Fig. 6.1
Pressure, resistance, and response to metabolites of epicardial coronary arteries, arterioles, and the microvasculature (From Camici and Crea [2] with permission)
(a)
Resistance arteries (100–400 μm) (pre-arterioles): these are primarily regulated by shear and myogenic forces)
(b)
Arterioles (100 μm) connect the resistance arteries to the microvascular bed, which in turn feeds into the myocardial venous return. Arterioles contribute approximately 25–35 % of total coronary resistance and are primarily regulated by myogenic and local forces; and
(c)
The remainder of coronary R2 resistance is demonstrated in the capillary bed component and coronary venules of the microcirculation [5]. Normally, there is minimal resistance offered by these two components and remain constant with changes in vasomotor tone. They can contribute up to 20 % of the coronary resistance in the presence of maximum coronary vasodilatation. The capillary density is 3,500/mm2 and is greater in the subendocardium that the subepicardium.
3.
Compressive resistance R3. Subendocardial flow primarily occurs in diastole and declines below a mean perfusion pressure of 40 mmHg. Conversely, subepicardial flow occurs throughout systole and diastole and only declines below a pressure of 25 mmHg. Cardiac contraction raises the perivascular pressure to that of LV systolic pressure at the subendocardium layers impeding flow and to subpleural pressures in the subepicardium. This may also occur with elevated LV diastolic pressures that occur with heart failure.
Importantly, factors that may result in elevated baseline flow may result in a decrease in flow reserve [1, 4]. These may be related to factors that:
1.
Increase oxygen consumption, such as heart rate, systolic blood pressure, increased contractility, age, or myocardial hypertrophy.
2.
Reduce arterial oxygen supply, such as anemia and hypoxia will also increase baseline flow.
Finally, vasoactive drugs such as calcium channel blockers may decrease baseline vasomotor tone, resulting in baseline increase in flow. Other contributing factors to CBF include the rheological composition of blood [1, 4].
Hyperemic Coronary Blood Flow
Coronary reserve is the ability of the coronary vascular bed to increased flow in response to stimuli. Such stimuli include reactive hyperemia that results from vessel occlusion, active hyperemia from exercise, or pharmacologic agents. Importantly, autoregulation results in intrinsic vasoconstrictor tone of the arterioles, and neural mechanisms though sympathetic or parasympathetic drive contributes to the overall tone of the coronary system.
Pharmacologic agents such as adenosine, regadenoson, dipyridamole, and papaverine, are utilized to induce hyperemia in the coronary system. This induction of hyperemia results in removal of the intrinsic tone of the arterioles and microvascularute, accentuating the contribution of epicardial stenoses to resistance in the coronary circuit. Impairment of endothelial-dependent vasodilatation, as with dyslipidemia, impairs hyperemic CBF and thus CFR.
Coronary flow reserve is the ratio of maximal hyperemic flow to resting coronary flow – usually a ratio of 2–5 in humans [6, 7]. however, is typically only 2–3 when measured invasively in the cath lab. As epicardial coronary obstruction increases, resistance increases in the coronary vessels resulting in a reduction of coronary flow reserve.
Importantly, stenosis severity first decreases flow across a lesion during maximal hyperemia; only when that reduction in flow falls to below resting levels does resting ischemia ensue [8]. Studies nearly 40 years ago demonstrated the “threshold” that a 70 % stenosis results in reduction in coronary flow, and resultant myocardial ischemia [5]. Additionally, a stenosis greater than 80–90 % result in reductions in resting blood flow [5, 9] (Fig. 6.2). Yet, the simplification of worsening stenosis correlating to reduced coronary blood flow does not take into account the three-dimensional anatomy of the coronary vascular bed, imprecisions in angiographic estimation of coronary stenosis, and lesion length. In addition, the use of coronary stenosis severity as guidance for percutaneous intervention is fraught with pitfalls; including interobserver and intraobserver variability, diffuse disease, and reference segment disease [10, 11].
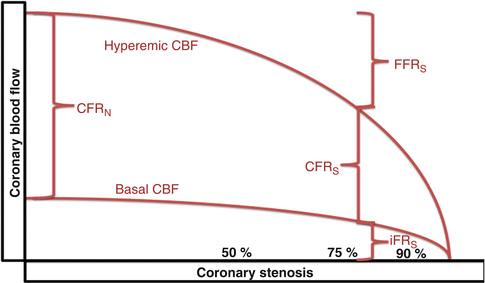
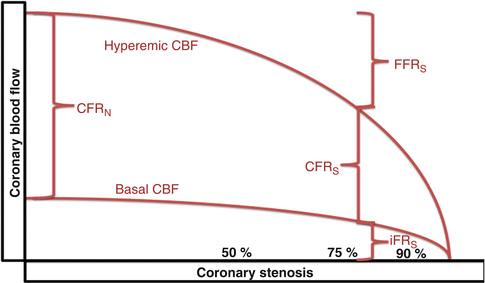
Fig. 6.2
Diagrammatic representation of basal and hyperemic coronary blood flow, fractional flow reserve (FFR), coronary flow reserve (CFR) in normal conditions (CFR N ), CFR in the presence of a stenosis (CFR S ), as well as instantaneous wave free ratio (iFR) in the presence of stenosis
Measures of Resistance and Flow in the Cardiac Catheterization Laboratory
1.
Coronary flow reserve (CFR): CFR assesses both epicardial vessel stenosis and microcirulatory function and is assessed by a velocity wire, thermodilution, combined FFR and CFR wire, and less accurately a pressure wire. Values >3 are non-ischemic, values less than 2 indicate either microcirculatory dysfuntion function and/or severe epicardial vessel stenosis [1, 4]. It is calculated as
Where Qh = hyperemic CBF, Qb = Basal CBF, Vh = hyperemic velocity, Vb = basal velocity
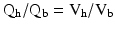
2.
Relative coronary flow reserve (rCFR): It is the basis of non-invasive assessment of ischemia. It can be performed in the cath lab by calculating the ratio of CFR of a stenotic epicardial segment to the CFR in a normal remote epicardial vessel allowing the assessment of epicardial stenosis severity [1, 4].
3.
4.
Hyperemic stenosis resistance (HSR): theoretically assesses epicardial vessel stenosis and requires both a pressure and velocity wire it is the ratio of the hyperemic stenosis pressure gradient to the hyperemic flow (assessed by the hyperemic average peak velocity. Pressure measurements may be obtained by the RADI wire (RADI medical systems, Uppsala, Sweden, and Doppler measurements by the Doppler-tipped guidewire ( as Volcano wire, San Diego, CA). A normal value is 0 and a value ≥0.8 mmHg/cm/s is abnormal [1, 4]. It is calculated as
It was initially touted as more reliable and more comparable to non-invasive SPECT testing than either CFR or FFR especially in the group of CFR/FFR mismatch. However, it is currently reserved to research purposes

5.
Index of microcirculatory resistance (IMR): it assesses the microcirculatory function and is assessed by a pressure wire [1, 4]. It is calculated as
Pd = distal pressure Pv = central venous pressure, Tmnh = hyperemic mean coronary thermodilution transit time.
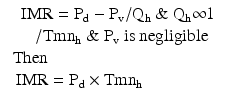
A normal value is 8–25, >25 is abnormal, and a value <32 denotes greater LV recovery after an MI. Usually it is calculated as the mean transit hyperemic time of 3 ml of saline bolus and measured simultaneously with the Pd. It may provide a qualitative assessment of microvascular function at the time of primary PCI and s linked to microvascular obstruction as assessed by contrast enhanced cardiac MRI. The coronary pressure/temperature-sensitive guidewire (RADI Medical Systems, Uppsala, Sweeden is used for obtaining this index.
6.
Hyperemic microvascular resistance (HMR): it assesses the microcirculatory function and is assessed by both a pressure and velocity wire. It is similar to IMR, however, it uses velocity rather than thermodilution as a correlate of flow after NTG administration to maintain vessel cross sectional area [1, 4]. It is calculated as
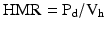
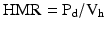
HMR > 1.9 mmHg/cm/s is defined as high and its adequate assessment of actual microvascular resistance in the presence of epicardial stenosis is controversial due to its neglect of collateral flow
7.
Endothelial function assessment: These tests assess the change in coronary artery diameter and Doppler wire velocity with acetylcholine injection. They demonstrate a decline or no change in in epicardial coronary artery diameter with epicardial endothelial dysfunction. However, in the presence of microvascular endothelial dysfunction, there is a decline in CBF [1, 4]. It requires a velocity wire and angiography.
Assessing Coronary Indices of Flow and Resistance: Technique and Performance
CFR measurements have been obtained with the use of a Doppler wire to measure coronary blood flow velocity, thermodilution, combined FFR and CFR wire measurements, and coronary pressure wire stand alone measurements. Since flow = area × velocity, velocity maybe used as a correlate of flow when the cross sectional are of the vessel remains constant. As such, nitroglycerin is used to maintain constant vessel diameter.
Coronary flow reserve is traditionally measured utilizing an angioplasty guidewire with a piezoelectric crystal at the tip to measure Doppler frequencies. The Volcano Flowire® (Fig. 6.3) is currently the only commercially available CFR wire using a piezoelectric crystal, which is available in 0.014 × 175 cm and 0.014 and 300 cm lengths. Coronary flow velocity is calculated utilizing the principle of Doppler shift, using the magnitude of frequency shift to calculate velocity of red blood cells.
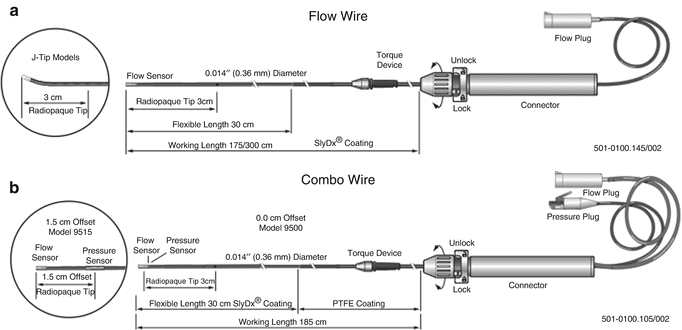
Where V equals velocity of red cells, F1 is the frequency of returning Doppler signals, F0 is the transmitting frequency, c is the speed of sound in blood, and cosθ is the angle of incidence of the Doppler signals to flow of red blood cells (this value presumed to be 1).
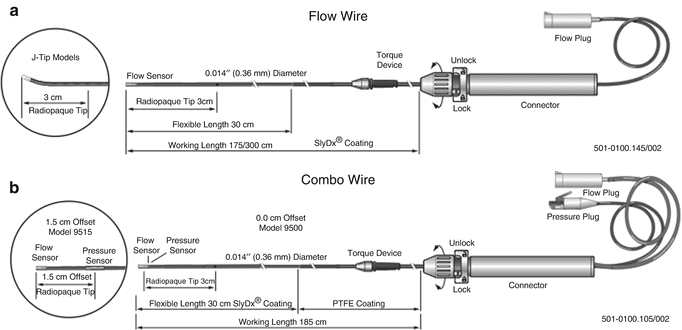
Fig. 6.3
(a) FloWire® Doppler guide wire – measures flow and provides CFR values; (b) ComboWire® pressure and Doppler guide wire – measures both flow and pressure and provides CFR and FFR values (Courtesy of Volcano Corp)
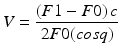
The velocity of red blood cells moving past the ultrasound is then measured at rest, and during peak hyperemia, and the ratio is calculated. Usually, in humans, this ratio is 2–5 [6, 7].
Thermodilution methods with injection of saline at proximal port and measurement of time of transport as measurement of velocity over a known distance have been utilized.
Novel mechanisms have been utilized recently with combined FFR and CFR wire, which has been well validated.
In addition, pressure-derived CFR was calculated by the square root of the pressure gradient across the stenosis during hyperemia divided by the square of the gradient at rest [12–16]. However, the latter assumes that friction losses across a coronary lesion are negligible. This method has been shown to systemically underestimate CFR values suggesting that friction loss is indeed an important determinant of pressure gradient along a coronary artery stenosis. Different agents that are used for hyperemia are include adenosine either intravenous (140 mcg/kg/min) or intracoronary (30–60 mcg), intracoronary papaverine (10–15 mg), and intracoronary nitroprusside (50–100 mcg). The fastest acting and shortest duration is intracoronary adenosine. All these agents can cause hypotension, in addition, adensoine can cause AV conduction disturbances, while papaverine can cause torsades [4].
Measurements Obtained
Because of the relative speed and ease at which the measurements can be obtained, obtaining two sequential measurements is recommended for reliable information.
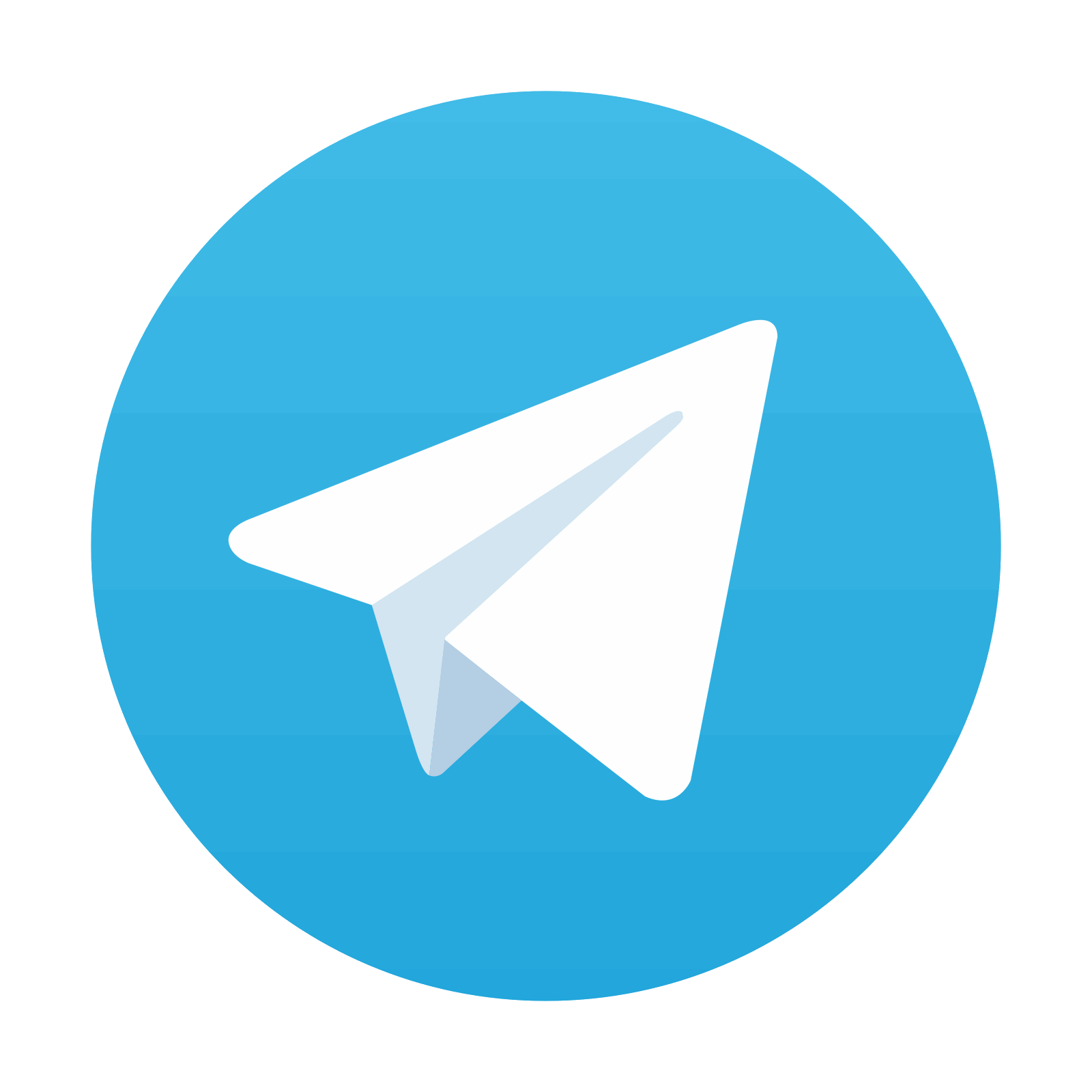
Stay updated, free articles. Join our Telegram channel

Full access? Get Clinical Tree
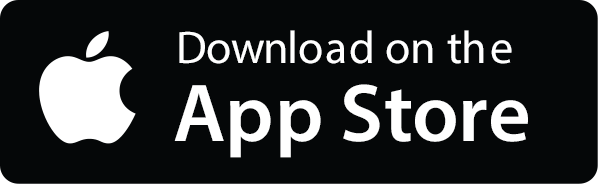
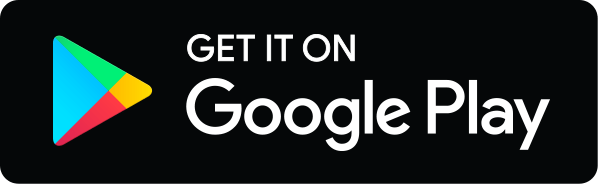