Diffusion sensitized MRI (dMRI) provides several useful metrics for the detection and characterization of traumatic brain injury (TBI).1 This chapter discusses applications of directionally nonselective dMRI techniques, such as diffusion weighted MRI (DWI), as well as directionally sensitive approaches, such as diffusion tensor MRI (DTI), for detection of brain tissue injury in patients with TBI. The chapter emphasizes the unique contribution of DWI and DTI for detection of pathology that is not revealed by other imaging modalities. TBI is a major cause of morbidity and mortality worldwide. Each year, in the United States alone, more than 2.5 million TBIs occur; over 55,000 individuals die, over 300,000 are hospitalized, and more than 2 million are treated and released from emergency departments.2 An unknown but likely very large number of TBIs are unrecognized or dismissed.3 TBI is commonly classified as to its severity based on clinical examination at the time of injury. This classification, based largely on the Glasgow Coma Scale (GCS; scale range 3–15), delineates severe (GCS 3–8), moderate (GCS 9–12), and mild (GCS 13–15) TBI. This GCS-based classification is an excellent predictor of survival to hospital discharge, but does not effectively predict long-term outcome, especially with more mild degrees of injury.4 Additional factors used for classification include the duration of unconsciousness, the duration of posttraumatic amnesia, and the presence of focal neurological deficits and imaging abnormalities.5,6 Mild TBI (mTBI, also termed concussion) is the most common form of TBI.2 Patients with mTBI experience a disturbance of neurocognitive function following the injury, which may include confusion, disorientation, imbalance, and other features. Some, but by no means all, mTBI patients experience actual loss of consciousness.7 Diagnostic criteria for mTBI include no more than 30 minutes of unconsciousness, no more than 24 hours of amnesia, and absence of focal neurological deficits.8 mTBI is typically not associated with abnormalities on conventional computed tomography (CT) and magnetic resonance imaging (MRI).1 Notwithstanding the relatively mild initial clinical features and absence of conventional imaging abnormalities, clinical manifestations of mTBI do arise from brain pathology, particularly traumatic axonal injury (TAI).9 Most patients with mTBI will recover, but a significant minority (15–30%,) will sustain long-term adverse consequences, including persistent postconcussive symptoms, cognitive impairment, and behavioral dysfunction.7 Thus the consequences of “mild” TBI are by no means necessarily mild. High-profile coverage of sports and military-related mTBI and its long-term adverse effects, including delayed neurodegenerative disorders, such as chronic traumatic encephalopathy (CTE),10 have driven increased awareness of mTBI. Patients with GCS scores in the mTBI range (13–15) who do have gross imaging abnormalities are often classified as mild-complicated TBI. These individuals may have worse prognosis for recovery.11 TBI pathology is commonly classified based on its gross pathological and imaging features as focal or diffuse injury.9 Focal injury may be extra-axial (skull fracture and epidural, subdural, or subarachnoid hemorrhage) or intra-axial (contusion, laceration, and hematoma). Much TBI tissue pathology, however, is a diffuse (or, more properly, multifocal) microscopic injury to the white matter of the brain, caused by linear and rotational forces. This pathology is termed traumatic axonal injury (TAI). When widespread it is often referred to as diffuse axonal injury (DAI). Pathological evidence of TAI has been demonstrated in all degrees of TBI severity, including mTBI. Many types of focal TBI pathology, including hemorrhage and contusion, are readily detected using CT and conventional MRI techniques, particularly those sensitive to hemorrhage such as T1-weighted and T2*-weighted imaging. Although microhemorrhage, as detected with susceptibility-weighted MRI (SWI), may be detectable in patients with TAI, widespread TAI may go entirely undetected on conventional MRI scans.1 This is because significant injury to axons occurs due to forces that do not rupture even the smallest blood vessels, which are at least 10 times the diameter of and more resilient than axons. Diffuse injury, TAI, is the major determinant of long-term symptoms, deficits, and disability in patients with TBI. Dysfunction arising from TBI is thought to be due to widespread brain network dysfunction, which can result from even microscopic axonal injury.12,13 In order to understand the nature of dMRI changes seen in the context of TBI, it is essential to understand the pathogenesis of brain tissue pathology underpinning the imaging changes. Moderate and severe TBI commonly entail frank physical trauma to the brain surface resulting in contusional injury. In all but the most severe TBI, however, frank disruption of deeper brain tissue does not result from the traumatic event. Rather, shear, stress and rotational force applied to white matter axons cause intra-axonal cytoskeletal alterations, such as microtubule damage and neurofilament misalignment, and set off a cascade of pathological cellular and molecular events.14 Component mechanisms include membrane pump dysfunction, inflammation, apoptosis, impaired function of cell survival pathways, and, eventually, loss of myelin and axons. TAI pathology evolves over a period of time (▶ Fig. 11.1), and the underlying pathological processes are typically not detectable using conventional CT and MRI.1,15 Tissue injury, particularly contusion as well as mass effect due to large hematomas, can lead to secondary ischemic injury or frank infarction, which may complicate the picture of focal TBI. Over the long term, loss of central nervous system (CNS) axons, due to processes including wallerian degeneration, ensues.14 White matter fibers remote from the apparent site of injury as well as cortical regions connected by these fibers may subsequently exhibit volume loss. Fig. 11.1 Timeframe of evolving pathological, imaging, and clinical features after traumatic brain injury. Note that diffusion sensitized magnetic resonance imaging features overlap and that diffusion tensor imaging findings persist into the chronic phase. As described elsewhere in this handbook, DWI has been known to be a sensitive means for detection of cytotoxic edema due to ischemic tissue injury since its clinical application to stroke became widespread in the 1990s.16 Although the clinical utility and acceptance of DWI in stroke is well established, the cellular mechanisms underlying these diffusion changes remain controversial. Similar to stroke, restriction of isotropic diffusion is a manifestation of cytotoxic edema in patients with traumatic injury. As with stroke, the precise underlying cellular events remain unclear. Cytotoxic edema and its associated effect on diffusion most commonly occurs in traumatic cortical contusion, but may also be a feature of acute TAI and of intracranial hematoma. Impact to the head, with or without consequent fracture, may cause contusion of the brain surface subjacent to the impact site, which is termed coup contusion. Subsequent acceleration of the brain and its impact against the skull opposite the site of the head impact leads to a secondary, typically more extensive, contusion. This secondary contusion is termed contrecoup contusion. Importantly, contusion also occurs in the absence of direct impact to the head. Acceleration of the head, as in a whiplash-type mechanism, can produce substantial acceleration of the brain, which subsequently impacts the skull, with potential for consequent cortical contusion. Contusion initially results in cytotoxic edema, which characteristically affects a contiguous region of brain tissue, affecting both superficial cortical gray matter and subjacent white matter, a pattern similar to ischemic injury. Unlike infarct, however, contusional injury does not typically follow a vascular distribution (▶ Fig. 11.2). Concurrent vascular injury or ischemia secondary to mass effect, however, may complicate the imaging appearance of contusion on DWI. Fig. 11.2 Contusion and traumatic axonal injury (TAI) due to nonaccidental injury. This 2-year-old boy was brought to the emergency department with multiple injuries and lethargy. Computed tomography was entirely unremarkable. (a) Susceptibility-weighted magnetic resonance imaging (SWI) showed hemorrhage confined to the splenium. (b) Hyperintensity is present within the splenium on T2-weighted fluid-attenuated inversion recovery imaging. (c) More extensive high signal on diffusion weighted imaging co-located with (d) low apparent diffusion coefficient indicates cytotoxic edema due to acute TAI, later determined to be a result of severe shaking. The earliest imaging manifestation of traumatic cortical contusion is restriction of isotropic diffusion. This appears as high signal on isotropic DWI and low apparent diffusion coefficient (ADC), seen on ADC images (see ▶ Fig. 11.2). Restricted diffusion in cortical contusion can be detected before CT or MRI reveal imaging evidence of tissue injury. However, DWI evidence of contusion is commonly identified in conjunction with signs of extracranial as well as intracranial injury, including scalp hematoma, skull fracture, hemorrhage, and edema (▶ Fig. 11.3). In this regard, location of the diffusion abnormalities relative to other signs of injury is key to correct diagnosis. DWI findings of contusion follow the classical coup contrecoup distribution already provided. Typically, the extent of injury opposite the site of impact (contrecoup) is greater relative to that immediately subjacent to the site of impact (coup). Fig. 11.3 Full extent of hemorrhagic contusion revealed by diffusion weighted imaging (DWI). (a) T2-weighted fluid attenuated inversion recovery, (b) diffusion weighted imaging and (c) apparent diffusion coefficient map shows heterogeneous signal due to hemorrhage, with areas of T2 shine-through (c) and diffusion restriction in the left frontal lobe. Note the additional area of diffusion restriction (white arrows; high signal on DWI (e) and (f) low apparent diffusion coefficient indicating tissue injury due to contusion beyond the area of hemorrhage. This area appeared relatively normal on computed tomography (not shown) and exhibited only minimal signal hyperintensity on (d) T2-weighted fluid attenuated inversion recovery. Restriction of isotropic diffusion is a nonspecific feature of many forms of brain tissue injury, including ischemia, infection, and inflammation (▶ Fig. 11.4). This lack of specificity, however, is typical of imaging findings in general and does not preclude the diagnostic utility of DWI in TBI. Additional features, when considered in conjunction with the presence of low ADC, are useful in arriving at a correct diagnosis of traumatic injury. The spatial distribution of DWI abnormalities will occur in typical locations and follow expected patterns of contusion or TAI. Anterior and inferior frontal and temporal lobe location and a coup contrecoup distribution of diffusion restriction, association with findings of extracranial soft tissue injury, and skull fracture are typical findings in contusion. The absence of conformity to arterial vascular distributions provides further supporting evidence that cortical diffusion restriction is due to traumatic contusion. Clear conformance of diffusion restriction to an arterial vascular distribution, on the other hand, is typical of stroke. Fig. 11.4 Differentiating diffusion restriction in multiple sclerosis from traumatic axonal injury (TAI). Axial T2-weighted fluid attenuated inversion recovery -FLAIR (a), diffusion weighted imaging (b) and apparent coefficients map (c). Diffusion restriction involves the large lesion adjacent to the ventricle (arrows in a, b and c) that can be differentiated from TAI by its location and association with other findings of multiple sclerosis (arrowheads in a). Focal diffusion restriction involving the white matter may be a manifestation of acute TAI (▶ Fig. 11.5, ▶ Fig. 11.6). Other disorders, such as brain abscess and multiple sclerosis (MS), also manifest focal diffusion restriction. Predilection for involvement of the corpus callosum, especially the splenium, deep white matter structures, and the gray matter–white matter junction are typical features of TBI. Concurrent identification of additional imaging features is also helpful in narrowing the differential diagnosis. Useful features might include white matter hyperintensities typical of MS, other imaging features of abscess, or additional findings of TBI, such as hemorrhage and extracranial injury. Fig. 11.5 (a) Computed tomography shows splenial hemorrhage typical of traumatic axonal injury (TAI) as well as diffuse edema, in a patient who was comatose following a high-speed motor vehicle accident. (b) Diffusion restriction due to TAI, however, is seen to involve the entire splenium, evidenced by high signal on diffusion weighted imaging and (c) low apparent diffusion coefficient. The additional area of diffusion restriction in the right frontal lobe represents cytotoxic edema due to surgical placement of an intracranial pressure monitor. (Images courtesy of: George Lantos, M.D., Jacobi Medical Center, Bronx, NY.) Fig. 11.6 In the same patient as in ▶ Fig. 11.4, (a) computed tomography at a more craniad level shows only diffuse edema. (b) High signal on diffusion weighted imaging and (c) low apparent diffusion coefficient delineate extensive traumatic axonal injury involving the corpus callosum and parasagittal gray–white junction bilaterally. (Images courtesy of: George Lantos, M.D., Jacobi Medical Center, Bronx, NY.) Clinical context is an essential factor in every differential diagnosis. History and other clinical evidence of TBI as well as the absence of known alternate diagnoses or their risk factors, including MS, cerebrovascular disease, or systemic infection, are necessary to support the correct attribution of DWI findings to TBI. Differential diagnosis for intra-axial isotropic diffusion restriction Contusion and traumatic axonal injury Stroke Cerebritis and abscess Demyelination Radiation injury Surgery Hematoma Seizures and postictal changes DWI detects decline in the magnitude (ADC; direction-independent net spatial displacement) of water self-diffusion in tissue. Lower magnitude (high signal on DWI and low ADC; i.e., diffusion restriction) is a manifestation of cytotoxic edema (▶ Fig. 11.7). DTI, however, uniquely indexes diffusion direction. As explained in Chapter 1, DTI provides two directional characteristics of water diffusion in tissue: the dominant direction of water diffusion (principle eigenvector) and the coherence (i.e., directional homogeneity, indexed by radial diffusivity [RD] and fractional anisotropy [FA]) of diffusion within the voxel. The normally high degree of anisotropic (directionally coherent) diffusion in normal white matter is conferred by its highly ordered microstructural environment, which includes multilayered parallel barriers to diffusion. These barriers include elements of the cytoskeleton, the axolemma and myelin sheath. As already described, one or more of these white matter constituents is affected and altered in TAI. The consequent loss of barriers to diffusion leads to less coherence of diffusion direction across the voxel, which manifests as lower FA. Higher RD may be the predominant factor driving increases in FA and may be a better predictor of adverse functional consequences of TAI. Loss of barriers to diffusion also facilitates an increase in the overall magnitude of diffusion (mean diffusivity [MD]), which has been repeatedly reported as a feature of TAI. FA, however, is the most widely studied and applied DTI parameter.17 Fig. 11.7 Cytotoxic edema in nonaccidental trauma. This 90-day-old boy was brought to the emergency department with lethargy and fussiness. Computed tomography (not shown) revealed skull fractures and a thin right convexity subdural collection. Edema was suspected, but no parenchymal brain abnormality was evident. (a, b) High signal on diffusion weighted imaging with (c, d) co-located low apparent diffusion coefficient delineates extensive right parietooccipital contusion (arrowheads) as well as traumatic axonal injury involving the splenium and deep right parietal white matter (arrows). Note that the region of cortical contusion crosses the middle cerebral artery -posterior cerebral artery border zone.
11.2 Clinical Context and Diagnostic Criteria
11.3 Pathology and Pathophysiology: Focal and Diffuse Injury
11.4 Diffusion Weighted Imaging—Sensitive Detection of Traumatic Tissue Injury
11.4.1 Pathophysiology of Diffusion Weighted Imaging Abnormalities in TBI
Detection of DWI abnormalities in TBI
Differential Diagnosis of DWI Abnormalities in Trauma Patients
11.5 Diffusion Tensor Imaging—Detection of Microstructural Traumatic Axonal Injury
11.5.1 Biophysical basis of DTI Abnormalities in TBI
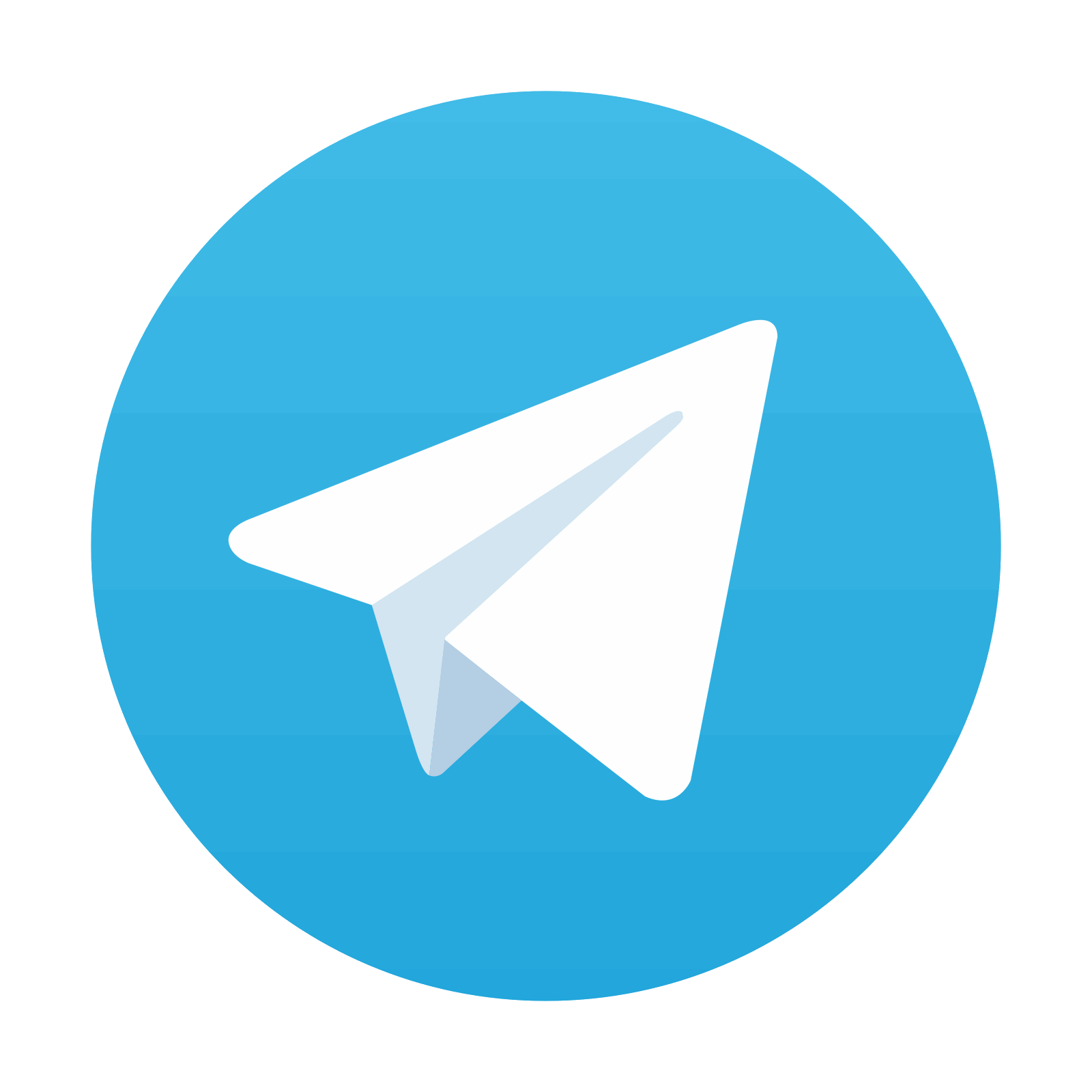
Stay updated, free articles. Join our Telegram channel

Full access? Get Clinical Tree
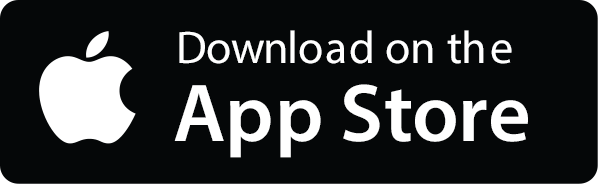
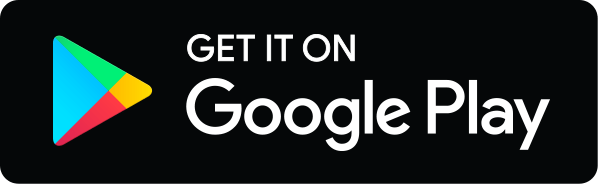