Diffusion based imaging strategies is sensitive to more subtle age-related changes in white matter integrity, and these methods may enable the differentiation of normal adult aging from mild cognitive impairment and Alzheimer dementia.
White matter indices are more sensitive to subtle changes than anatomical volumetric analyses examining atrophy in a variety of dementias.
Cognitive performance in specific domains, such as memory and executive function, corresponds with many diffusion tensor imaging (DTI)-based indicators within specific functionalized regions of the brain during aging.
Specific patterns of white matter degeneration may be discerned on DTI to distinguish between dementias.
5.1 Physics and Techniques
Both diffusion weighted imaging (DWI) and its related diffusion tensor imaging (DTI) rely on the premise of water diffusion changes as an indirect indicator of underlying brain parenchyma. Understanding the basis of diffusion methods and their limitations as assessments of surrogate measures of white matter tract and axonal integrity is critical for applying diffusion imaging methods clinically to the aging brain. These techniques are summarized in (▶ Table 5.1).
Imaging modality | Properties |
Diffusion weighted imaging (DWI) | Examines the ability of water to diffuse freely within tissues Diffusion restriction may indicate gross pathology within tissue |
Diffusion tensor imaging (DTI) | Examines the orientation of water diffusion by measuring diffusion in multiple directions, forming a vector sensitive to changes in brain microstructure Surrogate marker of white matter integrity Fiber tractography methods can reconstruct white matter tracts |
5.1.1 Diffusion Weighted Imaging
DWI uses a magnetic resonance sequence using two b values (based on magnetic gradient strength and pulse timing) to observe the motion of water molecules. If water molecules migrate during the interval between these gradients, then signal loss will occur. Such signal loss represents uninhibited random diffusion of water molecules representing isotropic diffusion. Diffusion restriction represents constriction of water molecules preventing random motion between gradient pulses and presenting as hyperintense signal on DWI. The apparent diffusion coefficient (ADC) is the quantitative index that separates the T2 shine-through effect from diffusion weighted images.
5.1.2 Diffusion Tensor Imaging
Because molecular water mobility may not be the same in all directions, DTI employs gradients in multiple noncollinear directions in order to quantify diffusion measurements in creating a three-dimensional ellipsoid representing the diffusion vector within a given voxel. In white matter tracts, water is observed to preferentially (nonrandomly) migrate in the direction of axonal fibers in an anisotropic manner. The extent to which white matter tracts are maintained indirectly influences water diffusion and therefore the measurement of ADC along various axes. DTI selectively highlights densely packed fibers with a highly ordered orientation parallel to each other.
The methods used to analyze DTI data are shown in (▶ Fig. 5.1) and are explored in greater technical detail in Chapter 1. Approaches range from region-of-interest methods that examine specific areas of the brain independently to whole brain voxel-based analyses. Tract-based spatial statistics (TBSS) is one means of delineating individual white matter tracts. Deterministic tractography methods are common techniques that examine the most likely white matter structure originating from a selected seed point, whereas probabilistic tractography estimates white matter trajectories for each voxel. These and other newer analytic techniques are being applied to the study of the aging brain. One newer approach, fiber density mapping (FDM), mitigates a key limitation of the aforementioned diffusion analyses, which only examine anisotropy measures for each voxel on an individual basis without the context of surrounding fiber patterns. FDM enhances analysis by examining the surrounding white matter fibers within the axonal bundle passing through a selected voxel.1
Fig. 5.1 Representations of diffusion tensor imaging data. Multiple methods are available for examining the multidirectional data acquired by diffusion tensor imaging. A region of interest (ROI) analysis method examines individual selected regions. Voxel-based morphometry (VBM) uses group-normalized data to quantitatively analyze white matter properties across the brain. Tract-based spatial statistics (TBSS) employs DTI information to generate putative representations of white matter tracts. Fiber tractography elucidates white matter pathways. (Reproduced with permission from Madden et al, 12 copyright Elsevier 2009.)
DTI-Based Measurements
No single characteristic of white matter integrity is directly measured by an individual DTI measure; therefore, multiple DTI-based variables offer insight into multifactorial aspects of white matter physiology. These quantitative calculations provide orientation-independent information on brain architecture and white matter integrity on the basis of a diffusion tensor mathematical matrix construct. The following values (summarized in ▶ Table 5.2) arise from calculations of the three eigenvectors that constitute the ellipsoid diffusion tensor.
Diffusion tensor imaging measure | Quantification | Details |
Fractional anisotropy (FA) |
| – Calculation involving the diffusivity differences – Values of 0 represent a spherical tensor and 1, an ellipsoid tensor – Provides information on microstructure integrity – Regarded as most robust DTI statistic |
Mean diffusivity (MD) | (λ1 + λ2 + λ3) / 3 | – Average of eigenvector diffusivities – Provides information on microstructure integrity |
Radial diffusivity (RD) – Perpendicular diffusivity |
| – Diffusivity perpendicular to the primary eigenvector – Primary contributor to FA – Reflects myelin integrity |
Axial diffusivity (AD) – Parallel diffusivity – Longitudinal diffusivity | λ1 | – Diffusion parallel to the primary eigenvector – Influenced by axonal diameter and number |
Radial diffusivity (RD) or perpendicular diffusivity (λ|) calculated from the diffusivity perpendicular to the first eigenvector is thought to represent a proxy for myelin integrity because water diffusion across axons would be easier with breakdown of normal myelin structures. RD is generally thought to represent a greater contributor to FA changes.
Fractional anisotropy (FA) is an index measuring intravoxel coherence (essentially, the normalized standard deviation of the three diffusivities) where the value ranges from isotropic (0), a spherical vector, to anisotropic (1), an ellipsoid vector. The FA reflects microstructure integrity.
Mean diffusivity (MD) provides information of the overall diffusion as the average of all three axes of the tensor (eigenvalues) within a selected volume.
Axial diffusivity (AD) or parallel diffusivity (λ//) measured from the diffusivity parallel to the first eigenvector, on the other hand, is inferred to indicate axonal integrity as a measure of fiber diameter and organization.
Of note, ADC can be viewed as an average of these two diffusivity values, RD and AD.
5.2 Clinical Applications
DTI provides detailed anatomical information about the status and directionality of white matter tracts. Because integrated coordination of neural activity across distant brain regions is essential to cognition, ascertaining axonal integrity could provide insight into the mechanisms by which cognitive functions are disrupted. Elucidating the basis of cognitive decline is an important problem in the clinical assessment of elderly individuals, which is becoming of pressing concern in most developed countries where aging demographics are generating large burdens on health care and the economy. Determining whether worsening reaction time or memory is related to mild cognitive impairment or “old age” versus a more debilitating dementia, such as Alzheimer disease, provides important information for patients and their families and can guide appropriate treatment strategies. Presently, the clinical definitions of cognitive aging and impairment are varied (▶ Fig. 5.3) and may not correspond well with objective measures. DTI may better assess the degree of neurodegeneration as a surrogate measure of white matter architectural integrity that correlates with pathology. Although not a functional imaging modality, the white matter pathways described by DTI serve as the conduits by which activity in brain networks occurs and may provide some information on functional pathways.2 Therefore, changes in DTI mirror alterations in functional networks that may be responsible for cognitive decline in aging and dementia.
Clinical manifestation | |
Cognitively normal aging | Optimal aging without manifestations of memory loss or cognitive impairment on neuropsychological testing |
Normal aging | Generally thought to include self-perceived memory loss without cognitive deficits relative to peers, but may include poorer performance relative to younger cohorts |
Mild cognitive impairment (MCI) | An ambiguous definition that includes individuals thought to perform between normal aging and dementia; generally regarded as a transitional period prior to dementia |
Amnestic mild cognitive impairment (aMCI) | Subclassification of MCI where individuals demonstrate prominent memory impairment symptoms; these individuals are at greatest risk of Alzheimer disease out of the general MCI population |
Dementia | Generic classification for cognitive impairment that interferes with daily functioning; includes cognitive impairments in addition to memory deficits |
Alzheimer disease | Most common form of dementia with hallmark neurodegenerative changes definitively diagnosed on autopsy, although frequently diagnosed clinically; targeted amyloid positron emission tomographic imaging is presently available for presumptive diagnosis |
Source: Adapted from Small GW, Bookheimer SY, Thompson PM, et al. Current and future uses of neuroimaging for cognitively impaired patients. Lancet neurology. 2008;7(2):161-172. doi:10.1016/S1474-4422(08)70019-X. |
The application of DTI clinically to the aging patient with cognitive decline or symptoms of dementia remains difficult. Nevertheless, there have been significant advances in the past decade that facilitate the use of DTI to answer clinical questions of differentiating between types of dementia. Most of the earlier studies that provide the fundamental basis for what is known about DTI changes in specific dementia types are based on the comparison of multiple individuals with dementia versus controls using groupwise analysis methods. More recent studies offer evidence to support the use of single-subject DTI studies to diagnose individual patients with neurodegenerative dementias.3
5.2.1 Normal Aging
Because white matter volume does not appear to decrease as much as gray matter volumes with aging, volumetric magnetic resonance imaging (MRI) analyses of white matter loss are inadequate to assess the aging brain.4 Likewise, white matter hyperintense lesion burden does not appear to consistently account for cognitive decline. DTI addresses this limitation by ascertaining the finer detail of subtle changes within axonal structures. As discussed in Chapter 4, normal white matter development leads to MD reduction and FA increase over normal development to middle age, which corresponds to axonal development and myelination with greater time spent maturing frontal–temporal connections.5 In later age, these developmental changes reverse, with decreasing anisotropy and increasing diffusivity values globally. Both abnormal and normal adult aging may lead to significant decline in white matter organization.6 While aging changes appear global and symmetric, some have shown the greatest FA decline within the corpus callosum, frontal, limbic, and temporal lobes, although these same regions have the highest anisotropy initially.1,6 More specifically, most literature supports an anterior-predominant gradient of FA decline and diffusivity increase (MD, AD, and RD) with age.7 ▶ Fig. 5.2 demonstrates the normal patterns of aging-related FA and MD changes across several brain regions. Furthermore, application of Fiber density mapping (FDM) to the aging brain reinforces these findings, which were observed to occur globally within white matter to a greater extent within anterior versus posterior regions.1 Thus it appears that frontal regions are both the latest to develop in maturation and likewise the most susceptible to functional decline in the later years of life, which also concurs with clinical findings of executive (frontal) dysfunction.8
Fig. 5.2 Plots of fractional anisotropy (FA) and mean diffusivity changes with age. (a) The corpus callosum is a vital component of white matter architecture for cognitive function and degeneration is implicated in worsening cognitive measures including reaction time. FA peaks in adulthood and decreases with aging, to a greater extent within the anterior portions. (continued)
(continued) (b) Association fibers are integral to the organization of neural activity, and white matter integrity within these fibers preserves higher-order cognition. As in the corpus callosum, FA peaks and mean diffusivity (MD) troughs occur in early adulthood. FA decreases and MD increases with age are slightly greater within the frontal association fibers. (Reproduced with permission from Lebel et al,5 copyright Elsevier Ltd, 2012.)
Pathophysiology of DTI Changes in Aging
With aging, myelin layers continue to be added with redundant myelin, and this myelin may be formed by oligodendrocytes in a manner making it vulnerable to healthy myelin formed by oligodendrocytes earlier in life. Myelin loss with both declining number and length of myelinated axonal fibers and demyelination are implicated in FA loss and increased diffusivity with increased extracellular space (▶ Fig. 5.3). Another mechanism by which increased diffusion may occur with aging is via splitting of myelin and cavitation within myelin sheaths, thereby also producing increased extracellular space encouraging free diffusion.8
Fig. 5.3 Comparison of normal axons and aging axons. Normal axon integrity exhibits a dense, rich microstructure with normal myelination and little extracellular space. In comparison, the aging brain demonstrates axonal loss and dysmyelination that results in thinning of the myelin sheath and increased extracellular space. The increase in extracellular space and the decreased hindrance of diffusion presented by decreased axonal size and number results in increased diffusivity with age.
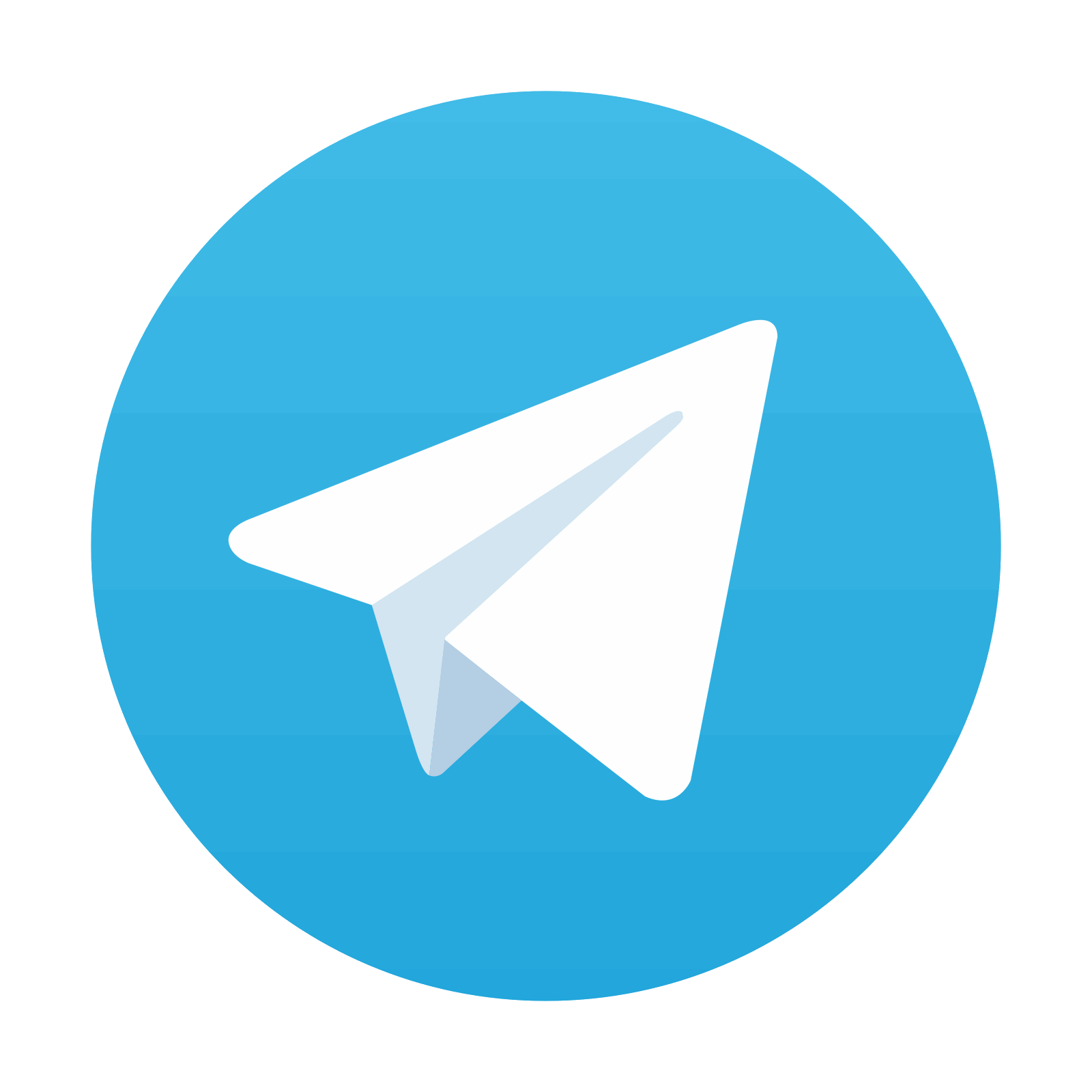
Stay updated, free articles. Join our Telegram channel

Full access? Get Clinical Tree
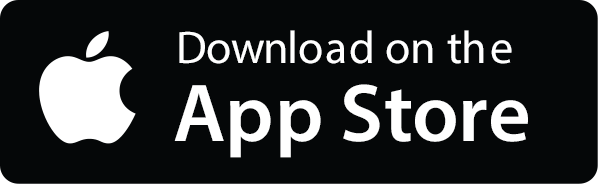
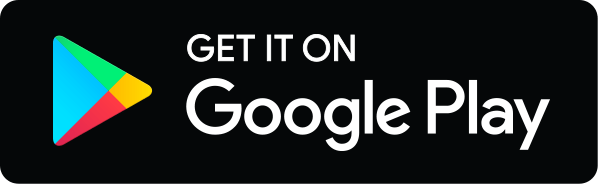