Fig. 15.1
Number of performed or ongoing oncological and non-oncological registered clinical trials from 01/2007 to 06/2010 using contrast media, (a) to investigate the CM itself or (b) to diagnose/monitor disease (Source: clinicaltrials.gov; search terms: contrast media)
At www.clinicaltrials.gov, analysis of registered trials shows the clinical importance of contrast-enhanced imaging with MRI being the most frequently used methodology in comparison to CT and US (Fig. 15.2). The number of registered clinical contrast media trials is highest in North America between 2007 and 2010 (Fig. 15.3).
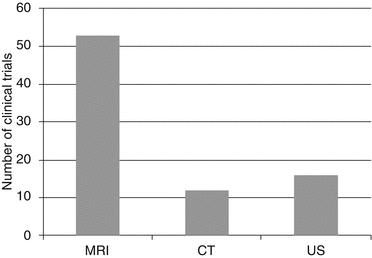
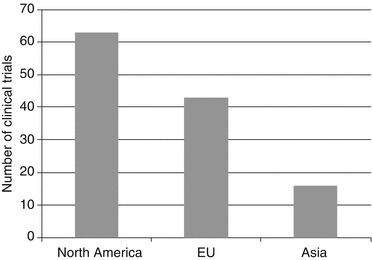
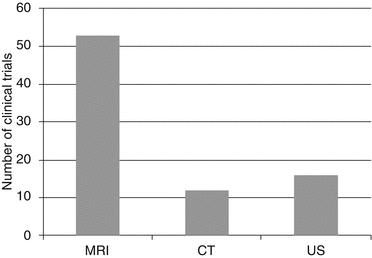
Fig. 15.2
Number of registered clinical trials using contrast media, which are, e.g., compared with existing CM or with native scans in order to investigate the CM itself (Source: clinicaltrials.gov; search terms: contrast media; from 01/2007 to 06/2010)
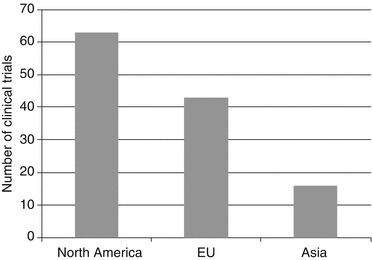
Fig. 15.3
Number of registered clinical trials from 01/2007 to 06/2010 using contrast media sort by region; source: clinicaltrials.gov; search terms: contrast media; (a) to investigate the CM itself or (b) to diagnose/monitor disease (Source: clinicaltrials.gov)
This chapter briefly presents different classes of MR, CT, and US contrast agents available on the market, some with limited labeled indication and therefore still involved in clinical trials to achieve further labeled indications. Mechanisms of action and functionality of various contrast agents will also be reviewed in some detail. The described advantages and drawbacks of different contrast agents allow a better insight into the clinically approved indications and off-label usage. The chapter does not address safety issues of the various contrast media. Finally, we will outline some future scenarios of selected contrast agents in the field of radiology.
Groups of Contrast Agents
Contrast agents are used to enhance image contrast between pathological or anatomical structures of interest and their surrounding tissue or liquid. Intravenously (IV)-injected CM behave differently. First-generation MR contrast agents distribute within the intravascular space and due to its small molecule size diffuse into the interstitial space of extracellular fluid (ECF) and according to the law of equilibrium back into the intravascular compartment. These are often called “unspecific agents” and allow the evaluation of physiological parameters, such as the status or existence of the blood/brain barrier or the renal function. For this class of agents (ECF compounds), there is no intracellular uptake described. CM with intracellular uptake provide tissue-specific characteristics, e.g., they are taken up by lymphatic cells or hepatocytes, e.g., Gd-EOB-DTPA. Some MR contrast media have characteristics of two classes, e.g., the majority of the molecules take an extracellular path being excreted via the kidney and the minor amount presents an intracellular pathway, e.g., liver-specific metallochelates are partially excreted via the biliary system [6–8].
Iodinated Contrast Media
Negative contrast agents are those which yield a lower X-ray attenuation than the body tissue and hence appear hypodense, e.g., gases such as air or CO2. Positive contrast agents yield higher attenuation in comparison to adjacent structures. The positive contrast agents can be further divided into different groups. Iodine and barium are heavy elements and are comparatively less harmful to body giving excellent contrast. A positive IV or IA contrast agent must have a high concentration of iodine atoms with a comparable osmolality to blood. Since their introduction in the 1950s, organic radiographic iodinated contrast media (ICM) have been among the most commonly prescribed drugs in the history of modern medicine. The phenomenon of present-day radiologic imaging would be lacking without these agents.
Over the years quite a number of different iodinated contrast agents have been developed and are distributed by different manufactures. All agents consist of iodinated benzene ring derivatives. All ionic agents are typically formulated as sodium and/or meglumine salts and can be classified as high-osmolar contrast media (HOCMs, “ionics”) and low-osmolar contrast media (LOCMs, “nonionics” and “ionics”) (Appendix 15.1 at the end of this chapter).
ICM generally have a good safety record. In this context, nonionic ICM are generally safer than ionic CM, with less idiosyncratic (non-dose-dependent, e.g., allergy-like) adverse reactions [9] and are better tolerated if extravasation occurs; therefore, they are used almost exclusively today in clinical routine and trials.
Osmolality
Normal human reference range of osmolality in plasma is about 280–300 milliosmoles per kilogram. The ionic iodinated contrast agents contain three iodine atoms (in a monomer unit) and are having higher osmolality than blood. This means that each ionic monomer will dissociate into two particles due their ionicity, so the ratio is 2:3. These agents belong to the group of high-osmolality contrast medium, short HOCM. Then there are ionic dimers containing 6 iodine atoms (ratio 1:3) and having less osmolality than HOCM. Nonionic iodinated monomers (ratio 1:3) are also of less osmolar than ionic because of fewer particle number in water, i.e., nonionic agents do not require an accompanying cation and therefore have lower osmolality and belong to the group of low-osmolar CM, short LOCM [10]. The osmolality depends also on the iodine concentration, which typically ranges from 300 mg I/mL (=670 mOsm/kg H2O) to 370 mg I/mL (=800 mOsm/kg H2O) (Appendix 15.1). In the end there are nonionic dimers containing 6 iodine atoms each molecule (ratio 1:6). An iso-osmolar contrast medium (IOCM) is considered to have least toxicity [9].
Iodine Content
For nonionic agents, the iodine (I) content is easy to determine because it is written on the label. For example, Omnipaque® 300 (GE Healthcare, Princeton, NJ, USA) contains 300 mg I/mL of solution. Vascular enhancement is proportional to the number of iodine molecules administered per unit of time [11]. Besides increasing the injection flow rate, the iodine concentration of the ICM can be adapted to the clinical need. If an iodine administration rate of, e.g., 1.5 g/s is desired, the injection rate needs to be 5 mL/s with a “standard” concentration (300 mg I/mL) compared with an injection rate of 4.3 mL/s using a higher iodinated compound (350 mg I/mL). The highest available concentration today is 400 mg I/mL reducing the flow rate to 3.8 mL/s in the given example. Due to their higher viscosity (Appendix 15.1), adequate cannula size and warming up the ICM to body temperature are highly recommended [12]. The maximum iodine concentration currently available in the USA is 370 mg I/mL.
Meglumine Versus Sodium Salts
All HOCMs are ionic. They are organic acids consisting of an anion (radiodense iodinated benzoic acid derivative) and cation (sodium or meglumine). Sodium salts result in better renal opacification than meglumine salts. Therefore, e.g., sodium salts are used in diatrizoate meglumine (Urovist®, Bayer HealthCare, Montville, NJ, USA).
Nonionic LOCMs
Nonionic monomers (LOCMs) are currently the IV contrast media of choice. They have a lower incidence of adverse reactions (by a factor of 6 for all reactions and a factor of 9 for severe reactions) due to their lower osmolalities and potentially less chemotoxic than the ionic monomers [9]. Nonionic LOCMs are equally effective as contrast agents compared to HOCMs, but they are much higher in cost. The main nonionic agents in the market are listed in Appendix 15.1. Common nonionic monomers are iohexol (Omnipaque®; GE Healthcare, Princeton, NJ, USA), iomeprol (Imeron®; Bracco, Princeton, NJ, USA), ioversol (Optiray®; Covidien, Mansfield, MA, USA), and iopromide (Ultravist®; Bayer HealthCare, Montville, NJ, USA) [15].
MR Contrast Media
There are four types of magnetic properties which MR contrast media fall into: paramagnetism, which is true for Gd and Mn agents; superparamagnetism produced by iron oxides; diamagnetism, which is generally not used for IV contrast agents; and ferromagnetism, also not used as IV contrast agents [16] (Fig. 15.4). Unlike CT contrast media, the effect of MRI contrast agents is indirectly visualized via changes in nearby tissue proton behavior or magnetic susceptibility.
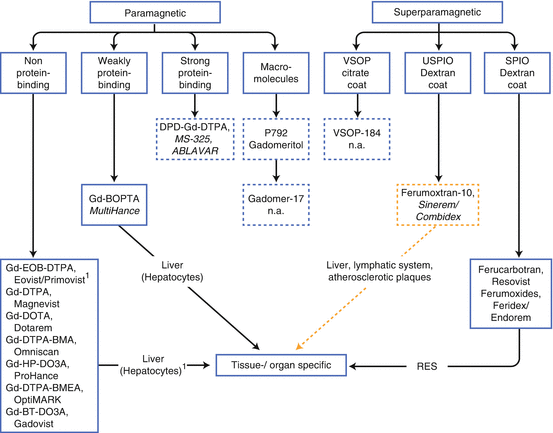
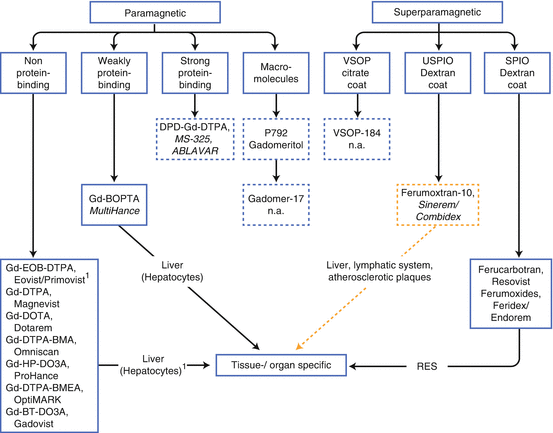
Fig. 15.4
Approved MR contrast media (CM) for intravenous administration. Development/marketing of SineremTM/CombidexTM and Feridex® are discontinued; macromolecules and very small iron oxide particles are/were only in preclinical and clinical trials (phase II): dotted framed boxes. Most of small-molecular CM without any protein-binding capacity are nonspecific except Gd-EOB-DTPA. CM with prolonged intravascular half-life are framed in blue dotted-line boxes. SPIO/USPIO = small and ultrasmall particles of iron oxides. RES reticuloendothelial system
Contrast media reduce the T1, T2, and T2* relaxation times of the tissue surrounding the protons, thus producing a contrast enhancement compared to the surrounding tissue. MR contrast agents have different potencies in shortening relaxation times, which is expressed as relaxivity. According to the T1 and T2 relaxation times, there are two relaxivity constants, r1 and r2. The ratio between r1 and r2 determines if the contrast agent is more suitable for T1 or T2. A CM with a positive r1/r2 ratio is more suitable for T1-weithted imaging and with a positive r2/r1 ratio more effective for T2-weithted imaging [17]. In general, T1 agents lead to a signal enhancement and T2 to a signal loss.
Relaxivities of the classical extracellular fluid (ECF) agents (all ≤1,000 Da, e.g., pioneer Gd-DTPA) show rather little field dependency, whereas the slow tumbling compounds, e.g., MS-325 (non-covalent bound to plasma albumin) and gadomer or P972 (both macromolecules), as well as the iron oxide particles show strong field dependence [18] (Appendix 15.2 at the end of this chapter). Contrast media like Gd-BOPTA with an albumin binding of about 10 % present relaxivities intermediate between albumin-bound MS-325 and ECF agents. Electronic and nuclear relaxation are described in more detail in various books and reviews [16, 18].
According to their magnetic behavior, approved MR contrast media can be divided into paramagnetic and superparamagnetic agents (Fig. 15.4). They are also classified according to their biodistribution: ECF, organ, or tissue specific as well as blood pool (intravascular). Targeting the liver cells, such as the hepatocytes and macrophages of the reticuloendothelial system (RES), is an effective approach for liver-specific agents (Fig. 15.5). Both the paramagnetic and the superparamagnetic agents target well-defined receptors or transporters that are uniquely expressed on the plasma membrane of specific liver cells [19], actually the first group of clinical available “molecular imaging agents.”
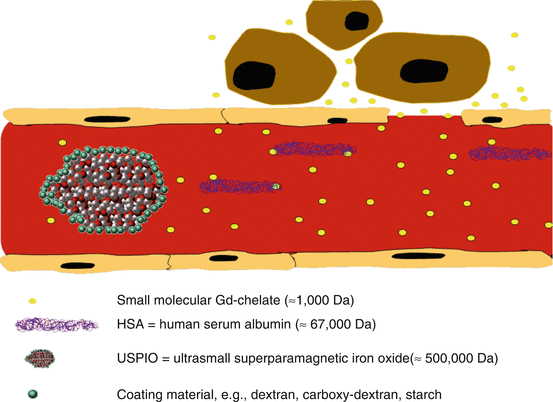
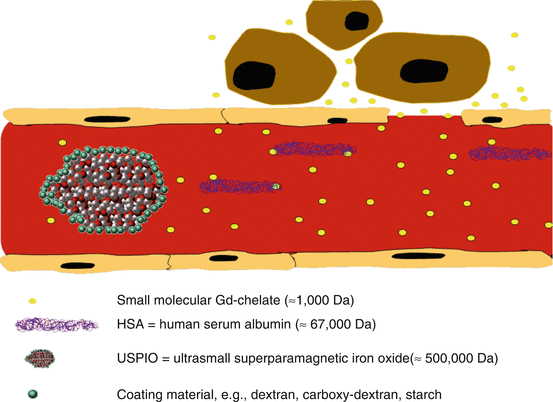
Fig. 15.5
Schematic healthy human capillary with pore size of 6–8 nm in diameter shows extravasation of extracellular fluid (ECF) agents like Gd-DTPA, whereas Gd-based molecules attached to HSA (e.g., Gd-BOPTA) remain intravascular. Also USPIOs (e.g., ferumoxtran-10) stay initially intravascular but leave the compartment by nonspecific vesicular transport or transendothelial channels
Appendix 15.3 (at the end of this chapter) shows an overview of brand names with the physicochemical properties of the currently available MRCM with their application areas. For educational purposes non-approved contrast media gadomer-17, gadomeritol (both macromolecules), ferumoxtran-10, as well as VSOP are listed (Fig. 15.4). All marketed MR contrast media can be considered as safe drugs [20] following adequate patient selection with regard to NSF. The Gd-based agents are safe to use up to dose of 0.3 mmol/kg body weight.
Gadopentetate dimeglumine was the first MR contrast agent available but now several new agents with, e.g., higher relaxivity are available on the market with different imaging properties [21, 22]. The currently available agents are classified as shown in the next section of this chapter.
Type of Contrast Media
Paramagnetic Contrast Media
0.5 M contrast agents with paramagnetic properties were the first to be introduced for clinical use. The first MR contrast agent, gadopentetate dimeglumine (Gd-DTPA; Magnevist®, Bayer HealthCare, Montville, NJ, USA), entered clinical trials of MRI brain studies [23, 24] and was initially marketed in parts of Europe and Asia in 1998 and later on in the USA. Additional six Gd-based contrast agents (GBCA) were developed and are now routinely used in many countries. The ECF MRCM currently approved in Europe for the diagnosis of, e.g., CNS diseases and in regular use are Gd-DTPA, Gd-HP-DO3A (gadoteridol; ProHance®, Bracco, Princeton, NJ, USA), Gd-DTPA-BMA (gadodiamide; Omniscan®, GE Healthcare, Princeton, NJ, USA), Gd-DOTA (gadoterate meglumine; Dotarem®, Guerbet, Villepinte, France), Gd-BT-DO3A (gadobutrol; Gadovist®, Bayer HealthCare, Montville, NJ, USA), and Gd-DTPA-BMEA (gadoversetamide; OptiMARK®, Covidien, Mansfield, MA, USA). All of them are paramagnetic; i.e., they gain magnetic properties in a strong magnetic field reducing the T1 and T2 relaxation times of nearby water protons [17]. Using a T1-weighted MR sequence, these agents increase signal-to-noise ratio (SNR) of perfused tissue and improve contrast-to-noise ratio (CNR). They are so-called positive enhancers. These Gd-chelates do not show any cellular uptake or any characteristics to bind serum proteins. Excretion is predominantly renal and 1 % or less via the hepatobiliary system [25]. Usually after intravenous bolus injection or infusion, GBCA do not cross the blood/brain barrier (BBB), but in the event of BBB disruption, increased extravasation of contrast medium into the CNS can occur quite rapidly [26].
The only paramagnetic non-Gd-based CM is Mn-DPDP (mangafodipir; Teslascan®, GE Healthcare, Princeton, NJ, USA) with a Mn2+ as a central moiety. It offers tissue-specific characteristics since peak liver enhancement occurs in ca. 15 min after injection and persists for several hours. Since manganese is a positive relaxation enhancer, a very small amount (5 μmol/kg) significantly enhances the contrast between healthy liver parenchyma and focal liver lesions. Due to safety reasons Mn-DPDP had to be slowely infused i.v., ie. high-contrasted first pass imaging was not labelled. Due to safety concerns Teslascan has been effectively withdrawn from the European and US market in 2012 (EMA/486286/2012).
Small-Molecular Contrast Media with Higher Molarity
The first Gd-chelates were all produced in the concentration of 0.5 M, which is considered as the standard. However, a compound with a higher concentration of 1.0 mol/L is now available in the market as gadobutrol (Gd-BT-DO3A; Gadovist®, Bayer HealthCare, Montville, NJ, USA) [27]. The size of this compound is comparable with that of conventional GBCA. Its in vitro relaxivity (r1) has been shown to be higher (approximately 20–25 % in plasma at 1.5 T) when compared with other non-protein-binding Gd-chelates [28]. The doubled concentration of this agent reduces the bolus volume by 50 %, which can be preferential for, e.g., neuroimaging depending upon the technique being used [29]. Imaging techniques such as T2* perfusion seem to benefit from this high-molar agent [30].
Small-Molecular Contrast Media with Weak Protein Affinity
Studies by Cavagna and colleagues [31] showed that Gd-BOPTA (gadobenate dimeglumine, MultiHance®, Bracco, Princeton, NJ, USA) leads to a stronger T1 shortening because of its weak affinity for human serum albumin (HSA) (Fig. 15.6) (Appendix 15.2). HSA (13 nm in diameter, 67,000 Da) serves as a macromolecule carrier for Gd-BOPTA demonstrating a stronger enhancing effect at equivalent dose and serum concentration to conventional extracellular contrast agents. This has been demonstrated in dedicated MR studies of the liver [32, 33], brain tumors [34], and the vascular system [35–37]. Compared to conventional ECF-CM, Gd-BOPTA allows a reduction in dose for vascular imaging that might be relevant for the discussion in the context of NSF [38]. Since it was proved in humans that approximately 0.6–6 % of the injected compound is excreted via hepatocytes into the hepatobiliary system [39], multiple studies on liver imaging using Gd-BOPTA have been published. Planchamp and colleagues showed that gadobenate dimeglumine accumulates in mildly cirrhotic rat liver, while, in severe disease, the expression of organic anion transporting peptides (OATPs) is so low that gadobenate dimeglumine behaves as an extracellular contrast [40]. Moreover, gadobenate dimeglumine remains trapped within hepatocytes in rats lacking the MRP2 transporter [41]. A dose of 50–100 μmol/kg of Gd-BOPTA produces significant liver enhancement in delayed imaging scans which peaks around 40–120 min after IV administration.
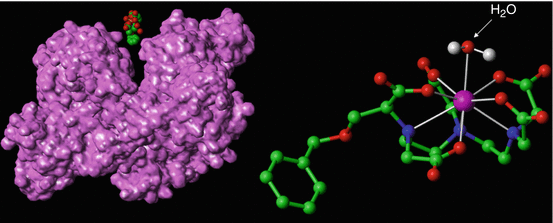
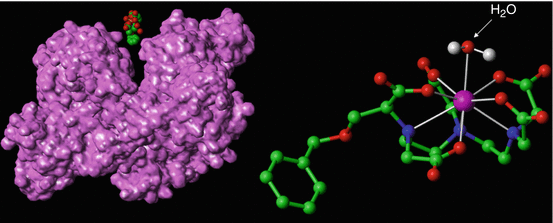
Fig. 15.6
Gadobenate dimeglumine [Gd-BOPTA]2− as a 3D model (right). The molecular structure is similar to that of Gd-DTPA with the exception of the extra benxiloxy-methyl group protruding from the molecule. This lipophilic structure leads to the reversible human serum albumin interaction, HSA (left), as well as its partial hepatobiliary excretion. The H2O molecule (white arrow) binds temporarily to the contrast molecule. The serum albumin shows the surface structure which is accessible to water. Notice the different binding portions of HSA which are potentially available for certain contrast molecules. Pink ball = Gd3+
The protein-binding effect depends on the field strength [28] as well as on the amount of HSA, as proved in vitro by Giesel and colleagues [42]. The relaxivity of Gd-BOPTA measured in serum at 0.5 T is approximately twice that of currently available other gadolinium agents [43]. A docking study demonstrated potentially ten small hydrophobic pockets on the HSA surface where aromatic chains of gadobenate dimeglumine can attach for protein interaction and a strong non-covalent bonding can occur [42].
Recently, the small-molecular Gd-BOPTA has become available for contrast-enhanced CNS imaging. This agent was initially designed for advanced liver imaging, but later it has been proved to be useful for CNS imaging as well [34, 44].
Gadoxetic acid disodium (Gd-EOB-DTPA, Primovist®/Eovist®, Bayer HealthCare, Montville, NJ, USA) is now available in the European and US market and is proved to have a weak (non-covalent) HSA-binding capacity [7]. Primovist is marketed as 0.25 mol/L and labeled exclusively for liver imaging. Due to its lipophilic ethoxybenzyl (EOB) group added, about 40–50 % is taken up by hepatocytes and excreted via the hepatobiliary system [45], the rest via the renal system. In healthy livers, following extracellular distribution, gadoxetic acid enters into hepatocytes through the organic anion transporting peptides (OATPs) located at the basolateral membrane and exits to bile through the canalicular transporter multidrug resistance associated protein 2 (MRP2). The two types of transporters are regulated differently [46]. Because of its hepatocellular accumulation and high relaxivity, a dose of only 10–25 mmol/kg was sufficient to selectively enhance the signal intensity of healthy liver parenchyma (starting at ~10 min postinjection) [19]. As described by Rohrer and colleagues, the effect of increased r1 due to HSA decreases with increasing field strength [28] (Appendix 15.2).
In summary, it needs to be emphasized that both agents, Gd-BOPTA and Gd-EOB-DTPA, show characteristics of an intracellular contrast agent due to uptake by hepatocytes. Therefore, they do not belong to the traditional group of “simple” ECF agents. Both agents belong to the group of liver-specific CM (LSCM) and are increasingly being used in clinical routine improving detection and characterization of liver masses during early dynamic perfusion phases and late hepatocyte phase in the cirrhotic and non-cirrhotic liver [47]. Gadoxetic acid-enhanced magnetic resonance (MR) imaging during the late hepatobiliary phase helps to characterize hepatocellular carcinomas (HCCs).
Small-Molecular Contrast Media with Stronger Protein Affinity
Recently MS-325 (gadophostriamine trisodium; ABLAVAR®, formerly Vasovist®, Lantheus Medical Imaging, N. Billerica, MA, USA) became available in selected markets globally. This compound is also a relatively small Gd-chelate, but has a much stronger affinity for HSA than Gd-BOPTA and Gd-EOB-DOTA [48]. The majority of the injected compound binds to HSA non-covalently [49]; depending on the administered dose and type of serum albumin (human or animal), the unbound fraction behaves as the a conventional ECF compound [25]. This “hermaphrodite” is defined as belonging to the group of intravascular contrast agents in spite of the fact that unbound fraction of this compound extravasates into the nonvascular space like any other small-molecular contrast agent. This strong binding effect can be explained by the so-called receptor-induced magnetization enhancement strategy [25]. The binding to HSA causes an increase in relaxivity of the molecule, an approximately ninefold increase at 20 MHz and four- to fivefold increase at 64 MHz (Appendix 15.2) [18, 28]. First published results in regard to this compound are for body contrast-enhanced MRA [50]. ABLAVAR® is currently only approved for peripheral vascular imaging (abdominal aorta and runoffs). Excretion of the compound is mainly via kidney and urinary system. Clinical studies need to prove how CNS imaging may benefit from its special pharmacokinetics and enhancement characteristics [51].
B-22956 is also an SMCM with a stronger affinity for HSA and hence exhibiting an increase T1 relaxivity (stronger binding with a bound fraction of HSA of approximately 94 %) as compared to MS-325 with an excretion pathway via the hepatobiliary (~45 %) and urinary system (~55 %) [52] but RND (phases II and III) has been discontinued after evaluation for coronary artery imaging.
Superparamagnetic Contrast Media
Historically, parental iron oxide particles have been used as contrast agents for a long time [53]. These colloid-based nanoparticles are produced with a core size of 50–180 nm as superparamagnetic or small particle of iron oxide (SPIO), with a core size of 10–50 nm as ultrasmall SPIO (USPIO) or even less than 10 nm as very small superparamagnetic iron oxide particle (VSOP) contrast agents. The core of the iron oxide colloids is composed of multiple 2–8 nm-sized iron oxide monocrystals and coated by, e.g., dextran or starch. Theses coated iron particles have a molecular weight of approx. 700,000 Da, similar to the endogenous ferritin with a diameter of approx. 11 nm (400,000–600,000 Da).
SPIOs present a high r2 during a short blood half-life time (within minutes). T2 relaxivities of >150 L/mmol/s are common with liver-specific SPIOs [19] (Appendix 15.2). Offering a stronger magnetic moment with a higher r2, they belong to the group of “negative enhancers” because in a stronger magnetic field, they predominantly cause strong susceptibility effects [54]. The apparent (hydrodynamic) size of the SPIO in blood is larger than the core because of hydration of the particle. The exact size of SPIO in blood is not known, probably in the order of 50–200 nm.
USPIOs present also a high r1 relaxivity offering a longer blood half-life (hours). Biodistribution and superparamagnetic effect varies strongly with size, electrical charge, and surface coating [55, 56].
In clinical routine there is not enough usage of this type of CM. Therefore, companies had to scale down or stop its production. However, in preclinical imaging of stem-cell tracking, many research groups are using (U)SPIO-based agents as described in more detail next.
SPIO
Depending on size and coating, SPIOs show specific uptake by the reticuloendothelial system (RES) of liver (~80 % of the injected dose) and spleen (6–10 % of the injected dose) within minutes after administration [57, 58]. Just 3 mg Fe/g liver will cause a signal decrease of 50 % in a standard T2-weighted sequence [19]. They have been available in the market as ferumoxides (Feridex®/Endorem®, Berlex, Guerbet), for example; however, they are only labeled for liver imaging [59] (Appendix 15.2). Ferumoxides were approved by the U.S. FDA in 1996 and is sold in the USA under the name Feridex®. It was originally developed as a liver contrast agent because it is taken up by Kupffer cells but did not live up to its promise and has been taken off the market because of lack of sales in November 2008. In Europe ferumoxides are still available under the name Endorem®. Ferucarbotran (Resovist®, Bayer HealthCare, Montville, NJ, USA) is the second available (Europe and Asia) SPIO, also coated with dextran with similar effects: using T2-weighted imaging techniques, a dose of ~8–15 μmol Fe/kg produced significant results in clinical studies.
The iron is handled by the usual metabolic pathways of the body after biodegradation of the compound. Once sequestered by phagocytic cells, the agent decreases liver and spleen signal intensity T2w (liver signal intensity is maximally decreased 0.5–6 h after administration and returns to normal within ~7 days). Malignant tumors are typically devoid of large number of phagocytic cells so that they appear as relatively hyperintense (“bright”) lesions contrasted against hypointense (“black”) background of normal liver parenchyma. Tumors with phagocytic elements (e.g., FNH) and hemangioma have been shown to also decrease in signal intensity [60].
USPIO
These agents have a blood half-life of over 20 h and thereafter accumulate in macrophages, e.g., lymph nodes, liver, and spleen (SPIOs, on the contrary, are too rapidly cleared by liver to be able to accumulate in lymph nodes). Because this group of agents has a significantly higher r1 compared to SPIO, it can also be used as T1-type blood-pool agents for imaging of tumor angiogenesis, atherosclerosis, and/or hepatic lesion characterization during the equilibrium phase [61, 62]. Perfused lesions (e.g., hemangioma) increase in signal intense on T12, whereas the same lesion decreases in signal intensity on T2w. Ferumoxtran-10 was in clinical trials (phase III) for lymph node imaging. The particles are phagocytosed by the macrophages of the lymphatic system [19].
The inhomogeneous distribution within nonmalignant lymph nodes increases their susceptibility effect (T2*) and leads to a reduction of the signal intensity. Unfortunately, it was not given market approval; therefore, no further clinical trials will be performed with support of the producer.
Ultrasmall and very small superparamagnetic particles of iron oxide have also been used for T1-weighted CE-MRA; however, for NC100150, clinical trials have been stopped [63, 64]. VSOP (very small superparamagnetic iron oxide particles) are a new class of contrast agents with smaller particle size than SPIO offering advantages for MR angiography. They have been tested in a phase I study [65]. SPIO particles are usually coated with an organic polymer such as dextran, carboxydextran, or polyethylene glycol, which limits the minimal overall particle size that can be obtained. VSOP-C184 consists of an aqueous solution of superparamagnetic iron oxide particles with a citrate coating with an overall particle size of only 4–8 nm.
In preclinical and clinical trials as well as in the clinical routine (as “off-label” use), USPIOs have been successfully also used for CNS imaging. In clinical studies, improved differentiation and characterization of the brain tumors using iron oxides have been shown as promising CNS applications [66].
Macromolecules
Macromolecular CM belong to the group of paramagnetic compounds. They are being tested worldwide (Weinmann, Corot, Choyke). Preclinical data show that medium-sized polymeric gadolinium complexes demonstrate great promise for improving MR angiography and in quantificating capillary permeability and myocardial perfusion. One of the most advanced compounds is gadomer-17 which carries 24 gadolinium atoms with a numeric molecular weight of 17.5 kDa (Appendix 15.2 and Fig. 15.4). However, due to the large hydration radius of the molecule, the apparent molecular weight is 35 kDa. Gadomer-17 is small enough to guarantee glomerular filtration and large enough to ensure a slow diffusion through the bigger pores of the endothelial wall. The agent is eliminated by glomerular filtration within 24 h in an unmetabolized form [19].
P-792 (Vistarem®, Guerbet, Villepinte, France) is a Gd-based macromolecule, which is a hybrid between small agents and polymeric compounds (Fig. 15.7). The agent has a molecular weight of 5 kDa and exhibits some self-assembling characteristics, resulting in a larger molecular volume [67]. The concentration of the aqueous formulation is 35 mmol Gd/L, considerably lower than the other blood-pool agents, which have a 250 or 500 mmol/L strength and a very high relaxivity of 25 L/mmol/s at 1.5 T (Appendix 15.2). P-792 has a short half-life, but it distributes strictly in the vascular space during the first minutes after dosing [68]. The compound is cleared primarily by renal filtration and, to a minor extent, by hepatobiliary excretion.
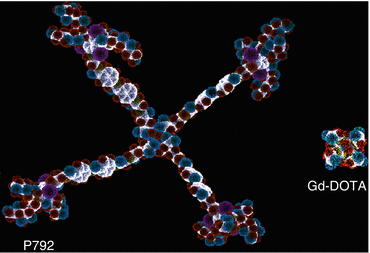
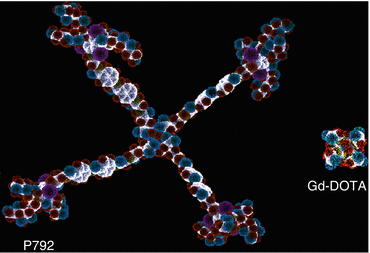
Fig. 15.7
Macromolecular compound P792 in comparison with Gd-DOTA. P792 itself has a macrocyclic Gd-DOTA molecule in its center. Both of them are paramagnetic contrast agents but with different diameters by molecular modelization, P792 = 29 nm and Gd-DOTA = 0.9 nm (Courtesy of Guerbet Research Group)
Ventilation Agents
For ventilation perfluorinated agents, Gd-based aerosols as well as hyperpolarized gases (3He and 129He) can be used as well as oxygen [69].
Molecular or Cellular Imaging Agents
Magnetic resonance imaging (MRI) is a powerful imaging modality that can provide an assessment of function or molecular expression in tandem with anatomic detail. Over the last 20–25 years, a number of gadolinium-based MR contrast agents have been developed to enhance signal by altering proton relaxation properties.
Gd-DTPA was the first approved MRI contrast agent and is the most widely used compound [70]. It is clinically safe and it seems logical to use it off label for clinical MRI cell tracking.
Unfortunately, there are some pitfalls with this famous compound; i.e., labeling cells with Gd-DTPA would result in long-term retention in the body with an uncertain clearing mechanism, i.e., it may not be renal. Although the pH of blood plasma is nearly neutral, sufficient stability of the chelate complex for its current clinical applications is challenged. The low pH in lysosomes and endosomes of cells may lead to rapid de-chelation once cells are labeled with a paramagnetic agent. There is potential toxicity concern about the existence of free Gd3+ ions leading to nephrogenic systemic fibrosis [71].
A further reason for not labeling cells with Gd-DTPA is that on the basis of the inherent physical principles of MR relaxation, intracellular Gd-DTPA has much reduced T1 relaxivity owing to differential water exchange and inner sphere relaxation [16]. Further challenges occur due to compartmentalization leading to local magnetic susceptibility effects that can produce ambiguous (e.g., positive vs. negative) contrast enhancement, particularly at higher field strengths.
SPIO and USPIO are a different class of MR contrast agent that leads to hypointense contrast enhancement of the cells of interest after proper labeling [72]. Ferumoxides are the only pharmaceutical-grade MR contrast agent that has been used for clinical cell tracking.
Many groups investigate which macromolecular structure might lead to an adequate MR-based labeling compound for clinical usage, which could be composed of albumin, polylysine, polysaccharides (dextran, inulin, starch), polyethylene glycol, copolymers of cystamine and cystine with GD-DTPA, and various dendritic structures based on polyamidoamine and polylysine (gadomers) [73, 74].
Gadofluorine M, Gf, is an amphiphilic Gd complex with a molecular weight of 1,528 g/mol and a concentration of 250 mmol Gd/L [75]. Gf binds to serum albumin at an affinity of kDa = 2 mmol/L. Moreover, Gf reveals a similar binding affinity to the extracellular matrix (ECM) components collagen, proteoglycan, and tenascin [68]. Unlike USPIOs, gadofluorines do not accumulate in the subendothelial monocyte-rich areas, but in deeper regions of the intima that are probably rich in foam cells and cellular debris. Porphyrins and other ring structures target atherosclerotic plaques too [19]. However, its localization of these compounds is not known.
About one-third of the dose is excreted by glomerular filtration, and two-thirds are excreted in the feces. Animal studies in VX2 tumor-bearing rabbits led by Misselwitz et al. showed that Gf accumulated in lymph nodes fascilitating the differentiation of metastastic tissue within the nodes. [75]. An in vitro and in vivo cell tracking study by Giesel and colleagues showed that Gf performs as a novel contrast agent with the capability of intracellular accumulation without an uptake mediator providing a T1-positive MRI signal at 1.5 T and may be suitable for cell tracking in animal models with intraparenchymal hemorrhages such as stroke or malignant tumors [76].
Gadofluorine uptake closely corresponded to inflammation and demyelination on tissue sections in a study performed by Bendszus and colleagues [68]. These unique features of gadofluorine M in visualizing inflammatory CNS lesions hold promise for future clinical development in multiple sclerosis.
Outlook
Four distinct future advantages of in vivo contrast agents can be defined: higher concentration, protein interaction, intracellular uptake in vivo, and intravascular characteristics although not exclusively found like ultrasound agents. Future MRI pulse sequence design will also need to take the potential benefits of the specific contrast agent into account for optimized results. The clinicians’ dream of a perfect contrast media that accumulates highly and specifically in malignant tumors, allowing an accurate diagnosis at a stage when the disease is still treatable, is still possible. It is realistic to assume that new specific low-molecular peptides will be coupled to high-relaxivity moieties as a practical approach for moving molecular imaging into clinical MR reality [19].
The high number of ongoing preclinical and clinical trials will lead to the availability of further several different MR contrast agents in the future provided the excellent safety profile of current CM can be met and costs of extensive study designs can be compensated by successful business.
US Contrast Media
In the past, the potential of echo-enhanced US was underestimated. In addition, it took some years to overcome all development problems. All US contrast media have in common is that they are based on microbubbles restricted in their pharmacokinetics to the intravascular space (Appendix 15.4 at the end of this chapter).
Albunex® (Covidien, Mansfield, MA, USA) was the first agent that contained microbubbles coated with sonicated albumin with a mean size of 4 μm (95 % below 10 μm in diameter) with a short plasma half-life time of approx. 1 min [77]. Optison™ (GE Healthcare, Princeton, NJ, USA) (introduced by GE Healthcare in 1998) was then developed as a modification of Albunex, i.e., nearly the same microbubbles but instead of air the gas octafluoropropane was used [78]. The diameters are 3–4.5 μm (max. 32 μm; 95 % less than 10 μm). Echovist (Bayer HealthCare, Montville, NJ, USA) was developed based on galactose-coated microbubbles filled with air and, therefore, missing transpulmonary stability. Its successor Levovist (Bayer HealthCare, Montville, NJ, USA) (introduced by Schering in 1996) is coated in addition with palmitic acid as a surfactant offering better stability, echo increase is described up to 24 dB and duration of echo enhancement approx. 5 min [79]. SonoVue® (Bracco, Princeton, NJ, USA) (introduced by Bracco in 2001) is a relatively new echo-contrast agent that is based on sulfur hexafluoride-filled microbubbles coated with phospholipids with a diameter of ca. 2.5 μm (90 % smaller than 8 μm) [80]. The stability lasts about 6 h after reconstitution, which allows a longer time window. SonoVue is currently being investigated in a number of clinical trials embracing the fields of cardiac as well as macro- and microvascular imaging in different organs and pathologies. It is already established in the diagnostic work-up of focal liver lesions. Perflutren protein type A microspheres (Definity®/Luminity® (Lantheus Medical Imaging, N. Billerica, MA, USA) introduced by Bristol-Myers Squibb in 2006) is based on lipid bilayers coating octafluoropropane with a size of 1.1–3.3 μm (98 % less than 10 μm) with a mean scan window is about 90s [81]. The shell of Imagent® (IMCOR Pharmaceutical, San Diego, CA, USA) (developed by Alliance Pharmaceutical Corporation and Schering AG; FDA approval 2002) is composed by surfactants coating perfluorohexane with a mean bubble diameter of 6 μm. Market approval was given for left ventricle echocardiography.
US Contrast Agents for Liver Imaging
Some US contrast agents have advantages in the liver-specific late phase where bubbles accumulate in normal liver parenchyma beginning approximately 2–5 min after injection when the vascular enhancement has faded, analogous to liver-specific contrast agents used in MRI. Microbubbles known to exhibit this behavior are Levovist and Sonazoid as well as, still under RND, Sonavist (GE Healthcare, Princeton, NJ, USA) and BR14 (Bracco, Princeton, NJ, USA) [82]. The mechanism of hepatic accumulation is not completely understood. Several experimental agents such as Sonazoid or BR14 combine the advantage of good enhancement at low MI with strong liver-specific properties. Uptake by the Kupffer cells of the liver has been demonstrated for Sonavist and Sonazoid. Sonazoid™ (Daiichi Sankyo, Parsippany, NJ, USA) (approved in Japan since 2006) is a lipid-stabilized suspension of perfluorocarbon microbubbles with a median diameter of 2.4–2.5 μm. The product is characterized by a prolonged ability to maintain its contrasting attributes with indication to improve differential diagnosis of hepatic lesions. In clinical trials, perflubutane-enhanced ultrasound was shown to have the same diagnostic ability as computed tomography for tumor detection and characterization of hepatic nodules.
Contrast Mechanism
Echo enhancement is managed by several factors. First of all, these gas bubbles, which are less than 10 μm in diameter, are able to enhance the echo strength (up to 25 dB; greater than 100-fold increase) by their compressibility under acoustic waves leading to impedance between the gas bubbles and the surrounding tissue or fluid. Another factor is that these bubbles are able to oscillate, which leads to a better sound energy absorption and reradiation [83].
But the most important factor for echo enhancement is the so-called nonlinear oscillation of the microbubbles. In higher acoustic powers, the microbubbles will enlarge more than they are compressed thus leading to reradiation, not only of the frequency with which the bubbles were excited but also containing harmonics [83]. SonoVue provides strong and persistent signal enhancement due to its strong harmonic resonance at low (≤0.2) and very low (≤0.1) mechanical index (MI), where minimal or no bubble destruction occurs. Real-time low-MI imaging is a preferred method in many instances, although SonoVue has weaker liver-specific properties than Levovist. Levovist, however, requires high-MI imaging (MI ≥0.7). The disadvantage of high-MI imaging is the highly transient nature of the signals, which persist only for a few frames.
An increase of the acoustic power leads to a destruction of the bubbles. This effect can be used for diagnostic purposes but also for targeted drug therapy.
Outlook
Microbubbles used as blood-pool tracer have also great potential in targeted molecular imaging [84]. This is possible by attaching molecules to the bubbles that are targeted to specific receptor sites. Currently such microbubbles are investigated in the field of angiogenesis, atherosclerosis, and inflammation.
BR14™ is a new experimental ultrasound contrast agent, consisting of bubbles containing a high molecular weight filling gas enclosed by a flexible phospholipid monolayer shell a few nanometers thick. This agent shows significant nonlinear scattering and agent modification even at low insonation pressures, the detection pulses do not destroy the contrast bubbles. The results obtained with harmonic power Doppler (HPD) before the release burst show that the BR14 bubbles are efficient scatterers that can be modified and, thus, detected by low-power insonation. First application targets are myocardial and microcirculatory kinetics [85]. BR55™ (Bracco, Princeton, NJ, USA) is a lipopeptide-based VEGFR2-targeted ultrasound contrast agent for molecular imaging of angiogenesis [86].
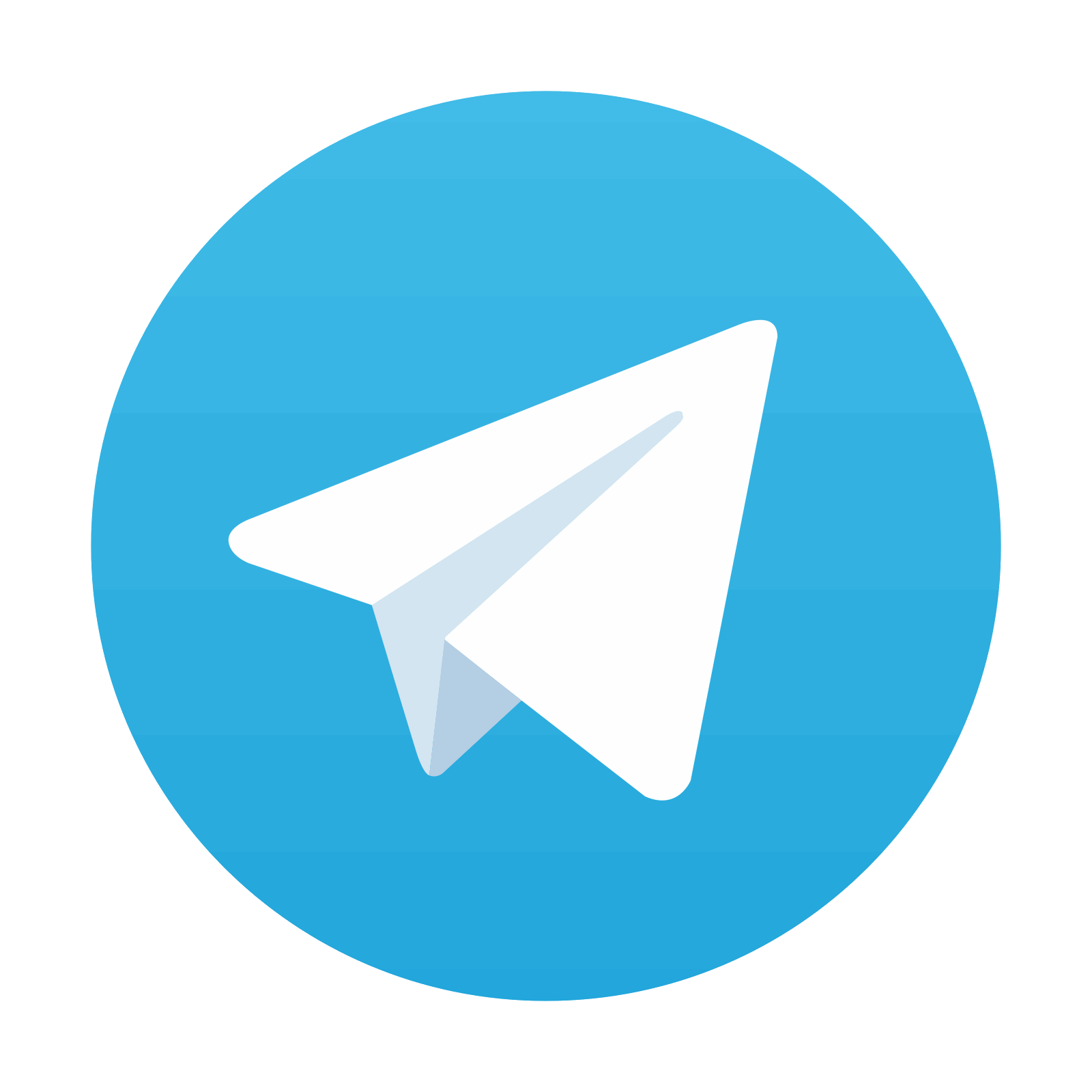
Stay updated, free articles. Join our Telegram channel

Full access? Get Clinical Tree
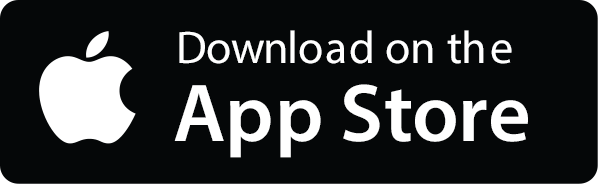
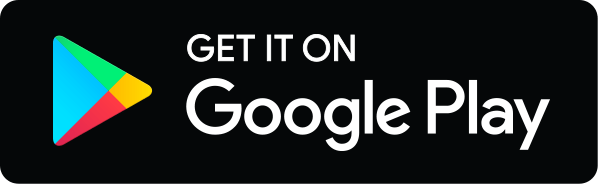