Diffusion weighted imaging (DWI) helps differentiate solid from cystic lesions and benign from malignant head and neck lesions.
The DWI imaging findings should always be correlated with the morphological anatomical images, and interpreting the findings requires experience and knowledge.
Some of the indications for DWI are tissue characterization for primary tumors and nodal metastases, prediction and monitoring of treatment response, differentiation of recurrent tumor from posttherapeutic changes, evaluation of inflammatory and infectious lesions, and study of salivary and thyroid glands.
Generally, malignant tumors show greater restriction of diffusion and a lower apparent diffusion coefficient (ADC) compared to normal tissues and benign tumors.
Lymphomas tend to have some of the lowest ADCs of all tumors in the head and neck.
Exceptions occur, such as nasopharyngeal adenoid hypertrophy, which has greater restriction of diffusion and a lower ADC, similar to malignant tumors, probably due to the high cellularity.
Other exceptions are classic chordomas and low-grade chondrosarcomas, which show high ADC values.
One of the most important indications for DWI in the head and neck is the evaluation of cholesteatomas, especially in their postoperative follow-up; they are hyperintense on DWI.
14.1 Introduction
The addition of diffusion weighted imaging (DWI) to magnetic resonance imaging (MRI) protocols in the evaluation of head and neck malignancies increases lesion detection and helps with differentiating solid from cystic lesions and benign from malignant lesions. Both the DWI and apparent diffusion coefficient (ADC) maps are valuable for assessing various head and neck pathological conditions; however, the DWI findings should always be correlated with the anatomical images, and good collaboration between radiologists, physicists, and clinicians is a prerequisite for optimal patient management.1 The recent studies of diffusion in the head and neck suffer from small samples, susceptibility artifacts associated with single-shot echo-planar image (EPI) sequences, and low resolution of diffusion images and ADC maps. Because of these issues, DWI characteristics and ADC values should not be used in isolation because there may be overlap between different tumor types.2 Correct interpretation of DWI requires experience and knowledge.3
Optimization and standardization of DWI technical parameters, comparison of DWI with morphological images, and increasing experience are prerequisites for successful application of this challenging technique to head and neck lesions. For this anatomical region, indications for DWI include, but are not limited to, tissue characterization for primary tumors and nodal metastases, prediction and monitoring of treatment response, differentiation of recurrent tumor from posttherapeutic changes, evaluation of inflammatory and infectious lesions, and study of salivary and thyroid glands.1
In general, malignant tumors tend to show greater restriction of diffusion and, hence, a lower ADC when compared with normal tissues and benign tumors. Lymphomas tend to have some of the lowest ADCs of all tumors in the head and neck. Furthermore, cancers that show only small changes (increase or decrease) in ADC during treatment or in the early posttreatment phase are more likely to fail treatment. For tumors in general, variations in ADC may arise from heterogeneity in cell size and density, as well as microscopic hemorrhage or calcification.
Future improvement of methods to analyze ADC maps, including histogram analysis and functional maps, should advance the applications of DWI in the head and neck, especially for treatment monitoring. Recently, several attempts have been made to determine perfusion and diffusion parameters separately by using intravoxel incoherent motion MRI (IVIM-MRI). Perfusion is an important marker of many physiological and pathological processes and can be used as a predictive indicator for effectiveness of chemoradiation and radiotherapy. Therefore, analyzing DWI and perfusion may be helpful in the preoperative tumor evaluation. A simplified IVIM-MRI technique based on the IVIM model has been used to assess perfusion-related parameters (PPs) and molecular diffusion (D) of head and neck tumors, which can be determined with three or four b values (0, 500 and 1,000 s/mm2; or 0, 200, 400 and 800 s/mm2). This technique is both fast and easy to apply compared with IVIM-MRI using multiple b values (e.g., 0, 10, 20, 30, 50, 80, 100, 200, 300, 400, and 800 s/mm2). The time–signal intensity curve (TIC) profiles obtained after dynamic contrast-enhanced (DCE) MRI represent tumor tissue perfusion properties. Therefore, the use of any single technique may not be effective in the differentiation of head and neck tumors. IVIM-MRI is a technique with the potential for simultaneously assessing both tissue perfusion and diffusion. This multiparametric approach using a TIC and IVIM criteria can help in the differentiation between benign and malignant head and neck tumors and between different histological tumor types with high levels of accuracy.4
This chapter emphasizes the main indications for DWI in the differentiation of tumors and inflammatory processes (▶ Table 14.1). The chapter closes with some comments about diffusion tensor imaging (DTI) applications in the head and neck.
Lesions of the head and neck with low ADC | Lesions of the head and neck with high ADC |
Lymphoma | Pleomorphic adenoma |
Squamous cell carcinoma | Inflammatory process |
Soft tissue sarcoma | Chordoma |
Metastatic lymph node | Low-grade chondrosarcoma |
Warthin tumor | Low-grade glioma (optic) |
Rhabdomyosarcoma | Vascular lesion |
Primitive neuroectodermal tumor | Hemangioma |
Eosinophilic granuloma | Cholesterol granuloma |
Inflammatory process | |
Fungal lesions | |
Abscess | |
Hemorrhage following recent surgery | |
Cerumen in the external ear canal | |
Meningioma | |
Fibrous histiocytoma | |
Cholesteatoma | |
Dermoid cyst | |
Nasopharyngeal adenoid hypertrophy |
14.2 Tumors
14.2.1 Squamous Cell Carcinoma
Squamous cell carcinoma (SCC) is the most prevalent tumor of the head and neck region, and MRI has aided in lesion detection and characterization, particularly for areas that are difficult to access during physical examination, such as the skull base, nasopharynx, palatine and lingual tonsils, laryngeal cartilages, and hypopharynx. When a patient presents with a clinical suspicion of a malignancy in the head and neck, the first step is to confirm the diagnosis with imaging. If the diagnosis is confirmed, the second step is to assess the disease extent, regional spread, and metastases. The third step is to supply specific information about the tumor nature, trying to identify which tumors will be more responsive to conventional chemoradiation. This step is important to select which cancers will need other therapies, such as surgery or different radiation types. DWI is the most important and established technique for the third step.2
Beyond the structural images, DWI is a functional technique used mostly for detection and characterization of head and neck tumors. Restriction of water diffusion is observed in malignant tumors that present low ADC values, due to high cellularity, dense cellular populations, and/or a high nucleocytoplasmic ratio. Necrosis and nonmalignant changes, such as fibrosis and inflammation, have less cellularity compared to viable tumor and hence show high ADC values3 (▶ Fig. 14.1).
Fig. 14.1 Squamous cell carcinoma of the nasopharynx invading the retropharyngeal, paraspinal, parapharyngeal, and masticator left spaces. The lesion shows (a) heterogeneous signal on the b 1,000 diffusion weighted imaging, (b) middle intensity signal on the apparent diffusion coefficient map, and (c) diffuse gadolinium enhancement.
There are several studies emphasizing the importance of DWI in the evaluation and differentiation of tumors in the head and neck.5,6,7 Wang et al6 and Maeda et al7 reported that it is possible to differentiate tumors applying the mean ADC value, showing that benign lesions have higher ADC values, lymphomas have lower values, and carcinomas had intermediate values. Wang et al determined a threshold ADC value of 1.22 × 10–3 mm2/s, with high accuracy (86%), sensitivity (84%), and specificity (91%) to predict malignancy.6 Other authors have presented different ADC values for malignant tumors of the head and neck, with lesser values for lymphomas, intermediate values for SCC, and greater ADC values for malignant salivary gland tumors.2 Other capacity attributed to DWI is to distinguish between well-differentiated and poorly differentiated SCCs by virtue of their ADC values. The most differentiated tumors have higher ADC values, whereas poorly differentiated tumors have lower ADC values.2,6,8 This difference is due to the higher concentration of cytoplasmic macromolecules, the higher nucleus–cytoplasm relationship, increased cellularity, and the presence of necrotic foci in undifferentiated neoplastic tissues.
On the other hand, a recent retrospective study by Surov et al found that the ADC values of nasopharyngeal adenoid hypertrophy (NAH) were as low those previously reported in carcinomas of the head and neck region, both in children and adults, a finding that was attributed to the NAH high cellularity. Because of this behavior, NAH can be misinterpreted as carcinoma or malignant lymphoma, especially in patients over 40 years of age.9
A recent review of the literature on DWI in the head and neck demonstrates that it shows no added value in the detection of primary epidermoid carcinoma, but it may show potential value in nodal staging and discrimination of recurrence from posttreatment changes.10
MRI has good diagnostic performance in the overall pretreatment evaluation of node staging in patients with SCC. A limited number of small studies suggest that DWI has a higher accuracy as compared with turbo spin-echo (TSE) MRI for the detection of nodal metastases. DWI can be complementary to conventional MRI because size-related and morphological criteria lack sufficient reliability for the identification of small nodal metastases in patients with SCC. Malignant lymph nodes have a lower ADC than benign lymph nodes, and DWI is most beneficial in the detection of subcentimeter metastatic lymph nodes.11,12
Oral inspection, laryngoscopy under general anesthesia, conventional image analysis of computed tomography (CT) and MRI can also be impaired by actinic changes, such as local edema, inflammation, necrosis, fibrosis, mucosal thickening, and diffuse contrast enhancement, limiting the identification of residual or recurrent tumors or suggesting false-positive diagnoses, which may result in unnecessary procedures. Moreover, some residual or recurrent tumors may not show increased contrast enhancement, further hindering the identification of disease.13
The need for early diagnosis of cancer recurrence is essential, so MRI techniques have been developed to increase the sensitivity and specificity for its detection. Diffusion imaging sequences have also been used to distinguish recurrent tumor from postoperative and postradition changes.14 The b = 0 signal intensity is lower in malignant lesions than in benign tissues with postradiation changes. The b = 1,000 signal intensity is higher in malignancy than in nonneoplastic tissues. Consequently, ADC values are significantly lower in cancer. These characteristics hold for before, during, or after the chemoradiation therapy between inflammatory, fibrotic, and persistent tumor.14 Some authors demonstrated that cellular death and vascular changes by therapy could be detected by DWI before a reduction of lesion diameter became apparent by conventional images. DWI allows monitoring of tumor response during radiotherapy because loss of tumor cells and increased free water in tissues result in increased ADC values14 (▶ Fig. 14.2).
Fig. 14.2 The same patient as in ▶ Fig. 14.1, with nasopharynx squamous cell carcinoma after 6 months of chemoradiation, presented (a) a reduction in the size of the lesion and signal reduction on the b 1,000 diffusion weighted imaging, (b) absence of reduced apparent diffusion coefficient, and (c) no gadolinium enhancement. The findings suggest treatment response with significant reduction in cellularity of the lesion.
DWI helps to distinguish responders from nonresponders, as well as early identification of nonresponding tumors. Radio resistance and poor outcomes during treatment are determined by lower cellularity and necrosis mediated by hypoxia. Radio sensitivity is determined by solid and hypercellular tissues with low pretreatment ADC values.14 Early studies show that changes in ADC values may occur before morphological changes, such as tumor dimension, volume or contrast enhancement, mainly in the first 3 months posttreatment. An increase in ADC during treatment is associated with higher survival rates15 (▶ Fig. 14.3 and ▶ Fig. 14.4). DWI can detect nonresponders and can lead to stopping ineffective treatments and avoiding delays in the administration of other, potentially more effective, treatment alternatives. Thus DWI has increased sensitivity and accuracy to detect recurrence and in the differential diagnosis in the posttreatment setting, providing better results than CT and fludeoxyglucose positron emission tomography (FDG-PET), including fewer false-positive results in persistent primary sites and in metastatic lymph nodes.15
Fig. 14.3 The same patient as in ▶ Fig. 14.1 and ▶ Fig. 14.2 with nasopharynx squamous cell carcinoma after 42 months of chemoradiation shows (a) significant reduction in the dimensions of the tumor and signal normalization on b 1,000 diffusion weighted imaging, (b) high signal on the apparent diffusion coefficient map, and (c) faint gadolinium enhancement. The results suggest complete response after treatment, with fibrous scarring at the site of the primary lesion.
Fig. 14.4 Left cervical level 2 lymph node metastases from the hypopharynx squamous cell carcinoma. (a) Axial enhanced computed tomography shows a heterogeneous node in contact with the left internal jugular vein. (b) After 4 months of chemoradiation a reduction in size and contrast enhancement of the lymph node are observed on the enhanced axial T1-weighted image. (c) There is intermediate signal intensity on the b 1,000 diffusion sequence. (d) There is high signal on the apparent diffusion coefficient map, which indicates response to treatment.
One technical advantage of DWI is the ability to acquire reproducible images in a short time period. One disadvantage is its magnetic susceptibility, especially that due to dental fillings. One potential solution to reduce susceptibility artifacts is acquisition time parallel imaging. The overlapping ADC values may be caused by partial volume effects in tiny structures and lowered ADC (false-positive) in fibrosis and higher ADC in necrotic parts of recurrent tumor (false-negative).13
Diffusion sequences should be used routinely in standard MRI studies as an adjunct to evaluated response of cancers to nonsurgical treatment.14
14.2.2 Lymphomas
Lymphomas are tumors with high cellularity and scarce stroma. The usual imaging analysis based on the characterization of lymph node morphology, size, and shape is not reliable to differentiate benign from malignant nodes. Molecular imaging is a noninvasive modality that has the potential of tissue characterization based on the results of DWI, spectroscopy, single-photon emission computed tomography (SPECT), and PET-CT.16 Even though ADC varies with b value, field strength, lesion size, and presence of necrosis, Maeda et al found that the mean ADC for lymphoma is consistent in 0.65 + − 0.09 × 10–3 mm2/s7 range. According to these authors an ADC of 0.76 × 10–3 mm2/s may be used to distinguish between SCC and lymphoma with an accuracy of 98%.7 Sumi et al found differences in ADC values in lymph nodes and benign lymphadenopathy; the latter had higher values, whereas lymphomas had the lowest values (▶ Fig. 14.5), and metastatic lymph nodes had values that were slightly higher than those in lymphomas.8
Fig. 14.5 B cell lymphoma. (a) Coronal T2-weighted imaging shows a hyperintense mass in the left cervical region. (b) Contrast enhanced T1-weighted imaging shows enhancement of the lesion. (c) The lesion is hyperintense on diffusion weighted imaging and (d) shows restricted diffusion on the apparent diffusion coefficient map.
Compared to PET-CT, which is considered the gold standard in the detection and monitoring of lymphoma, DWI has sensitivity and specificity of 90% and 94%, respectively, for this purpose.16 IVIM-MRI may improve the use of DWI and allow differentiation of benign from malignant head and neck tumors. According to Sumi et al the ADC values and perfusion-related parameter values of lymphomas were the smallest among all head and neck tumors17
14.2.3 Orbital Tumors
Orbital tumors are very heterogeneous, and it is sometimes a challenge to distinguish inflammatory lesions from neoplasms. Diffusion restriction is observed in high cellularity tumors; however, not all malignant lesions are hypercellular, and some benign tumors, such as meningioma, fibrous histiocytoma, and dermoid cyst, have restricted diffusion.18
According to Sepahdari et al the optimal ADC threshold for differentiating malignant from benign lesions is 1 × 10–3 mm2/s, and an ADC ratio of 1.2 results in a 63% sensitivity, 84% specificity, and 77% accuracy. The best results in malignant tumors are among T2-dark infiltrative lesions because of their high cellularity. DWI is excellent in differentiating lymphoma, which has a low ADC from inflammatory pseudotumor that has high ADC; however, there is an overlap between benign and malignant lesions, particularly in the intermediate ones19 (▶ Fig. 14.6). Hemangioma is composed of capillaries in a fibrous stroma. Some may grow rapidly and have a tendency to bleed. DWI shows low signal intensity, and their ADC value is higher compared to that of the brain (▶ Fig. 14.7).20
Fig. 14.6 Rhabdomyosarcoma. (a) Coronal T2-weighted imaging shows a hyperintense mass in the right orbit. (b) T1-weighted pre- and (c) postcontrast images show enhancement of the lesion. (d) The lesion is hyperintense on diffusion weighted imaging and (e) shows restricted diffusion on the apparent diffusion coefficient map.
Fig. 14.7 Capillary hemangioma. (a) Coronal T2-weighted imaging shows a hyperintense mass in the right eyelid. (b) Pre- and (c) postcontrast T1-weighted images show enhancement of the lesion. (d) Diffusion weighted imaging and (e) apparent diffusion coefficient map shows no restricted diffusion. (f) Magnetic resonance venography shows vessels communicating the hemangioma and superficial and deep venous drainage systems.
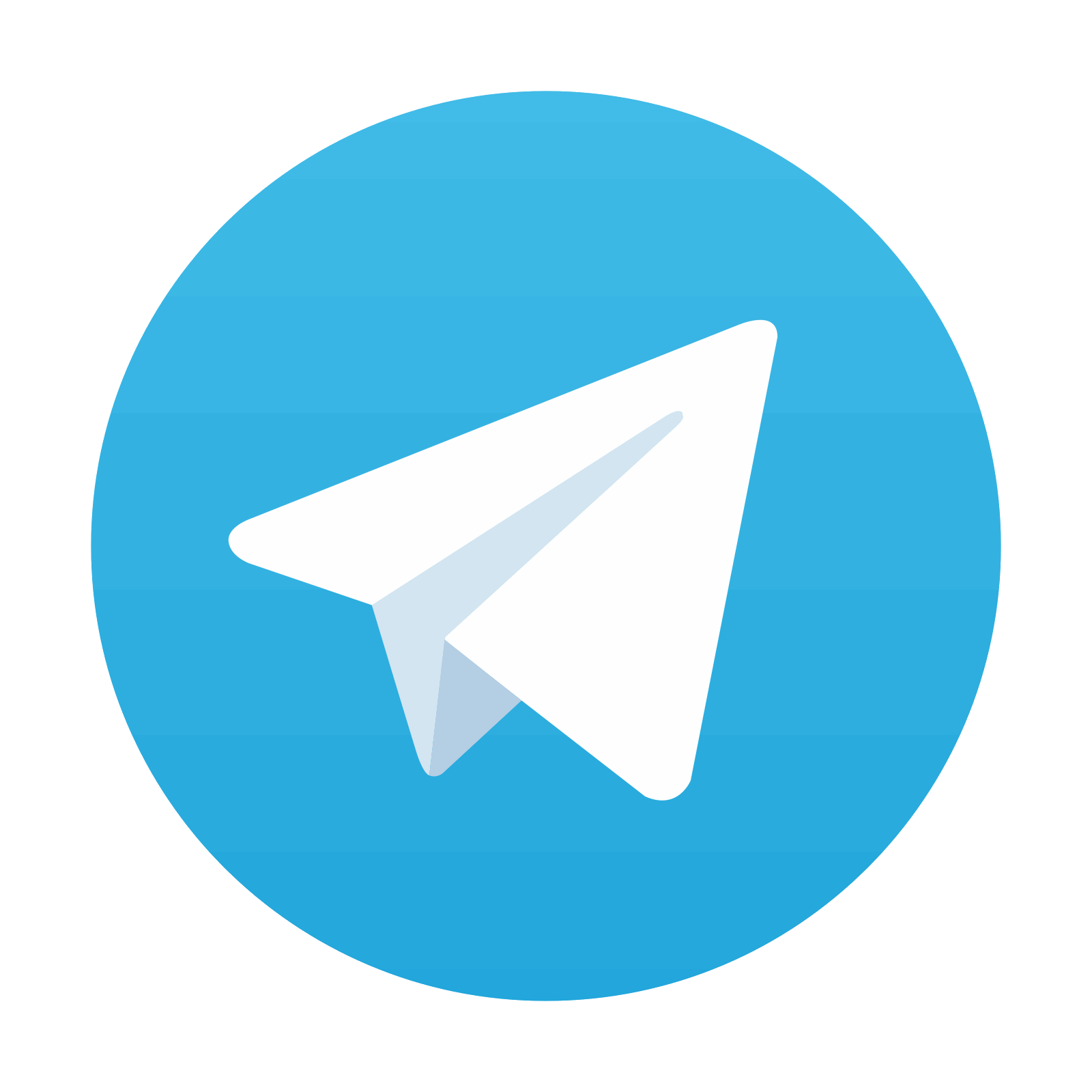
Stay updated, free articles. Join our Telegram channel

Full access? Get Clinical Tree
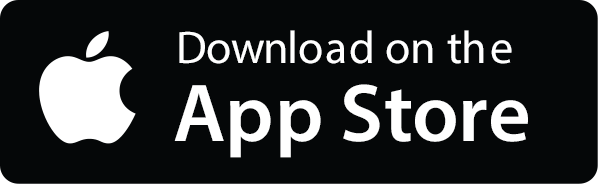
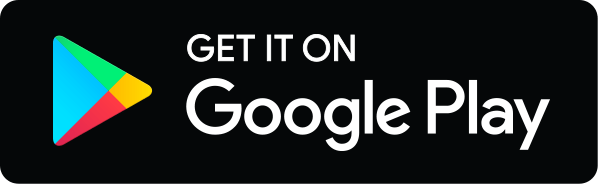