Soheil Kooraki1 and Hossein Jadvar2 1 Department of Molecular and Medical Pharmacology, David Geffen School of Medicine at UCLA, University of California, Los Angeles, CA, USA 2 Professor of Radiology, Urology, and Biomedical Engineering, Keck School of Medicine and Viterbi School of Engineering, University of Southern California, Los Angeles, CA, USA Imaging plays a pivotal role in the diagnostic pathway and management of prostate cancer. The imaging of prostate cancer has evolved during the past decade with image‐guided biopsy techniques and the development of diverse prostate‐specific molecular radiotracers. After a brief review of prostate embryology, anatomy, and prostate diseases, the use of correlative nonmolecular and molecular imaging modalities will be discussed. During the embryonic period in a 50‐mm male fetus, under the influence of testosterone produced by the Leydig cells of the fetal testis, the prostate gland starts to develop as epithelial buds from the walls of the urogenital sinus at the site of the Mullerian tubercle [1]. Resembling the shape of an inverted pyramid, the prostate gland base is located below the neck of the urinary bladder and the apex is located inferiorly at the level of the urogenital diaphragm. The normal adult prostate gland measures approximately 5 × 3 × 3 cm with a volume of 25 mL or less. The gland consists of four zones: the peripheral zone (PZ) is the outermost zone, comprising 70–80% of the glandular tissue, the transition zone (TZ) wraps around the urethra proximal to the verumontanum comprises 5% of the glandular tissue, the central zone (CZ) surrounds the ejaculatory ducts and comprises 20% of the glandular tissue, and the anterior fibromuscular stroma (AFMS) does not contain any glandular tissue. The TZ is the main site of benign prostatic hyperplasia accounting for a higher proportion of the glandular tissue. In contrast, most (70%) prostate cancers arise from PZ, followed by TZ, and rarely CZ. Prostate cancer does not arise from AFMS, and the presence of prostate cancer in CZ or AFMS is usually the result of extension from PZ or TZ. Zonal anatomy might be appreciated on computed tomography (CT) scan with a more hypodense appearance of PZ. Prostate gland zonal anatomy is best delineated on magnetic resonance imaging (MRI), with PZ showing a higher T1‐weighted signal than TZ due to its greater water content. The normal CZ is visualized on T2‐weighted and diffusion‐weighted images as symmetric low‐signal areas surrounding the ejaculatory ducts bilaterally. The ejaculatory ducts join the prostatic urethra at the mid‐gland level of the prostate at a urothelial elevation called the verumontanum. AFMS is seen as a bilateral crescentic‐shaped low‐signal area on T2‐weighted imaging [2]. The term “prostate capsule” has been colloquially used, since it is a pseudo‐capsule formed by the fibromuscular tissue. The prostate pseud‐capsule is often seen as a rim of T2 hypointensity surrounding the gland. It fuses anteriorly with AFMS at the apex with the external urethral sphincter and at the base with the bladder detrusor. The posterior surface of the gland is separated from the rectum by a membrane called the rectovesical (Denovillier’s) fascia. The paired seminal vesicles are located superior and posterior to the gland and are seen as multicystic structures on MRI. The paired neurovascular bundles run at the posterolateral aspects of the gland at 5 and 7 o’clock, and comprise branches of inferior vesicular artery, accompanying veins and nerves. The venous plexus of Santorini surrounds the prostate anteriorly and anterolaterally. The prostatic venous complex drains to the paravertebral venous plexus and the inferior vesicular vein. The communication between the prostatic and prevertebral venous complexes is responsible for the high frequency of vertebral metastasis from prostate cancer. Benign prostatic hyperplasia (BPH) is the most common symptomatic disease of the prostate gland, with a prevalence of 50–60% by the age of 60. BPH occurs as the result of glandular and stromal proliferation, and often leads to urinary tract symptoms [3]. The diagnosis is clinical and a variety of medical and interventional treatment options are available. Prostatitis is a general term which encompasses four distinct clinical entities: acute bacterial prostatitis, chronic bacterial prostatitis, inflammatory or noninflammatory pelvic pain syndrome, and asymptomatic prostatitis. Overall prevalence is 8.2%, the diagnosis is clinical, and imaging is rarely requested for diagnosis [4]. Prostate cancer is the leading cause of malignancy and the second leading cause of cancer‐related mortality in men in the United States. Twelve‐core trans‐rectal ultrasound biopsy (TRUS) is typically performed if there is clinical suspicion for malignancy based on elevated or rising serum prostate specific antigen (PSA) level or abnormal digital rectal examination (DRE). Developments in the multiparametric MRI of the prostate have revolutionized the work‐up of prostate cancer, with MRI‐guided biopsy methods now widely used in clinical practice. The prostate cancer grading system has long been based on the greatest Gleason score on pathology. A new prostate cancer grading system was introduced [5] by the International Society of Urological Pathology (ISUP) in 2014 which assigns Gleason groups 1–5 derived from the more traditional Gleason scores. This grading system has been validated in large cohorts and is now widely accepted [6, 7]. Cohort studies have found that the cancer‐related mortality is low in patients with low‐grade prostate cancer, and they do not benefit from invasive treatments. These patients are typically followed with active surveillance, while those with unfavorable intermediate‐grade or high‐grade prostate cancer require more extensive diagnostic workup and treatment planning. Radical prostatectomy, external beam radiation therapy or brachytherapy is used for the treatment of localized nonmetastatic prostate cancer. After curative‐intent treatment, patients undergo regular follow‐up with serum PSA to detect possible biochemical recurrence (BCR), which occurs in about 40% of men over a decade post initial definitive therapy (Table 19.1). When higher serum PSA level (>10 ng/mL) is associated with BCR of treated prostate cancer, CT scan and bone scan are employed. In lower serum PSA levels, the sensitivity of these conventional imaging studies decreases markedly, necessitating the use of more sophisticated modalities. Early and accurate detection of the sites of recurrent disease is of paramount importance for patient management and treatment, since local salvage therapy is used for pelvic recurrence and systematic treatments are used for metastatic disease [10, 11]. Locoregional salvage therapies are most effective with serum PSA <1 ng/mL [12]. Metastasis‐directed therapy (MDT) may also be considered in patients with oligometastatic disease (typically up to five lesions identified on imaging). Comprehensive guidelines for the initial diagnosis, management, and treatment of prostate cancer can be found at the National Comprehensive Cancer Network (NCCN) website [13]. Table 19.1 Definition of commonly used clinical terms in patients with prostate cancer. With a distended urinary bladder, the prostate gland can be grossly assessed on pelvic ultrasound with a curved array transducer. On ultrasound, BPH is identified with visualization of an enlarged prostate (volume >30 mL), heterogeneous TZ, bladder wall hypertrophy and trabeculation, and post‐micturition urine residue in the urinary bladder. Gray‐scale transrectal ultrasound (TRUS) was introduced in 1968, but despite the development of higher frequency transducers and improved signal reception, it is still not an optimal modality for detection of prostate cancer at early stages, with many prostate cancer foci appearing isoechoic on TRUS. Color Doppler ultrasound improves the diagnostic performance of gray‐scale TRUS. In 1989, the first TRUS‐guided systematic sextant biopsy protocol was described, and gradually the 12‐sysmetic biopsy turned into the standard of care for the diagnosis of prostate cancer. Biopsy is performed after initiation of prophylactic antibiotics (fluroquinolones by choice, a widely used regimen is 500 mg of ciprofloxacin 1 day prior to biopsy and for 2 days after) and under local anesthesia. Contrast‐enhanced TRUS and elasto‐sonography can augment visualization of suspicious foci for targeted TRUS biopsy. Biopsy is carried out using a guided biopsy system attached to the transrectal probe. Overall, CT scan is an insufficient modality for assessment of prostatic disease. Prostate volume measurement might be overestimated on CT scan. In daily practice, a prostate gland with a transverse diameter of greater than 4.5 cm in the axial plane is considered enlarged. Prostate calcifications are frequently seen on CT scan, without clear clinical significance. In the setting of BPH, enlarged prostate gland, heterogenous appearance of TZ with visible high‐ or low‐density nodules, and, in severe cases, protrusion of the gland into the urinary bladder might be seen. Bladder wall thickening and trabeculation are indirect clues for the presence of BPH. With acute prostatitis, the prostate gland becomes enlarged and edematous, and if a prostate abscess develops, a rim‐enhancing unilocular or multilocular hypodense area in the PZ is expected on contrast‐enhanced CT scan. CT scan is limited for the primary detection of prostate cancer, but focal increased enhancement in the PZ of prostate gland is described in some patients with prostate cancer. In high‐grade disease or when there is suspicion for locoregional or distant metastasis, chest and abdominopelvic CT scan are commonly used for detection of nodal and soft tissue metastasis, and planning for radiotherapy. MRI is not routinely used for the diagnosis of benign prostate conditions. MRI, however, is superior to ultrasound for classification of BPH subtypes and estimation of glandular/stromal ratio, findings which can help to tailor treatment. On MRI, chronic prostatitis and granulomatous prostatitis can cause signal abnormality and mimic prostate cancer [14]. The potential application of MRI in prostate cancer was first described in 1982 [15]. With remarkable advances in image acquisition techniques, postprocessing software, and standardized interpretation guidelines during the past decade, multiparametric prostate MRI (mpMRI) has turned into the imaging modality of choice when assessment of intraprostatic malignancy or image‐guided prostate biopsy is indicated. Prostate mpMRI is generally recommended when there is either (i) clinically high suspicion for intermediate or high‐grade prostate cancer (based on serum PSA measures and findings of DRE) in patients who had a prior negative transrectal ultrasound guided biopsy or (ii) restaging or re‐biopsy planning in patients with known low‐grade prostate cancer under active surveillance [16]. The American Urologic Association currently does not recommend mpMRI for the purpose of prostate cancer screening or surveillance [17]. Adapted from a similar scoring system in breast cancer, the European Society of Urogenital Radiology (ESUR) introduced the first Prostate Imaging‐Reporting and Data System (PI‐RADS) in 2012, which included guidelines for prostate MRI acquisition, interpretation, and reporting [18]. PI‐RADS version 1 (v1) was succeeded by recommendations of a steering committee from the American College of Radiology (ACR), ESUR, and the AdMeTech Foundation, formulated as PI‐RADS v2. in 2016 [19] followed by PI‐RADS v2.1 in 2019 [16]. Since their introduction, PI‐RADS guidelines have been widely validated and right now are widely applied to daily practice in the United States and Europe. PI‐RADS guidelines assess the probability of clinically significant prostate cancer on a five‐point scale for each lesion on a standard prostate mpMRI (Table 19.2). For the acquiring prostate mpMRI, most experts recommend using a 3 T MRI scanner when available. Compared to 1.5 T scanner, a 3 T scanner is more prone to the susceptibility artifact, which might compromise the image quality (particularly in diffusion‐weighted imaging [DWI]), and therefore in specific situations (such as the presence of metallic hip prosthesis) a 1.5 T scanner might be preferred. An external phased array coil is used with or without an endorectal coil. An endorectal coil increases signal‐to‐noise ratio (SNR), particularly in larger patients, but it increases the time and cost of the scan, and is associated with patient discomfort. Rectal evacuation before examination is recommended. The use of antispasmodic agents (such as glucagon and hyoscine bromide) to decrease bowel peristalsis and rectal gas is controversial. Table 19.2 The likelihood of clinically significant prostate cancer with each PI‐RADS score and the related recommendation. Prostate multiparametric MRI initially included T1‐weighted imaging (T1WI), T2‐weighted imaging (T2WI), DWI with its corresponding apparent diffusion coefficient (ADC) map, dynamic contrast‐enhanced imaging (DCE), and magnetic resonance spectroscopy (MRS). With the widespread use of DWI, and based on the recommendations of PIRADS v.2, MRS has now fallen out of favor. For interpretation of prostate mpMRI, the PZ and TZ should be separately scrutinized for the presence of focal lesions, with the assessments primarily based on DWI/ADC at PZ and T2WI at TZ. DCE has a secondary role in PZ lesions with PI‐RADS score 3 (Figure 19.1). T1‐weighted imaging is not part of the PIRADS criteria but it is useful for identification of intraprostatic hemorrhage and for detection of pelvic lymph node or bone involvement. The details of PI‐RADS guidelines for the acquisition, interpretation, and reporting of prostate mpMRI are available at the American College of Radiology website (https://www.acr.org/‐/media/ACR/Files/RADS/Pi‐RADS/PIRADS‐V2‐1.pdf). In a meta‐analysis of 21 studies (3857 patients), PIRADS v2 had pooled sensitivity and pooled specificity of 89% and 73%, respectively, for detection of prostate cancer, using prostatectomy or prostate biopsy as the reference standard [20]. Prostate mpMRI has significantly higher sensitivity and negative predictive value for the detection of clinically significant prostate cancer compared to TRUS biopsy, which indicates that acquiring prostate mpMRI could potentially avoid biopsy in up to 27% of men at risk [21]. Systematic TRUS‐guided biopsy commonly oversamples clinically insignificant disease and has a limited role for the diagnosis of prostate cancer in TZ. Based on the results of the PRECISION trial (which included 500 biopsy‐naïve patients), performing MRI with or without MRI‐targeted biopsy results in 12% higher detection of clinically significant prostate cancer and 13% lower detection of clinically insignificant cancers compared to TRUS‐guided biopsy [22]. MRI‐targeted biopsy is usually indicated with prostate lesions PIRADS categories 3, 4, and 5. Several approaches are used for performing MRI‐targeted biopsy of prostate without an absolute consensus on the preferred method. MRI‐targeted prostate biopsy might be performed via three approaches: MRI‐ultrasound fusion biopsy, cognitive MRI‐guided biopsy, and direct in‐bore MRI‐guided biopsy. In MRI‐ultrasound fusion biopsy, the previously acquired MRI data are fused on the real‐time TRUS on a fusion platform and sampling is performed under the tracking system. In cognitive MRI‐guided biopsy, the operator mentally maps (i.e. cognitively fuses) the previously acquired MRI target to the real‐time TRUS and guides the needle to this location. Finally, in‐bore MRI‐guided biopsy is performed under direct MRI guidance while the patient is lying in the MRI gantry. Figure 19.1 PI‐RADS v2.1 scoring schematic in the peripheral zone (PZ) and transition zone (TZ). Overall, with MRI‐targeted approaches there is a significantly higher detection rate of clinically significant prostate cancer (relative risk [RR] 1.16) and a significantly lower detection rate of clinically insignificant cancer (RR 0.47) over TRUS‐guided biopsy [23]. There is not any significant difference in detection of clinically significant prostate cancer between MRI‐ultrasound fusion biopsy, cognitive MRI‐ultrasound fusion biopsy, and direct in‐bore MRI‐guided biopsy. Direct MRI‐guided biopsy, however, allows direct visualization of suspicious lesions with higher core positivity rate (47.7%) compared to cognitive MRI‐guided biopsy (33.3%) and MRI‐ultrasound fusion biopsy (31.3%) [24]. Cost‐effectiveness studies have found both MRI‐based approaches to have higher quality‐adjusted life‐year benefits [25, 26]. The optimal biopsy approach should be chosen per patient and per center basis, considering the availability of biopsy platforms and the experience of the performing operators. Fluorodeoxyglucose (FDG) is a synthetic glucose analog that is taken up by glucose transporters (GLUTs), phosphorylated by hexokinase, and trapped in the cells. Most malignant tumors demonstrate enhanced glucose metabolism, increased hexokinase enzymatic activity, and upregulation of GLUTs (Warburg effect) compared to normal tissue. With relatively low glucose metabolism in the majority of prostate cancers, FDG PET/CT has a limited role in the primary detection, staging, and restaging of prostate cancer [27]. Castration‐resistant prostate cancer is typically FDG avid, and therefore FDG PET/CT might be useful for assessment of its treatment response and prognostication (time to hormonal treatment failure in castrate‐sensitive disease and overall survival in castrate‐resistant metastatic disease) (Figure 19.2) [28]. PET Response Criteria in Solid Tumors (PERCIST) 1.0, particularly in combination with PSA response criteria [29], sum of SUVmax, and the number of FDG‐avid lesions [30], can independently provide prognostic information in this group of patients. Incidental focal intraprostatic FDG uptake is nonspecific but may carry a risk of malignancy. The prevalence of incidental high intraprostatic activity is 1.8%, with at least 17% risk of malignancy [31]. Some investigators have suggested that focal incidental intraprostatic FDG activity with SUV more than 6 should be further assessed by prostate mpMRI or serum PSA level [32]. Choline, as a component of the cytoplasmic membrane phospholipid layer, is phosphorylated by the enzyme choline kinase and is trapped in the cell membrane in the form of phosphatidylcholine [33]. The upregulation of choline kinase in prostate cancer is the most likely mechanism leading to enhanced expression of choline in prostate cancer. The most available radiolabeled choline ligands are 11C‐choline, 18F‐fluoroethylcholine, and 18F‐fluoromethylcholine (latter two are commonly known as 18F‐fluorocholine or FCH). Normal biodistribution of 11C‐choline demonstrates relatively high accumulation in the pancreas, liver, kidneys, and salivary glands, and variable uptake in the bowel, with minimal urinary excretion (latter only with 11C‐choline) [34]. The short half‐life (~20 minutes) of 11C limits its utility to institutions with an on‐site cyclotron. 18F‐labeled choline radiotracers have a longer half‐life (~110 minutes) and allow transportation from off‐site cyclotrons. With a shorter position range of 18F‐labeled choline ligands, the spatial resolution of images improves, but the higher urine activity with 18F‐choline in contrast to 11C‐choline is disadvantageous. Physiologic ureteric or urinary bladder activity might limit assessment of prostate bed or pelvic nodal involvement. With 11C‐choline, imaging starts immediately after injection, while with 18F‐choline imaging starts at, most commonly, 60 minutes post injection. When 18F‐choline is used, additional post‐micturition images may help distinguish pathologic activity from physiologic urine activity. Choline PET/CT is not indicated for the detection of primary prostate cancer or routine initial staging. Tracer uptake in prostatic intraepithelial neoplasia and benign conditions such as prostatitis and BPH lowers its specificity for assessment of intraprostatic malignancy. 18F‐fluorocholine PET/CT has slightly higher sensitivity and specificity compared to 11C‐choline. Choline PET/CT is more accurate than conventional imaging for detection of pelvic nodal disease at initial staging with pooled sensitivity and specificity of 62% and 92%, respectively [35]. Figure 19.2 Treatment response evaluation with FDG PET/CT in metastatic castration‐resistant prostate cancer. The patient was scanned at baseline and at 4, 8, and 12 months (rows) after the start of docetaxel chemotherapy. From left to right columns: Axial CT at bone window level, axial FDG PET, axial fused FDG PET/CT, sagittal CT at bone window level, FDG MIP images, and bone scan. The FDG MIP images clearly demonstrate decline in the total metallically active tumor burden as result of favorable response to docetaxel chemotherapy in concordance with the decline in the serum PSA level while CT and bone scan are inconclusive. (Courtesy of Hossein Jadvar, MD, PhD, University of Southern California, Los Angeles, CA, USA; NIH R01‐CA111613.) When there is biochemical recurrence of treated prostate cancer, choline PET/CT has higher accuracy than conventional imaging modalities; however, the disease detection is highly influenced by serum PSA level and the sensitivity of the scan drops sharply, with 80% of scans being negative at PSA levels <1 ng/mL [36]. In a meta‐analysis of 2126 patients with biochemical recurrence of treated prostate cancer, the pooled detection rate of 11C‐choline was 62%. The pooled sensitivity and specificity in 1270 patients were both 89% [37]. On September 12, 2012, 11C‐choline was approved by the United States Food and Drug Administration (FDA) for imaging of patients with suspected prostate cancer recurrence and noninformative bone scan, CT, or MRI. Acetate, a key substrate in energy metabolism, provides the only carbon source for fatty acid synthesis. Fatty acid synthesis is enhanced in prostate cancer cells as a response to increased demand for energy and cell membrane lipid production [38]. The tracer primarily accumulates in the myocardium, kidneys, pancreas, spleen, and bone marrow. An advantage of prostate imaging, is that urinary bladder activity is absent as a result of active reabsorption of 11C‐acetate in the renal proximal convoluted tubule [39]. 11C‐acetate PET is overall less investigated than 11C‐choline PET. In a similar fashion to choline‐based imaging, 11C‐acetate PET cannot reliably distinguish between BPH and prostate cancer, and therefore is not a practical modality for localization of intraprostatic, specifically low‐volume disease [38]. Some studies have shown that 11C‐acetate PET may be less sensitive than 11C‐choline PET for the detection of pelvic lymph nodes [40]. The diagnostic performance of 11C‐acetate PET/CT in patients with biochemical recurrence of prostate cancer is similar to that of choline PET/CT with low yield when serum PSA level is under 1–2 ng/mL [38]. In a study of 358 patients with biochemical recurrence of prostate cancer, the detection rate varied from 19% in those with a PSA level between 0.2 and 1 ng/mL, to 46% in those with a PSA level between 1 and 3 ng/mL, and to 82% in those with a PSA level of more than 3 ng/mL [41]. Amino acid metabolism and transport is upregulated in certain types of cancers, including prostate cancer. L‐11C methionine (MET) was one of the first tracers used for this purpose but it was not widely investigated and it is not currently used in clinical practice. Anti‐1‐amino‐3‐18F‐fluorocyclobutane‐1‐carboxylic acid (18F‐FACBC, also known as 18F‐fluciclovine or commercially as Axumin; Blue Earth Diagnostics, Inc.) is a synthetic L‐leucine analog. This synthetic amino acid is transported into cells via specific amino acid transporters, such as alanine‐serine‐cysteine transporter 2 (ASCT2). ASCT2 is an essential transporter of glutamine, an amino acid which is upregulated in prostate cancer and plays an important role in the cancer signaling pathway. Fluciclovine does not undergo further intracellular metabolism and is not incorporated into protein structure [42]. Tracer activity is highest in the pancreas, with less activity in the liver, salivary glands, pituitary gland, marrow, lymphoid tissue, breast, gastrointestinal (GI) tract, adrenal glands, and renal parenchyma, with minimal urine activity (Figure 19.3a) [43]. Due to rapid influx and efflux of amino acids in prostate cancer, the peak activity happens very early after tracer injection, and therefore image acquisition should begin 3–5 minutes after injection to enhance the target‐to‐background ratio. The uptake is mainly assessed visually rather than by measuring SUV [44]. Table 19.3 provides a summary of 18F‐FACBC PET/CT scan interpretation. Additional comprehensive guidelines can be found in the literature. Figure 19.3 (a) Normal distribution of 18F‐fluciclovine PET. Note the intense activity in the pancreas, minimal urine activity, and diffuse low‐level marrow activity. (b) Normal distribution of 68Ga‐PSMA‐11 PET is similar to (c) 18F‐DCFPyL PET. Note the urine activity and significant GI tract activity in both scans. 18F‐DCFPyL PET tends to have higher liver, and lower renal and spleen activity compared to 68Ga‐PSMA‐11 PET. (Images a and b courtesy of Jeremie Calais, MD, David Geffen School of Medicine at University of California Los Angeles (UCLA); image C courtesy of Gholam Reza Berenji, MD, VA Healthcare Greater Los Angeles, David Geffen School of Medicine at UCLA, Los Angeles, CA.) Table 19.3 Summary of guidelines for interpreting 18F‐FACBC PET/CT scan. Fluciclovine is most beneficial in individuals with biochemical recurrence of treated prostate cancer, but it has low sensitivity for nodal staging and is not recommended or approved for initial staging of prostate cancer. A metanalysis of 13 studies (563 patients) showed that fluciclovine PET/CT has pooled sensitivity and specificity of 87% and 84%, respectively, for the detection of primary prostate cancer, pooled sensitivity and specificity of 56% and 98%, respectively, for nodal staging, and pooled sensitivity and specificity of 79% and 69%, respectively, for localization of recurrent disease [45]. Fluciclovine is superior to choline PET/CT for the detection of intraprostatic, lymph node, and bone lesions in biochemical recurrence after radical treatment of prostate cancer [46]. In a limited evaluation, prostate 18F‐fluciclovin PET/MRI was found to have high specificity but low sensitivity for lymph node detection in high‐risk prostate cancer before undergoing radical prostatectomy, and lymph nodes smaller than 8 mm might not show tracer activity [47]. On May 27, 2016, 18F‐FACBC was approved by the United States FDA for imaging prostate cancer in patients with suspected recurrence based on rising serum PSA after prior definitive primary treatment. With remarkable advances in PSMA‐based tracers, 18F‐FACBC has started to fall out of favor (Figure 19.4). Prostate‐specific membrane antigen (PSMA), also known as glutamate carboxypeptidase II or folate hydrolase I, is a type II transmembrane glycoprotein (100–120 kDa) which is mostly localized to the intracellular compartment in normal prostate gland cells, but in 90% of prostate cancers it highly expresses in the extracellular luminal compartment [48]. PSMA expression is 100–1000 times higher in density in prostate cancer compared to normal non‐neoplastic prostatic tissue [49], and the expression enhances as the cancer becomes castrate‐resistant. PSMA expression in prostate cancer is probably related to its role in folate transportation and metabolism, which further facilitates cell proliferation [50]. The fraction of prostate cancers that do not express PSMA are typically more aggressive. PSMA has shown unique characteristics which have made it a practical molecular target for the development of small‐molecule labeled inhibitors. The first commercially available PSMA targeting antibody was 111In‐ capromab‐pendetide (ProstaScint, AYTU Bioscience, Englewood, USA), which was approved by the United States FDA in 1996 for single‐photon emission computed tomography (SPECT) imaging of biopsy‐proven prostate cancer localized to the prostatic bed but at high risk for pelvic lymph node metastasis. Unlike the modern PSMA ligands, ProstaScint binds only to the intracellular epitope of PSMA. ProstaScint showed moderate sensitivity for localization of primary and metastatic prostate cancer even with added SPECT/CT, and with emerging of more sensitive radiotracers it is no longer used in clinical practice. The development of PSMA ligands dates to as early as 2001, with the first clinical experiences reported between 2012 and 2015. The most studied and currently FDA‐approved PSMA‐targeted ligands are 18F‐DCFPyl, and 68Ga‐PSMA‐11 [51]. Another notable tracer is 18F‐PSMA‐1007, which has the advantage of predominant hepatobiliary excretion and minimal urine activity [52]. Extraprostatic PSMA expression is noted in the salivary glands, liver, spleen, kidneys, neural ganglia, duodenal mucosa, and other parts of the GI tract. Slight differences in uptake distribution exist between 68GA‐PSMA‐11 and 18F‐DCFPyL (Figure 19.3b,c), with 18F‐DCFPyL showing higher liver uptake and lower renal, spleen, and salivary glands uptake [53]. One of the most common pitfalls in the interpretation of PSMA‐based PET is the ganglia uptake, which might mimic pathologic lymph nodes. Commonly visualized ganglia on PSMA‐targeted PET/CT are cervicothoracic/stellate, coeliac, lumbar, and sacral ganglia (Figure 19.5). Correlation to the typical anatomic location and the more linear/triangular shape of ganglia on CT scan is helpful. PSMA expression is also described in other nonprostate pathologies, including, but not limited to, pulmonary sarcoidosis, Wegner’s granulomatosis, benign neurogenic tumors (i.e. meningioma, neurofibroma, paraganglioma), benign bone diseases (e.g. fibro‐osseous disease, Paget’s disease), gynecomastia, and a few nonprostate malignancies (e.g. renal cell carcinoma, hepatocellular carcinoma) [54]. Figure 19.4 68‐year‐old male with high‐risk (Gleason score 4 + 4) prostate adenocarcinoma was referred for initial staging with 18F‐fluciclovine PET/CT as shown in (a) coronal MIP, (b) fused axial PET/CT, and the corresponding (c) axial PET and (d) axial CT scan. There is tracer activity in the right prostate midgland to apex (blue arrow), compatible with the location of the patient’s diagnosed malignancy. No suspicious metastatic lesion was seen in this scan. The patient underwent radical prostatectomy and bilateral pelvic lymphadenectomy, and was restaged as T3N1M0 at surgery. Three months after surgery, serum PSA level had increased to 6.4 ng/dL and (e) 68Ga‐PSMA‐11 PET/CT was acquired. (f) Upper abdomen axial PET and corresponding fused PET/CT shows PSMA expression in a right‐side rib without CT correlation (not shown). (g) Pelvic axial PET and fused PET/CT show PSMA expression in a tiny right perirectal nodule. (h) Pelvic axial CT and fused PET/CT show PSMA expression in the left sacral ala with corresponding faint sclerosis on CT scan. None of these findings on PSMA PET/CT had 18F‐fluciclovine uptake in the earlier scan. (Images courtesy of Jeremie Calais, MD, David Geffen School of Medicine at University of California Los Angeles (UCLA), Los Angeles, CA.) Figure 19.5 Fused 18F‐DCFPyL PET/CT scan and corresponding CT in three different patients show physiologic PSMA expression in the cervicothoracic stellate ganglia (a and b, red arrows), celiac ganglion (c and d, yellow arrows), and sacral ganglia (e and f, gray arrows). Correlating with the characteristic location and shape of the ganglia on CT scan is helpful to avoid misinterpretation. (Images courtesy of Gholam Reza Berenji, MD, VA Healthcare Greater Los Angeles, David Geffen School of Medicine at UCLA, Los Angeles, CA.) PSMA PET/CT is consistently found to outperform other molecular and nonmolecular imaging modalities, with overall more accurate initial staging, higher detection rate of lymph node metastasis, and higher lesion uptake with lower background activity (Figures 19.6 and 19.7) [55]. 68Ga‐PSMA‐11 is more sensitive than 11C/18F‐choline and 18F‐fluciclovine in detecting recurrence at PSA levels <2.0 ng/mL [42]. PSMA ligands have moderate‐to‐high sensitivity and very high specificity for lymph node detection, and have enabled localization of positive lymph nodes in stations which are otherwise overlooked, including deep posterior pelvic, mesorectal, and supraclavicular stations. The mesorectum/posterior pelvic area is not usually included in the lymph node dissection or the radiation field unless the involvement is known prior to the treatment (Figures 19.8 and 19.9) [56]. Imaging usually begins 60 minutes after injection of PSMA ligand, however with 18F‐DCFPyL a 2‐hour and with 68Ga‐PSMA‐11 a 3‐hour post‐injection image might improve tumor localization [56]. 18F labeling carries several advantages over 68Ga labeling, including longer half‐life (110 vs. 68 minutes) and more availability of cyclotron‐produced 18F than 68Ga, which needs a germanium generator. Higher image resolution and lower urinary excretion with 18F‐labeled ligands are also advantageous [57]. In a meta‐analysis of 37 studies (4790 patients with BCR of prostate cancer), the detection rate of 68Ga‐PSMA PET/CT scan at PSA categories 0–0.19, 0.2–0.49, 0.5–0.99, 1–1.99, and ≥2 ng/mL was 33%, 45%, 59%, 75%, and 95%, respectively [58]. According to the results of the CONDOR trial, 18F‐DCFPyL PET/CT results in a correct cancer localization rate of 84.8–87% with a change in the initially intended management plan in 63.9% of patients with BCR of prostate cancer [59]. 18F‐DCFPyL might allow detection of additional lesions, specifically at serum PSA levels <0.5 ng/mL, in comparison with 68Ga‐PSMA‐11 [60]. European Association of Urology (EAU) guidelines on prostate cancer recommend the use of PSMA PET imaging for any case of biochemical recurrence after radical prostatectomy (PSA >0.2 ng/mL) [61] On December 1, 2020, 68Ga‐PSMA‐11 was approved by the United States FDA, followed by the approval of 18F‐DCFPyL on May 27, 2021. According to the approval statements, both agents can be used for the initial staging of prostate cancer with suspected metastasis in patients who are considered for initial definitive therapy or in the setting of suspected recurrent prostate cancer based on the rising serum PSA after definitive radiotherapy or radical prostatectomy. A multidisciplinary workgroup has published appropriate use criteria which describe the clinical appropriateness of PSMA PET imaging in different scenarios of prostate cancer [62]. Figure 19.6 73‐year‐old male with castration‐resistant oligometastatic prostate cancer, referred for restaging. (a) Axial fused 18F‐DCFPyL PET/CT scan shows PSMA expression (orange arrow) in the left mid‐gland prostate, corresponding to the hypointense lesion (orange circle) on (b) T2‐weighted image. (c) PSMA expression (yellow arrow) is seen in a left pelvic sidewall lymph node on fused PET/CT which corresponds to the suspicious lymph node (yellow circle) on the (d) T2‐weighted image. (e) Fused 18F‐DCFPyL PET/CT scan shows PSMA expression on the anterior aspect of the T9 body, corresponding to sclerotic lesion on the (f) axial CT scan and (g) tracer uptake on 18F‐NaF bone PET. (h) Intense PSMA expression is also seen in the right fourth rib (light blue arrow) on the fused 18F‐DCFPyL PET/CT scan, corresponding to (i) focal sclerotic lesion on the CT scan (light blue arrow), compatible with metastasis. (j) The baseline coronal MIP 18F‐DCFPyL PET/CT scan again shows PSMA expression in the prostate gland, regional lymph node, T10 body, and right fourth rib. Following radiation therapy to the prostate gland and pelvic lymph node, stereotactic body radiation therapy to the rib and vertebral lesions combined with androgen deprivation therapy and enzalutamide. (k) Repeat 18F‐DCFPyL PET/CT scan in 9 months shows resolution of PSMA expression in all regions, suggestive of excellent treatment response. (Images courtesy of Gholam Reza Berenji, MD, VA Healthcare Greater Los Angeles, David Geffen School of Medicine at UCLA, Los Angeles, CA.) Several guidelines have attempted to standardize the interpretation and reporting of PSMA PET/CT. Based on Prostate Cancer Molecular Imaging Standardized Evaluation (PROMISE), the molecular imaging TNM (miTNM) version 1.0 was proposed in 2018. miTNM staging combines the anatomic distribution of the disease and the degree of PSMA expression (miPSMA scores) (Tables 19.4 and 19.5). For intraprostatic tumor evaluation, miTNM suggests the use of complementary mpMRI using a hybrid PET/MRI or separate PET/MRI fusion [63]. Figure 19.7 64‐year‐old male with history of high‐risk prostate cancer status post radiotherapy and androgen deprivation therapy presented with biochemical recurrence (serum PSA 5.26 ng/mL). (a, b) Axial fused PET/CT and its corresponding CT scan show a subcentimeter left supraclavicular PSMA‐positive lymph node (blue arrows). (c, d) Axial fused PET/CT and its corresponding CT scan show a small PSMA‐positive sclerotic lesion in right humeral neck (orange arrows). No other PSMA‐positive foci were seen. (Images courtesy of Gholam Reza Berenji, MD, VA Healthcare Greater Los Angeles, David Geffen School of Medicine at UCLA, Los Angeles, CA.) Figure 19.8 68Ga‐PSMA‐11 image in two different patients acquired for localization of biochemical recurrence. Patient 1: (a) coronal MIP, (b) axial CT and its corresponding PET/CT, (c) axial PET shows PSMA expression in the right iliac bone without CT correlation. This finding suggests skeletal metastasis. Patient 2: (d) coronal MIP, (e) axial CT and its corresponding PET/CT, (f) axial PET shows PSMA expression in a tiny left peri‐rectal nodule (not positive by CT criteria), compatible with metastasis. Figure 19.9 18F‐DCFPyL PSMA PET/CT in three different patients shows PSMA‐positive metastatic lesions which are not positive by CT criteria only. (a, b, c) Fused PSMA PET/CT and coned‐down images show PSMA expression in left second rib corresponding to very faint sclerosis on CT scan. (d, e, f) PSMA expression in a retroperitoneal para‐aortic lymph node which measures 6.8 mm on the short‐axis diameter and would not have been called positive based on the size criteria only. (g, h, i) PSMA expression in a very small right peri‐rectal soft tissue nodule. (Images courtesy of Gholam Reza Berenji, MD, VA Healthcare Greater Los Angeles, David Geffen School of Medicine at UCLA, Los Angeles, CA.) Table 19.4 miPSMA scoring system. The PSMA Reporting and Data System (PSMA‐RADS) has been proposed, which uses a five‐point scale for categorization of positive findings, considering the anatomic location, the degree of PSMA expression, and anatomic image correlation. Up to five target lesions can be defined by the reader based on the expression intensity and diameter, and then the final overall PSMA‐RADS score is defined as the highest scored target lesion among these five representative target lesions. A high inter‐reader agreement is found for the use of PSAM‐RADS in the interpretation of PSMA‐targeted PET/CT [64]. A number of other radiotracers have been under investigation, with most of them lacking enough reliable data to be used in clinical practice. Androgen receptor overexpression has been described in prostate cancer. 16β‐18F‐fluoro‐5α‐dihydrotestosterone (FDHT), an androgen receptor ligand, has been suggested to be useful for estimation of androgen receptor saturation and the optimal anti‐androgen dose. Relatively sparse data are available regarding the accuracy of this class of radiotracer. Gastrin‐releasing peptide receptor (GRPR) is overexpressed in prostate cancer with little or no expression in nonmalignant prostate tissue. Bombesin is a shortened analog of the human GRP with very high affinity to GRPR. Preliminary data has shown the degree of 68Ga‐RM2 uptake, a bombesin‐based GRPR antagonist radiotracer, in malignant lesions is similar to 68Ga‐PSMA‐11, and that the two radiotracers are complementary [65]. Table 19.5 A summary of miTNM classification for PSMA‐based PET/CT or PET/MRI. The prostate gland frequently demonstrates diffuse low‐level uptake (SUV between 3.5 and 7) on somatostatin receptor‐specific PET/CT scans, such as 68Ga‐DOTATOC and 68Ga‐DOTATATE, with higher diffuse uptake seen in the setting of prostatitis. A fraction of prostate cancers might present with neuroendocrine differentiation, mimicking the features of neuroendocrine tumors. Neuroendocrine‐differentiated prostate cancer can arise de novo or from transformation of a prostate cancer under prolonged androgen deprivation therapy. This type of prostate cancer is typically rapidly progressive, with early visceral metastasis. Pure neuroendocrine‐differentiated prostate cancer does not express PSMA and therefore PSMA‐targeted PET/CT is negative in these patients [56]. 68Ga‐DOTATOC and 68Ga‐DOTATATE PET/CT will show intense focal activity and are useful for the assessment of disease burden in this type of prostate cancer [66]. The possibility and effectiveness of peptide receptor radionuclide therapy (PRRT) using 177Lu‐DOTATATE in patients with castration‐resistant, neuroendocrine‐differentiated prostate cancer is under investigation.
19
Correlative Approach to Prostate Imaging
Background
Prostate Embryology and Anatomy
Prostate Diseases
Term
Definition
Clinically significant prostate cancer
Histologic Gleason score >7 (including 3 + 4 with prominent but not predominant Gleason 4 component) and/or volume>0.5 mL and/or the presence of extraprostatic extension
Biochemical recurrence
Biochemical recurrence of prostate cancer is defined as either (a) PSA >0.2 ng/mL 6 weeks after prostatectomy 6 weeks, confirmed by a second PSA measurement (AUA) or (b) PSA increase greater than 2 ng/mL above nadir after radiation therapy or brachytherapy (ASTRO Phoenix) [8, 9]
Oligometastatic disease
Limited number of metastatic lesions (up to three to five lesions) in up to two organs
Castration‐resistant prostate cancer
Progressive disease despite androgen deprivation therapy and low levels of testosterone
Nonmolecular Conventional Prostate Imaging
Ultrasound and Ultrasound‐guided Biopsy
CT Scan
Multiparametric MRI and MRI‐directed Biopsy
PI‐RADS score
Likelihood of clinically significant prostate cancer
Recommendation
PI‐RADS 1
Very low
Follow‐up
PI‐RADS 2
Low
Follow‐up
PI‐RADS 3
Intermediate
Depends on pre‐test probability, most guidelines recommend MRI‐directed biopsy
PI‐RADS 4
High
MRI‐directed biopsy
PI‐RADS 5
Very high
MRI‐directed biopsy
Molecular Prostate Imaging
Fluorodeoxyglucose
Choline
Acetate
18F‐fluciclovine
Uptake location
Positive criteria suspicious for malignancy
Focal soft tissue lesions ≥1 cm
Tracer activity equal to or greater than the mean activity of bone marrow (using L3 as the standardized reference)
Focal soft tissue lesions <1 cm
Tracer activity significantly greater than that of the mean blood pool activity
Skeletal uptake
Focal skeletal uptake which is unequivocally visualized on MIP images of PET is regarded as suspicious for malignancy
18F‐fluciclovine shows uptake in osteolytic and osteoblastic lesions, but densely sclerotic osseous lesions might not show uptake
Prostate‐Specific Membrane Antigen Ligands
miPSMA score
Degree of tracer uptake
Reported PSMA expression
0
Less than blood pool
No
1
Equal to or greater than blood pool, less than liver
Low
2
Equal to or greater than liver, less than parotid gland
Intermediate
3
Equal to or greater than parotid gland
High
Other Molecular Tracers
miTNM staging
Description
T
miT0
No local tumor
miT2
Tumor confined to prostate, unifocal (u) or multifocal (m)
miT3
Tumor with extracapsular extension (miT3a), invading seminal vesicles (miT3b)
miT4
Tumor invading local structures other than seminal vesicles
miTr
Local tumor recurrence after radical prostatectomy
N
miN0
No regional nodal metastasis
miN1a
Single regional positive lymph node
miN1b
Multiple regional positive lymph nodes
M
miM0
No distant metastasis
miM1a
Extrapelvic positive lymph nodes
miM1b
Skeletal metastasis
miM1c
Other sites of metastasis
PSMA‐RADS score
Description
Interpretation
PSMA‐RADS‐1
Lesions with or without tracer uptake that are definitely benign
Definitely benign
PSMA‐RADS‐2
Low‐level bone/soft tissue PSMA expression in atypical sites of prostate cancer, likely benign
Likely benign
PSMA‐RADS‐3
PSMA‐RADS‐3A: Soft tissue in a typical location of prostate cancer with equivocal PSMA expression
Equivocal
PSMA‐RADS‐3B: Indeterminate bone lesion with equivocal PSMA expression
PSMA‐RADS‐3C: Atypical lesions for prostate cancer with high PSMA expression, might be malignancy of nonprostate origin
PSMA‐RADS‐3D: Lesions concerning for malignancy (of prostate or nonprostate origin) without PSMA expression
PSMA‐RADS‐4
Lesions with PSMA expression typical of prostate cancer without definite corresponding anatomic abnormality
Likely malignant
PSMA‐RADS‐5
Lesions with PSMA expression typical of prostate cancer with corresponding anatomic abnormality
Definitely malignant
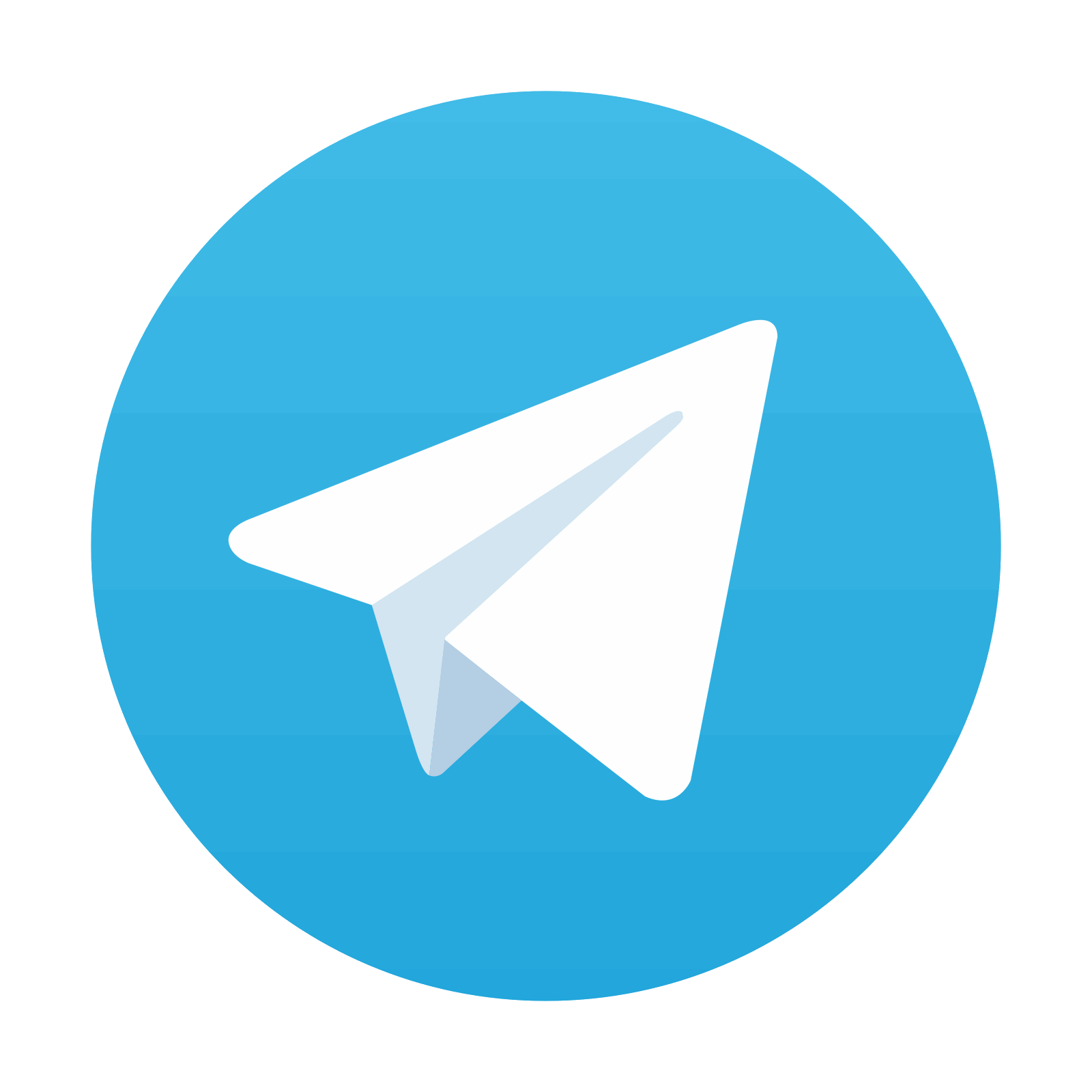
Stay updated, free articles. Join our Telegram channel

Full access? Get Clinical Tree
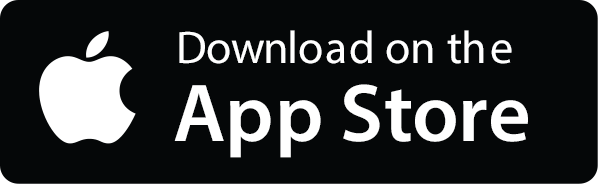
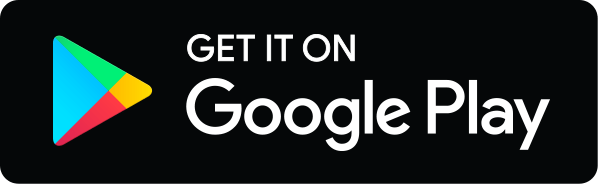