the chest, the right aortic arch may cause deviation of the trachea toward the smaller left aortic arch. On a lateral view, anterior bowing of the trachea may be seen [ Fig. 2.29A and B ]. A double aortic arch should be suspected if there is posterior and bilateral extrinsic compression of the esophagus on a barium esophagram or upper GI series. More precise delineation of arch morphology and the degree of tracheal compression requires preoperative planning with an MRA or CTA [ Fig. 2.29C through E ]. These cross-sectional studies will show the double arch encircling both the trachea and the esophagus. Right versus left arch dominance can be determined, and any existing coarctation or narrowing in either arch can be identified. The nondominant or narrowed arch and the ligament of the ductus arteriosus are surgically ligated and divided to relieve the compression of the airway.
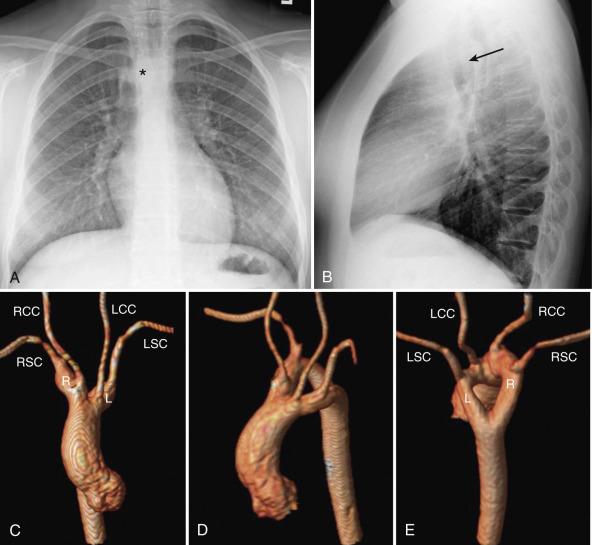
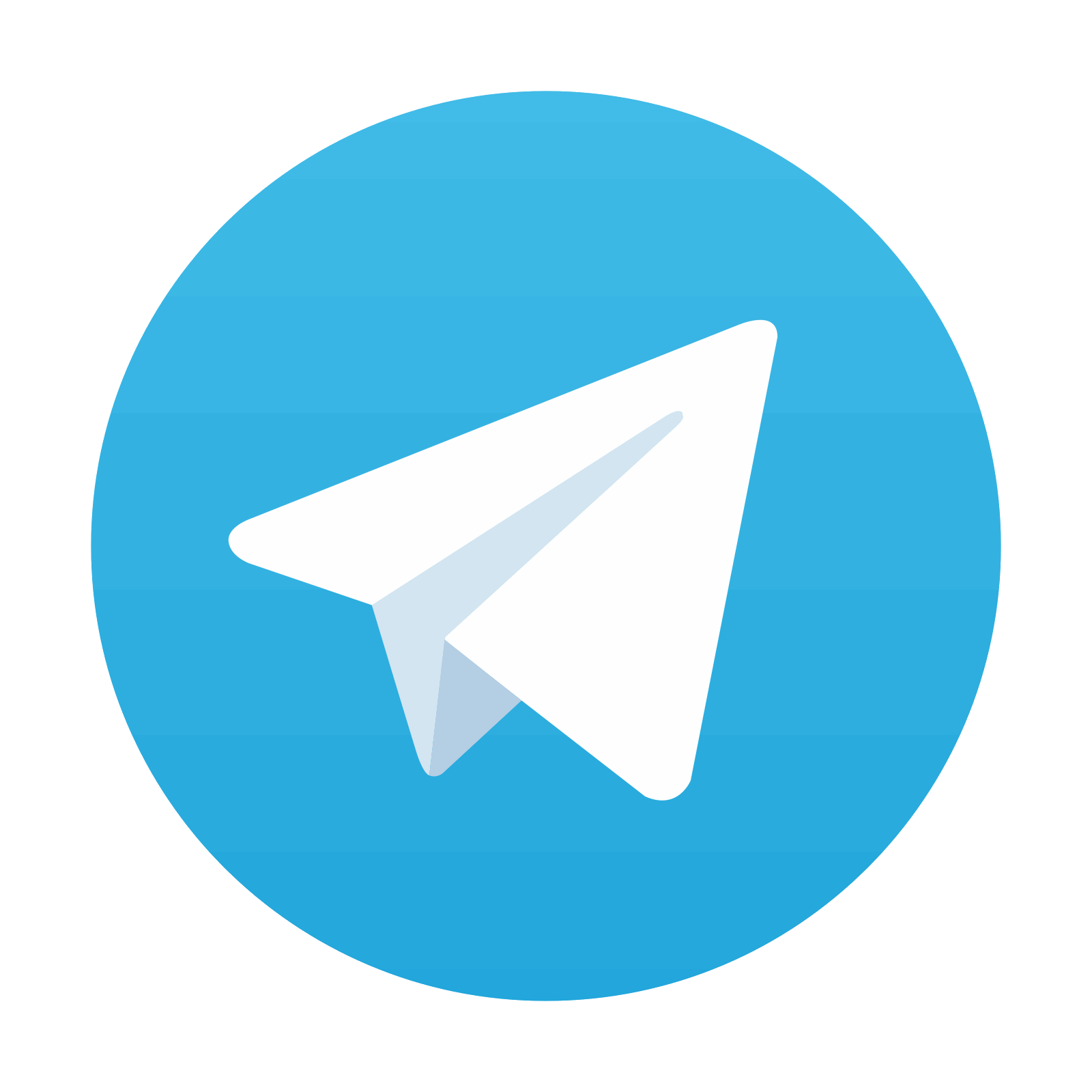
Stay updated, free articles. Join our Telegram channel

Full access? Get Clinical Tree
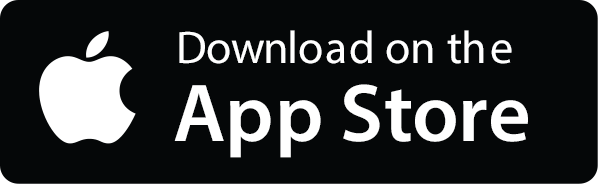
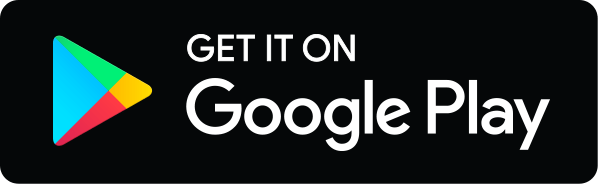
