Global N = 206
Group under analysis
All grades
Grade 4
Radiation pneumonitis
206 treatments
1 (0.4 %)
0
GI ulcers
215 procedures
3 (1.4 %)
0
REILD (global)
206 treatments
24 (11 %)
6 (2.9 %)
REILD (since Sep 2006)
132 treatments
7 (5.3 %)
0
It can be easily appreciated that a learning curve of patient selection and treatment design significantly reduced the incidence of liver toxicity that is the most relevant adverse event after Y-90 RE.
2 Evaluation Work-Up
2.1 Angiography
In 55–65 % of the cases, the liver arterial vascularisation comes exclusively from the celiac trunk (CT). Usually, the CT trifurcates into the splenic artery (SA), the left gastric artery (LGA) and the common hepatic artery (CHA). In 90 % of the cases, the CHA gives off the gastroduodenal artery (GDA). After doing so, the hepatic artery is named the proper hepatic artery (PHA) (Salem et al. 2008).
2.1.1 Liver Vascular Patterns and Definitions
During the embryonary development, the arterial supply to the liver comes from three major trunks: the CHA, the LGA (the gastro-hepatic trunk) and the superior mesenteric artery (SMA) (the hepato-mesenteric trunk). As a result of anomalies during the embryonary process, the liver may have “aberrant” arteries that come from several sources different from the CHA and the CT. The first known study about variations in the “normal pattern” of the liver arterial branching was presented in the 1950s by Michels (1955). He described up to 10 anatomical variations including the Type I, which represents the standard (normal) anatomy. Aberrant arteries were defined as “replaced” when the entire lobar or segmental vascularization is supplied by an artery whose origin is other than the PHA. “Accessory” arteries are those in which a portion of the liver lobe or segment is supplied by an aberrant artery, no matter the size, and there is an artery that comes from the PHA to the same lobe or segment. The widespread use of refined imaging techniques, such as superselective angiography or computed tomography angiography (CTA) has demonstrated that there are several variants in the arterial liver anatomy which were not defined by Michels, however, his classification still remains as the basis for the description of the arterial patterns.
Potential complications, such as gastroduodenal ulceration, may appear following the inadequate delivery of Y-90 microspheres. Therefore, a thorough study of vascular variants must be performed before performing Y-90 RE procedure (Carretero et al. 2007). Furthermore, the poor response of some liver tumors to Y-90 RE may not be related to the ineffectiveness of the procedure but to the lack of target afferent artery recognition.
2.1.2 Technique
Because of the above mentioned reasons, and some other that will be presented later, a careful angiographic technique has to be performed, including selective studies, “angiographic interrogations”, of each vessel that may supply the liver parenchyma. If possible, it may be of great help to evaluate any sectional imaging studies (preferably a CTA) before performing the catheter arteriography. Also, if deemed necessary, a CTA should be performed with the catheter in place and with a selective arterial injection of contrast (this possibility is now more frequently performed with the C-arm CT systems) (Wallace et al. 2007). Some authors recommend initiating the angiography with and abdominal aortography performed with a pigtail catheter. With this approach, any abdominal vessel (CT, SMA, phrenic, intercostal, renal, adrenal, etc.) that may go to the liver can be opacified. Others directly catheterize the SMA in order to detect any hepatic vessel that may arise from this trunk and last, but not least, perform and indirect portography with the aim to evaluate the portal morphology and its flow pattern. The CT is catheterized (sidewinder or C-shaped 4 or 5 F catheters) and an angiography is then performed. This is an important step since placing the catheter directly into the CHA could miss a lot of anatomical and functional (hemodynamic) information. Selective hepatic angiographies should be performed with coaxial (2.3–2.8 F) catheters. The use of such microcatheters is recommended because of the “vessel interrogation”, and the information obtained, is not compromised by the size of the regular 4–5 F catheters.
2.1.3 Anatomy
2.1.3.1 Hepatic Arteries
As said before in a number of cases, not yet well-established but that could be around 45 %, there are aberrant hepatic arteries that may arise from places different than the PHA. According to Michels, the most commons variations are the presence of replaced arteries. Type II (10 %) is a left replaced hepatic artery from the LGA and Type III (11 %) is a right replaced hepatic artery from the SMA. Type IV (1 %) is defined when both replaced arteries are present. Types V–VIII (18 %) consist in accessory arteries from different origins. Finally, Types IX and X are diagnosed when there is and aberrant origin, different that the CT (SMA or LGA), of the CHA. These anatomical variations should be evaluated, if available, with a CTA.
Once the origin of any hepatic artery has been detected, a selective angiography of every branch should be performed. The angiographic study (interrogation) must answer three important questions: (1) Search of the tumoral afferences, (2) Evaluation of tumoral hemodynamics, and (3) presence of extrahepatic branches that may arise from hepatic arteries (Liu et al. 2005; Lewandowski et al. 2007).
(1)
Although CT and MR can precisely localize the segments/lobe in which the tumor is placed, it is not infrequent to have arterial afferences, from unexpected origins, with several arteries to a specific tumoral area. Among many others, there are two clear examples of such situation. A tumor located in segment I may receive arteries from the left, the right and/or the proper hepatic arteries (Yoon et al. 2008). Lesions involving segment IV can receive afferences from the left hepatic (segmental arteries of S4a and S4b), the middle (an artery arising directly from the right hepatic) and/or the right hepatic (segmental artery of SVIII) branches. Most Y-90 RE procedures are performed with the infusion of the microspheres in just one or two arterial pedicles. This means that in cases of multiple afferent vessels to the whole tumoral volume, a “redistribution” of the arterial flow should be performed. When a lobar/segmental artery is occluded with microcoils at its origin, the distal branches will receive their blood supply from the nearby lobe or segmental arteries by the development of intrahepatic communicating channels. These vessels, also called “connecting arcades” consist, in most cases, the peribiliary arterial network (Bilbao et al. 2010; Gunji et al. 2006).
(2)
A power injection at the rate of 4 ml/sec. with a 4 F catheter placed at the CH, is an excellent way to depict the morphology of any artery that is opacified. However, it is important to realize that, for any endovascular procedure, the rate at which particles (bland or chemo-embolization and Y-90 RE) are hand-injected is quite different. The agent may not be exactly delivered into the desired artery. In other words, you may advance the tip of the microcatheter into an artery from which you can opacify the tumor on power injections, but the particles may not necessarily reach the tumor when hand delivered. This is the case of some hypervascular tumors, mainly hepatocellular carcinomas, in which the tumoral hemodynamics need to be carefully evaluated before the infusion of Y-90 microspheres. The operator needs to be aware of such situation in order to deploy the material correctly within the tumor.
(3)
Liver arteries give off many non-hepatic arteries that need to be carefully explored and, if deemed necessary, occluded with coils or microcoils. In the absence of previous surgery, this embolization is safe and the distal territories may receive the blood from other, non-hepatic vessels. The most common artery that should be occluded is the GDA (92 %). A paper published by Song demonstrates that non-hepatic vessels may be detected in as much as 82 % of patients with hepatocellular carcinoma (Song et al. 2006). Most of these vessels arise from the left hepatic artery. If embolization of the GDA is going to be performed, the recommendation is to place the coils precisely at its origin. If not, small non-detected supraduodenal collaterals may develop and revascularize the pyloric area. An exception of this rule would be those cases in which and accessory right hepatic artery has its origin in the GDA, in these cases, the embolization may be performed preserving the flow in this vessel. The second most common extrahepatic vessel is the right gastric artery. Although infrequent, there could be two and even three right gastric arteries. Its origin is, in most of the cases, situated in the left hepatic artery or in the distal part of the PHA. Other less common sources are the GDA and the CHA. This artery should be occluded because, if included in the area to be treated, there is a high probability of gastric ulceration. It is sometimes technically difficult to get into that artery safely enough to deploy the microcoils; the reason is that there could be a sharp angulation of its initial segment. In this situation an alternative could be to place the microcatheter from the LGA through the communication, almost always present, between both vessels along the lesser gastric curvature (Cosin et al. 2007) (Fig. 1).
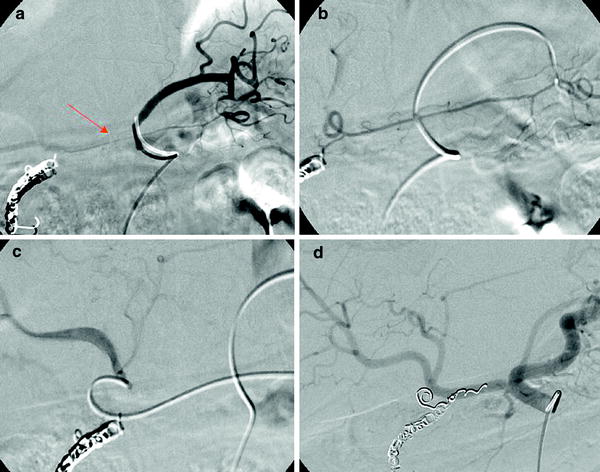
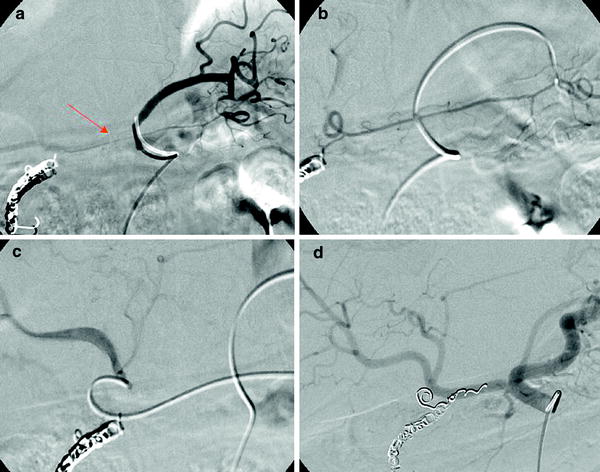
Fig. 1
a Left gastric angiography with retrograde opacification of the right gastric artery (arrow). b The catheter has been placed in the right gastric artery. c A microcatheter has been placed at the origin of the right gastric artery close to its origin in the left hepatic artery. d Hepatic angiography performed after the occlusion of the right gastric and the gastroduodenal arteries
There are several other extrahepatic arteries that give off from the left hepatic artery. Among them, the accessory LGA and the accessory left phrenic artery. These arteries may not be easily detected in a conventional arteriography. As a technical recommendation, images of selective angiographies of the left hepatic artery should be taken including the left hypocondrium and any vessel that goes beyond the left lobe (a possible phrenic artery) should be explored. Images should also be obtained in late venous phase since the opacification of portal branches may indicate that a gastric or an esophageal branch has its origin in the left hepatic artery.
The falciform artery vascularises the anterior abdominal wall alone or in connection with the distal branches of the internal mammary and the epigastric arteries. If present it should be occluded. Several authors have described the appearance of cutaneous rash, and even, necrosis of the anterior abdominal wall if particles pass through this vessel. The origin of the falciform artery is situated, mainly, in the branch of segment IVa no matter if this branch has its origin in the left or in the middle hepatic artery (Kim et al. 1999) (Fig. 2).
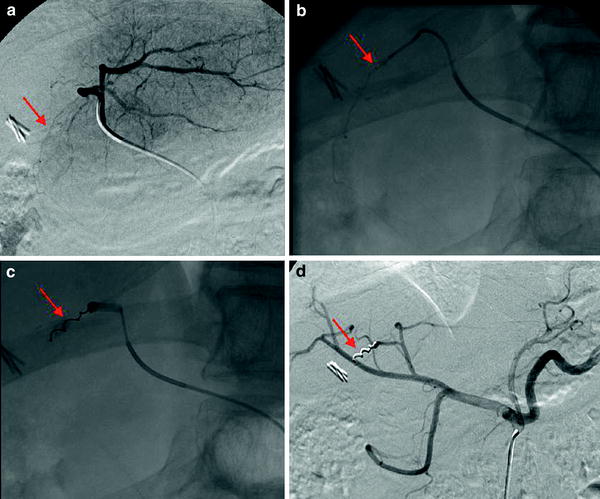
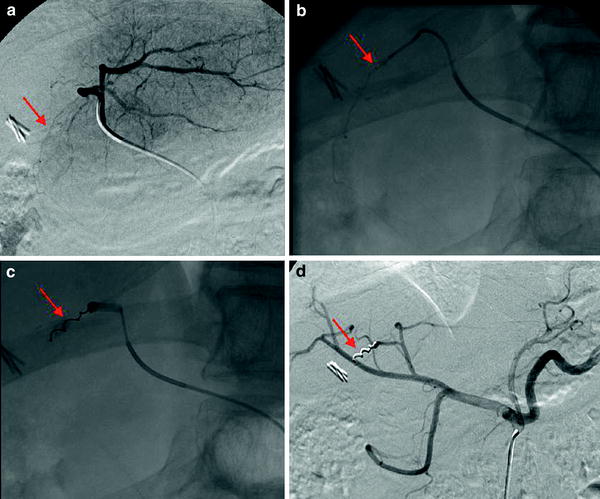
Fig. 2
a Selective injection in the left hepatic artery. The falciform artery is opacified (arrow). b and c The tip of the microcatheter has been placed at the origin of the falciform artery (arrow) and a micocoil has been deployed (arrow). d Hepatic angiography performed after the occlusion of the falciform artery (arrow)
Some experienced interventional radiologists say that in spite of feeding the liver parenchyma, the left and the right hepatic arteries are two completely different vascular territories with their own peculiarities. The left artery has, as seen, some specific extrahepatic arteries and the right has the peculiarity of feeding the gallbladder vascularization. It is known that the cystic artery, a single and terminal branch, arises from the right hepatic artery. However, this statement is true in a minority of cases, since the cystic artery/arteries may come from different segmental branches from the right, the left or the GDA, in this case with a common trunk with the right gastric artery. The gallbladder vascularization may also come from the biliary plexus or the SMA. Radiation induced cholecystitis requiring surgery has been described in cases where there was passage of microspheres into the gallbladder. How to avoid this complication?, the answer is not easy, because cystic artery embolization in cases of terminal vascularization of the gallbladder may induce an ischemic cholecystitis. Also with non-terminal vascularization, if the cystic artery is occluded, an accessory artery may develop from a distal hepatic branch and the complication may not be prevented. The easiest solution could be to place the tip of the catheter distally to the origin of the detected cystic artery although the operator could select other alternatives in a case-by-case manner. It is worth remembering that perforator arteries from parenchymal branches may reach the gallbladder. A reverse flow may take place and represent the major afferent vessels to the liver tumor. The right hepatic artery gives off another vessel that needs to be explored: the retroduodenal or posterior pancreato-duodenal artery. The origin of this artery is situated in the right hepatic or a segmental branch. If patent, it connects with the inferior pancreato-duodenal arcade (SMA) so it can be occluded by using both approaches.
2.1.3.2 Non-hepatic vessels
The liver parenchyma can receive arterial vascularization from branches different than the previously described as “hepatic arteries” (Miyayama et al. 2006). These arteries may enter from the dorsal (posterior) surface of the liver. This area, the bare area, is not covered by peritoneum and is in direct contact with the retroperitoneum. If they are involved in the tumoral vascularization, all these arteries should be occluded in order to redistribute its distal flow, which then may come exclusively from liver arteries. In the presence of tumors adjacent to the bare area (segments IV and VIII mainly, but also the segment I) several arteries need to be interrogated. In theory, all arteries that vascularise the diaphragm and the adrenal area need to be explored. The first are the inferior phrenic arteries (mainly the right). Tumoral vessels may enter the liver from their two main trunks, the superior suprarenal and the distal phrenic branches. Distal phrenic branches may also come from the right internal mammary artery and intercostal arteries. Adrenal branches may have its origin not only in the inferior phrenic but also in the middle adrenal and the renal/capsular arteries. If the tumor is close or has “ruptured” the liver capsule, it may be possible to have afferent vessels that may enter the liver tumor through the disrupted liver surface. The most common vessels involved in such situation are the omental/epiploic vessels. There are two gastroepiploic arteries, the left one is more distal branch of the SA and the right one represents the distal branch of the GDA. So, in cases of large tumors situated in segments VI and VII, before performing any “prophylactic” embolization of the GDA, it is necessary to explore its epiploic branches. If the GDA embolization is done too proximally, some tumoral areas may still be fed from the inferior pancreatoduodenal arteries (SMA) and this area will only be partially treated from the right hepatic approach. For the same anatomical reason, in some cases, colonic branches may also reach the liver.
The final aim of the angiographic study is to deliver the microspheres safely into the whole tumoral volume that has to be treated. The scope of the anatomical evaluation of any hepatic, extrahepatic, and nonhepatic arteries is to skeletonize (a surgical definition) the tumoral afferent vessels and to avoid potential complications. The purpose of the hemodynamical study, which at this moment is only a rough evaluation, is to deploy the particles within the small tumoral feeding vessels that are around or inside the lesion.
2.2 Sham Procedure Using Tc-MAA
Once the anatomy has been established, the angiographic evaluation must be accomplished with 111–185 MBq of Tc-MAA injected into the vessel of interest, in order to mimic the microsphere application during the treatment. The MAA particles (average size of 35 μm and none greater than 150 μm) are approximately similar in size to the SIR-Spheres™ microspheres (29–35 μm), and smaller than TheraSphere™. Therefore, Tc-MAA scan could be used as sham procedure to indicate approximate distribution of Y-90 microspheres (imaging and measuring) to be expected during treatment. For this purpose, the same procedure is performed for both SIR-Spheres and TheraSphere and gives an accurate estimation of Y-90 microspheres distribution.
On the other hand, Tc-MAA scan allows the physician to plan the RE therapy and to detect any occult arteriovenous shunt from the hepatic arterial system to the pulmonary or gastrointestinal venous systems and so identify potentially dangerous misplacement of the microspheres (Fig. 3).
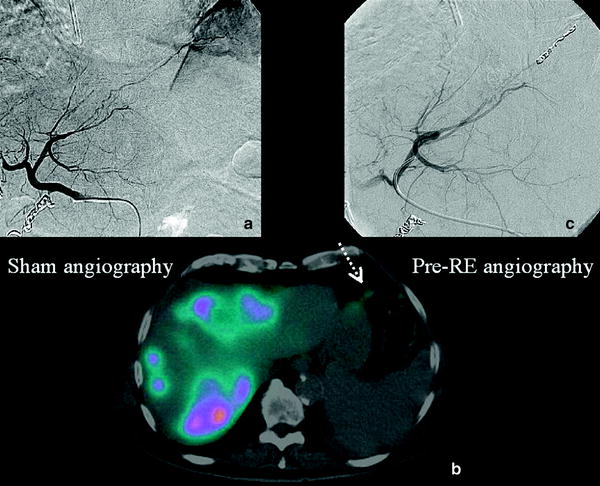
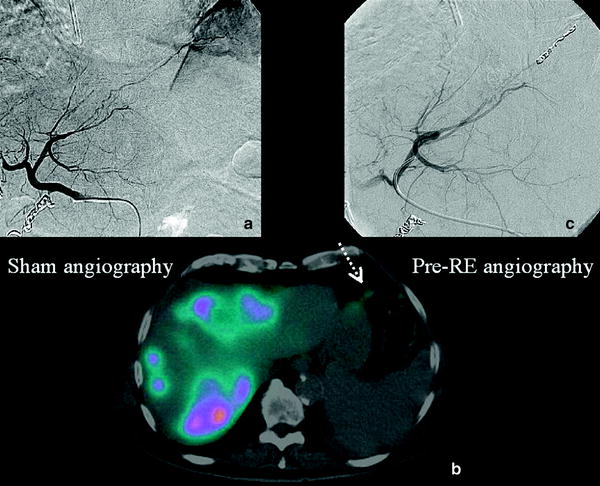
Fig. 3
a Pre-treatment angiography: Frenic artery coming from the left hepatic artery. b Tc-MAA SPECT/CT. Fused images show an Tc-MAA uptake in the diaphragmatic muscule (spotted arrow). c Selective catheterization of the left hepatic artery wich is subsequently entered with a microcatheter in order to coil it, thus preventing non-target radioembolization
2.2.1 Calculation of Hepatopulmonary Shunting
A feature of the neoplastic vasculature within tumors is the formation of arteriovenous anastomoses or shunts. A high pulmonary shunting is most frequently encountered among patients with hepatocellular carcinoma or metastatic disease with large tumor burden (Leung et al. 1995). Measurement of this hepatopulmonary shunt is essential for ensuring the suitability of the procedure and calculating the dose to be administered. This is done at the same time as the diagnostic angiogram by injecting Tc-MAA through the catheter placed in the feeding arteries. In the presence of detectable hepatopulmonary shunts, a proportion of the microspheres would bypass the hepatic capillaries and end up in the pulmonary capillary mesh. Because lung tissue is sensitive to radiation, administering microspheres in the presence of a sizable shunt increases the risk of clinically significant radiation pneumonitis. Radiation pneumonitis is an inflammatory reaction that resembles pneumonia and the symptoms include dry cough, progressive dyspnea, and restrictive ventilation deficits resulting in deteriorating lung function, and in the worst case, even death 1 month after SIRT. Heparin-resistant thromboembolic incidents could appear together with the pneumonitis.
Using a gammacamera, the amount of Tc-MAA in the lung can be quantitated and compared to the injected dose, allowing for calculation of hepatopulmonary shunt (Fig. 4).
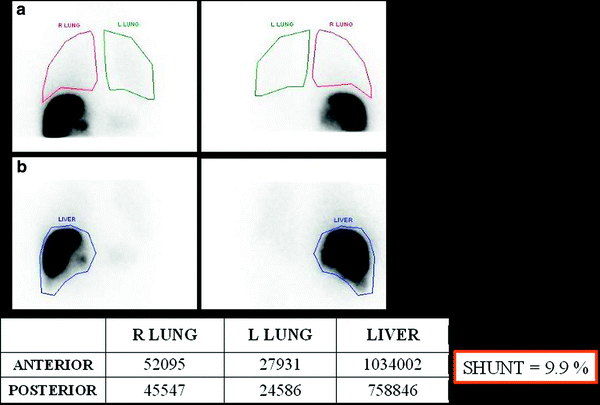
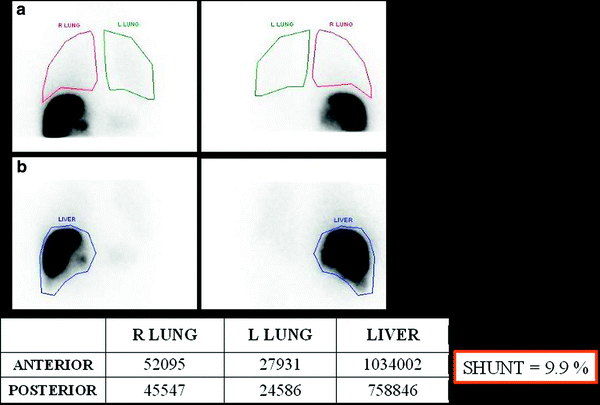
Fig. 4
Liver–lung shunt calculation using the values obtained in the Tc-MAA. ROIs are drawn in planar images covering both lungs (a) and the liver area (b). Geometrical mean of these areas should be calculated to calculate the lung shunt
The percentage of lung shunting can be determined from the total counts within regions of interest over both lobes of the lung and the liver, using the geometrical mean of anterior and posterior thoracic and abdominal planar images.
![$$ {\text{L}}\left[ {{\text{Lung shunt}}\;\% } \right.\left[ {\text{ Lung shunt }} \right]\; = \;\frac{{{\text{ROI Lung shunt}}\; \times \;100}}{{{\text{ROI Lung counts}}\; + \;{\text{ROI Liver counts}}}} $$](/wp-content/uploads/2016/09/A174_2012_793_Chapter_Equa.gif)
![$$ {\text{L}}\left[ {{\text{Lung shunt}}\;\% } \right.\left[ {\text{ Lung shunt }} \right]\; = \;\frac{{{\text{ROI Lung shunt}}\; \times \;100}}{{{\text{ROI Lung counts}}\; + \;{\text{ROI Liver counts}}}} $$](/wp-content/uploads/2016/09/A174_2012_793_Chapter_Equa.gif)
Because the shunt fraction estimate is significantly affected by the estimation procedure used, a geometric mean analysis with a hepatic region of interest (ROI) is most frequently employed. This ROI is obtained by increasing the image intensity to include most of the scatter originating from that organ. All ROI counts are corrected for background obtained from the abdominal region below the liver, preventing the urinary tract.
The estimated dose (Gy) to the lungs can be predicted by the Tc-MAA shunt fraction and the injected activity of the radiomicrospheres, with the following equation (TheraSphere yttrium-90 glass microspheres users manual; Salem and Hunter 2006).
where

A i
activity infused
LSF i
lung shunt fraction during infusion
n
number of infusions, and approximate vascular lung mass = 1 kg.
Previous preclinical and clinical studies with Y-90 microspheres demonstrated that the highest tolerable dose to the lungs is 30 Gy for a single injection, and 50 Gy for multiple injections (Leung et al. 1995). In cases where several treatments are administered, the lung dose is the cumulative absorbed lung radiation dose from all treatments and should not exceed 50 Gy. Depending on the shunt volume, a reduction in the total administered dose to the liver is necessary or Y-90 RE is even contraindicated (Sirtex medical training manual, training program physicians, and institutions).
2.2.2 Extrahepatic Vessels (Unnoticed Collateral Vessels)
To perform any therapeutic transarterial procedure in the liver in a safe and efficient manner, one should be acquainted with the hepatic arterial anatomy (Covey et al. 2002). This is particularly important when microspheres could be inadvertently deposited in excessive amounts in organs other than the liver such as stomach, duodenum, gallbladder, pancreas, mesentery, and also in vascular structures such as the falciform artery. Serious complications include gastrointestinal ulceration, bleeding, gastritis, duodenitis, cholecystitis, pancreatitis, radiation dermatitis, and pneumonitis (Leong et al. 2009; Riaz et al. 2009; Liu et al. 2005).
Due to free technetium, thyroid gland and often the stomach may be seen in Tc-MAA images, which usually seem confusing. A pathologic uptake in the stomach should be ruled out in such cases before the treatment. To avoid this accumulation, scintigraphy should be performed within 1 h of injection of Tc-MAA. Receiving 600 mg perchlorate per os 30 min before angiography seems to prevent also “unspecific” uptake of Tc-99m pertechnetate in the thyroid and stomach (Ahmadzadehfar et al. 2010). Anyway, overall training, careful patient selection, meticulous pretreatment assessment, and coiling of relevant vasculature reduce complication rates.
2.2.3 Advantages of SPECT or SPECT/CT
Although most reports have focused on planar Tc-MAA scintigraphy, the addition of 3-D acquisition (SPECT) provides valuable additional information. SPECT images not only allow one to identify metastases and evaluate the arterial perfusion of single lesions without overlap, but also form the basis for image fusion with other pre- and post-therapeutic SPECT, PET, CT, or MRI images (Koch and Tatsch 2008). Additionally, the increasing availability of new multimodality SPECT/CT devices with advanced technology offers the opportunity to shorten acquisition time and to provide accurate fusion imaging and attenuation correction. SPECT/CT has been clearly shown superior over planar scintigraphy or SPECT for the imaging of different pathologies such as skeletal diseases, thyroid cancer, neuroendocrine cancer, parathyroid adenoma, and mapping of sentinel lymph node in the head and neck and in the pelvic region (Bockisch et al. 2009).
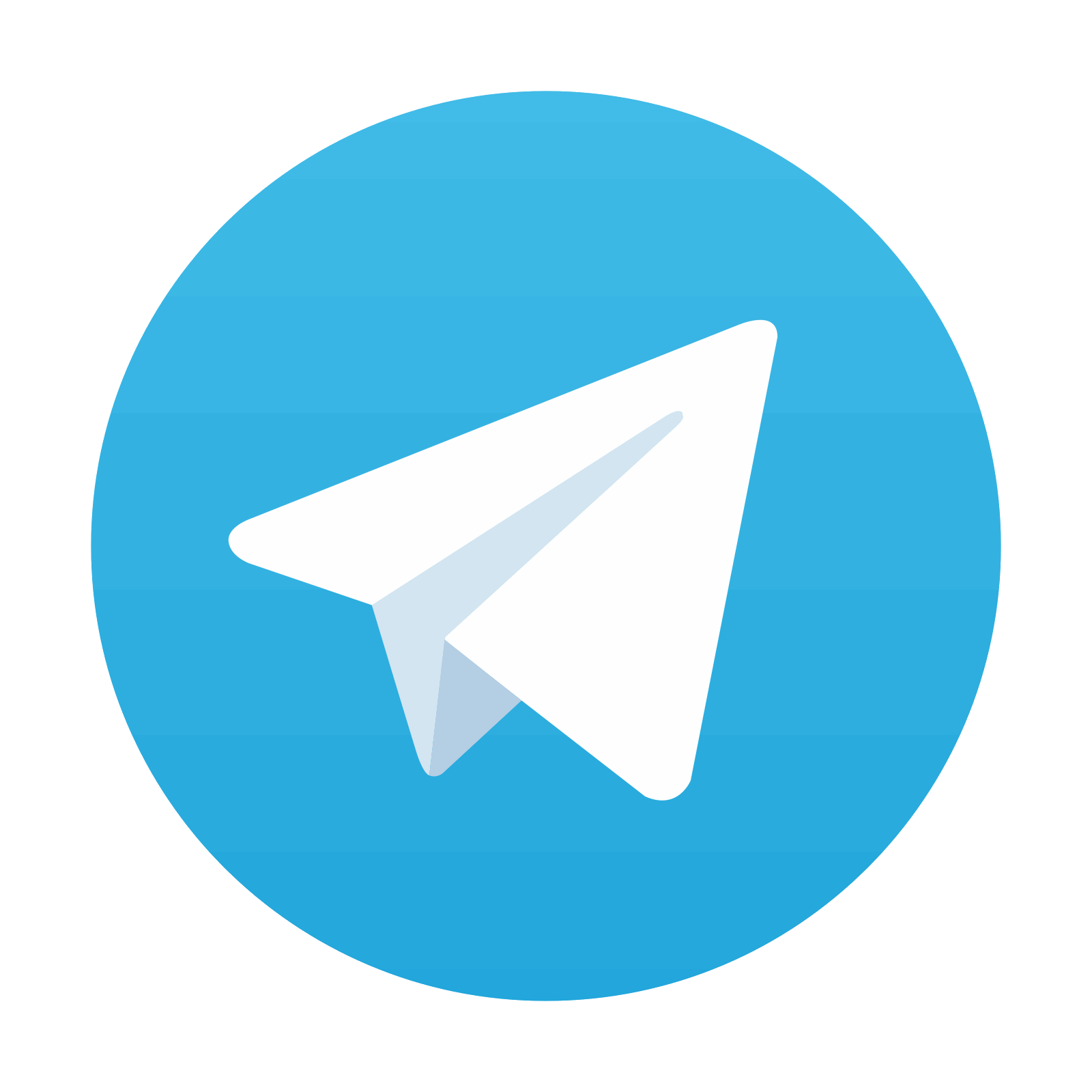
Stay updated, free articles. Join our Telegram channel

Full access? Get Clinical Tree
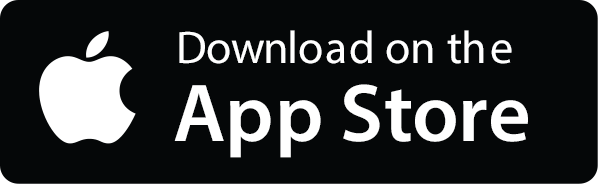
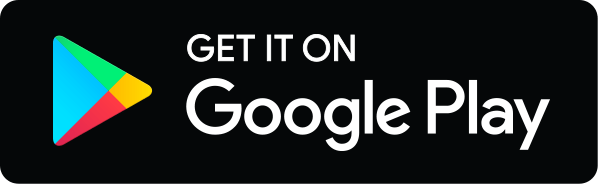