Diffusion weighted imaging (DWI) is the method of choice for the detection of spinal cord infarction.
Diffusion tensor imaging (DTI) can be helpful for differentiating ependymoma from astrocytoma in adults.
DTI is a promising technique for evaluating patients with spinal cord injury (detecting the injury epicenter, differentiating injured from normal spinal cord in the absence of T2-signal changes).
DWI is a useful tool for differentiating benign from malignant vertebral body fractures (when used with conventional sequences).
DWI can be a helpful tool for differentiating Modic I changes from acute spondylodiskitis.
13.1 Introduction
13.1.1 Technical Considerations
The role of diffusion weighted magnetic resonance imaging (DWI) in the evaluation of brain diseases was established shortly after its introduction. DWI became an irreplaceable part of the routine brain imaging protocol. Further developments with diffusion tensor magnetic resonance imaging (DTI) and fiber tractography (FT) have catapulted brain imaging to an even higher level.
Since the first published report on the usefulness of DWI in the differentiation between osteoporotic and malignant vertebral body fractures in 1998,1 physicists and clinicians continue to grapple with constructing clinically applicable and reproducible DWI and DTI sequences for the spine and spinal cord. Nevertheless, obtaining spinal cord DWI and DTI remains a challenge due to numerous technical difficulties. The size of the spinal cord, the macroscopic motion related to the surrounding cerebrospinal fluid (CSF) pulsations, breathing and swallowing movement artifacts, and local field inhomogeneities are the major technical issues. Technical solutions are needed to optimize both image acquisition and image processing. Studies published on DWI and DTI of the spine are limited and have mainly been restricted to the cervical spine.
Recently, fairly good technical solutions have been developed, such as multishot echo-planar imaging (EPI), diffusion weighted periodically rotated overlapping parallel lines with enhanced reconstruction (PROPELLER), spin-echo navigator spiral DTI, and parallel imaging. The acquisition times have been reduced, allowing implementation of DWI/DTI in routine clinical protocols. Through spatially selective excitation, zoomed DWI allows for acquisition of high-resolution images, with a reduced scan time, due to a reduced field-of-view (rFOV) in the phase-encoding direction.2 rFOV sequences3,4 have shown promise in producing high-quality, in vivo human spinal cord DTI. In one larger study of 223 patients, performed on a 1.5 T clinical scanner, an rFOV DWI sequence added clinical utility in 33% of cases where pathology was done.4 The rFOV DWI sequence was found to be helpful in the evaluation of acute infarction, demyelination, infection, neoplasm, and intradural and epidural collections.4 In another study, the diagnostic value of DWI and the measurement of the apparent diffusion coefficient (ADC) were evaluated in 33 patients who presented with a spinal cord syndrome due to a noncompressive myelopathy.5 ADC values measured in that study were significantly lower in spinal cord infarct (mean ADC 0.81 × 10–3 mm2/s) when compared with inflammatory spinal cord lesions (mean ADC 1.37 × 10–3 mm2/s) and with those measured in healthy control spinal cord (mean ADC 0.93 × 10–3 mm2/s).5
The usefulness of DWI and DTI of the spine has also been reported in children. In one study, five controls and five children with cervical spinal cord injury (SCI) were imaged by using a single-shot, echo-planar, diffusion weighted sequence. Children with SCI showed reduced fractional anisotropy (FA) and increased diffusion (D) values compared with controls. The study has shown that the differences in diffusion metrics between noninjured and injured spinal cords can be demonstrated in the pediatric population.6
13.2 Clinical Applications for DWI and DTI of the Spinal Cord
13.2.1 Spinal Cord Ischemia
Acute spinal cord ischemia is an uncommon condition that accounts for 5 to 8% of acute myelopathies. It is associated with severe functional neurological loss, and paraplegia in up to 33% of the cases.7 Aortic dissection, open thoracoabdominal aortic surgery (5–21% risk), mechanical trauma, and systemic hypotension are the most common causes of acute spinal cord ischemia.7 Rarely, a fibrocartilaginous embolism (FCE) with a progressive onset (over several hours) could be the cause.8 Linear T2-hyperintensity on sagittal magnetic resonance imaging (MRI) scans, and the appearance of “snake-eyes” on axial T2-weighted MRI in the cord, are typical MRI findings. The spinal cord will be swollen, and contrast enhancement will be present in the subacute stage. Although MRI is the method of choice in the detection of spinal cord ischemia, in a large number of cases, initial MRI will not show any abnormality.7
There have been only smaller series published that describe DWI findings in acute spinal cord ischemia.9,10,11,12,13,14 The ADC measured in one published series of spinal cord infarction ranged between 0.23 and 0.86 × 10–3 mm2/s.14 The shortest time reported in the literature between the onset of clinical symptoms and abnormalities shown on DWI was 3 hours after the onset of symptoms (▶ Fig. 13.1). In one small series of four children with anterior spinal artery infarction after minor trauma, DWI confirmed the clinical diagnosis in all cases15 and showed the feasibility of DWI in spinal cord ischemia in a pediatric population.
Fig. 13.1 Spinal cord ischemia in a 64-year-old woman who presented with acute back pain and paraplegia. (a) Sagittal T2-weighted image of the lumbar spine performed 4 hours after the onset of symptoms shows no abnormality. (b) Sagittal T2-weighted image 48 hours later demonstrates hyperintensity and swelling of the conus medullaris. (c) On the T2-weighted image, there is swelling of the anterior gray matter. (d) Diffusion weighted imaging shows high signal intensity as the result of restricted diffusion in the conus medullaris (with low apparent diffusion coefficient, not shown).
Clinical rationale for DWI/DTI in spinal cord ischemia:
Early detection of spinal cord infarction when conventional sequences are unremarkable
Detection of spinal cord infarction due to FCE in young athletes
13.2.2 Spinal Cord Tumors
The most common intramedullary tumors in adults are ependymomas, followed by astrocytomas, other gliomas, hemangioblastomas, and metastases. In the pediatric population, astrocytomas are diagnosed in a higher percentage of cases. Diagnostic challenges are the differentiation of ependymoma and astrocytoma, and the differentiation of resectable from nonresectable spinal cord tumors based on MRI findings. Ependymomas and hemangioblastomas are considered resectable, whereas astrocytomas, due to their infiltrative nature, are considered nonresectable. Therefore, the determination of resectability is essential. The value of DWI and DTI in predicting the resectability of intramedullary tumors has been shown in a recent study.16 Patients were classified according to the fiber course with respect to the lesion. The results were compared with the surgical findings (existence or absence of cleavage plane). Despite the low number of patients in that study (14 patients), the results showed good interrater reliability for DTI and intraoperative findings, and the presence or absence of a cleavage plane.16 Caution is necessary when DTI is used in the pediatric population. The pilocytic astrocytomas, that are common intramedullary neoplasms in children, show histological and structural differences from the infiltrating astrocytomas seen in adults. In one published series of 10 children with intramedullary neoplasms, preoperative DTI was performed to characterize the margins of tumors for surgical planning.17 In all pilocytic astrocytomas (seven cases) (▶ Fig. 13.2) and one ependymoma (▶ Fig. 13.3), splaying of fibers was found. The ganglioglioma and high-grade glioma showed evidence of infiltration of fibers.17 In another small series, displacement of the fibers was seen in three cases of ependymoma.18
Fig. 13.2 Surgery-proven ependymoma in a 39-year-old female patient (a) On the sagittal T2WI image of the cervical spine, a bulky mass is noted in the spinal cord at the Th1–2 level, with cord hyperintensity above and below the mass, representing edema. (b) The mass is T1-isointense to the spinal cord. (c) On the post-contrast T1WI image, strong enhancement is shown of the solid tumor part. (d) DT-FT Diffusion tensor-Fiber tractograpy shows centrally located mass with displacement of the fibers.
Fig. 13.3 Histology-proven the spinal cord astrocytoma (grade III) in a 38-year-old male patient who presented with pain in the thoracic region, sensory disturbances in the left lower extremity followed by left hemiparesis, and gait disorder. On spinal sagittal T2-weighted image (a) and Short tau inversion recovery (STIR) (b) hyperintensity and enlargement of the cord is detected from the C6 to the T10 level. (c) On enhanced T1-weighted image, an ill-defined enhancement is shown at the T3–6 level. (d) Fiber tractography shows diffuse infiltration of the fibers with no displacement.
Ducreaux et al described DTI findings in five cases of spinal cord astrocytoma (▶ Fig. 13.4).18,19 The data suggest that FT can be used for visualization of warped and destroyed fibers in cases of solid astrocytomas, but showed some limitations in cystic astrocytomas. Furthermore, when using DTI metrics, astrocytomas, ependymomas, and metastases showed similar FA values.18 The lowest FA values were measured in metastases and the highest in hemangioblastomas. Metastatic infiltration of nerve roots can also be detected by DTI (▶ Fig. 13.5).
Fig. 13.4 Pilocytic astrocytoma of the cervical spinal cord in a five-year-old boy. (a) Sagittal T2-weighted image shows high signal intensity mass in the cervical spinal cord extending from C2 to the T1 levels, with cord enlargement. The mass did not show enhancement (not shown). (b) Fiber tractography nicely demonstrates splaying of the fibers.
Fig. 13.5 Biopsy-proven metastatic neuropathy in a 44-year-old woman with breast carcinoma who presented with lumbar pain irradiating to the right side. (a) Thoracic spine coronal short tau inversion recovery (STIR) shows low signal linear abnormalities following the spinal roots over multiple segments. (b) Diffusion tensor imaging demonstrates asymmetry of the nerve roots with multiple thickened nerve roots on the right side. (c) Positron emission tomography/computed tomography confirmed metastatic disease affecting multiple thoracic nerve roots.
It is imperative to identify drop metastases in children with brain tumors. The presence of drop metastases is associated with a poor prognosis, so early detection is crucial to allow modification of the treatment. Recently, a case of drop metastasis was reported in which readout-segmented echo-planar imaging (EPI) was used to identify hypercellular metastases in a child with a primary CNS tumor.20
The differentiation between an intramedullary tumor and tumorlike lesions (TLLs) in the cervicomedullary junction region and cervical spinal cord can sometimes be challenging. A recently published series evaluated DTI and perfusion-weighted imaging (PWI) in 12 patients with intramedullary tumors and 13 with TLL in the cervicomedullary junction and cervical spinal cord. The mean FA value of tumors was found to be significantly lower when compared with TLL, and the mean trace ADC and peak height values of tumors were significantly higher.21
Clinical Rationale for DWI/DTI in Spinal Cord Tumors
Differentiation of ependymoma and astrocytoma in adults (not in children!) (splayed and destroyed fibers in ependymoma, diffuse involvement of fibers in astrocytoma)
Determination of resectability of spinal cord tumors
Identification of hypercellular drop metastases in children with central nervous system (CNS) neoplasms
Differentiation between intramedullary tumor and tumefactive demyelinating lesions in the cervicomedullary junction or cervical spinal cord (high perfusion and low FA in tumors)
13.2.3 Multiple Sclerosis
Multiple sclerosis (MS) is an inflammatory demyelinating disorder of the brain and spinal cord, with cord lesions found in up to 99% of autopsy cases.22,23
Increased diffusivity has been found in focal MS lesions in the cord, with a significantly higher isotropic diffusion coefficient, when compared to healthy controls.24 Valsasina et al have shown, in their study of 44 patients with MS and 17 healthy controls, that the average cervical cord FA was significantly lower in MS patients compared to controls.25
Early studies that performed DTI measurements in patients without T2-visible cord signal abnormalities have revealed significantly lower FA values in the lateral, dorsal, and central parts of the normal-appearing white matter (NAWM) in MS patients.26 Several studies confirmed that significant changes in DTI metrics are present in the cervical spinal cord of MS patients, in the absence of spinal cord signal abnormality at conventional MRI examination.26,27,28 Diffusional kurtosis imaging of the spinal cord, used recently in one study, has demonstrated not only white but also gray matter damage in patients with MS.29
Furthermore, DTI metrics of the spinal cord in MS patients has been compared with electrophysiological studies. On DTI acquired in 28 healthy volunteers and 41 MS patients, FA and ADC were evaluated in NAWM at the cervical level and correlated with motor-evoked potentials (n = 34).30 Asymmetric anatomical changes in spinal cord NAWM were found in DTI, which corresponded to asymmetric electrophysiological deficits for both arms and legs. Overall, DTI studies of the spinal cord in MS patients have demonstrated and confirmed the spinal cord damage and opened the pathway for further clinical studies.
13.2.4 Neuromyelitis Optica
The identification, in 2004, of a disease-specific autoantibody, neuromyelitis optica immunoglobulin G (NMO-IgG), in the serum of patients with NMO ended the debate about whether NMO is a variant of MS or a distinct disease.31 NMO-IgG binds selectively to aquaporin-4 (AQP-4), a water channel that is densely expressed in astrocytic foot processes at the blood-brain barrier (BBB). NMO is currently defined as an autoimmune antibody-mediated disease, induced by a specific serum autoantibody, the NMO-IgG, directed against AQP-4. Unilateral or bilateral severe optic neuritis (ON) usually precedes longitudinally extensive transverse myelitis (LETM). Extensive signal abnormalities that extend to multiple segments (> three segments), with cavitations, and patchy enhancement are typical imaging findings. Reported brain abnormalities range from 20 to 89% of cases, depending on geographical area.32
The crucial point is the differentiation from MS, because some MS treatments can exacerbate NMO. DTI of the cervical spinal cord was used in one study to assess cord damage in NMO.33 Ten NMO patients showed reduced FA and increased MD, compared with 12 healthy controls. In another study, decreased FA was found in patients with NMO when compared with patients with relapsing-remitting MS.34 The study demonstrated extensive normal-appearing spinal cord damage in NMO patients, including peripheral areas of the cervical spinal cord.
13.2.5 Transverse Myelitis
Transverse myelitis (TM) is an acute inflammatory disease of the spinal cord with a heterogeneous pathogenesis. MRI findings include central T2-hyperintensity usually extending over more than two segments, and involving more than two-thirds of the cross-sectional area of the cord, with or without enhancement.35
In one study, a pulse-triggered DTI sequence with an rFOV technique and coronal acquisition was used for the assessment of the cervical spinal cord in patients with myelitis.36 Qualitative assessment of the spinal cord damage was evaluated in 12 healthy volunteers and 40 consecutive patients with myelitis (25 MS, 11 neuromyelitis optica, 1 sarcoidosis, 1 Gougerot–Sjögren syndrome, and 2 cases of idiopathic myelitis). FA and MD measurements clearly demonstrated a significant FA increase and an MD decrease in active lesions. In the absence of active lesions, and regardless of the pathology, FA decreased and MD increased significantly.36
Clinical Rationale for DWI/DTI in MS, NMO, and TM
Not yet part of the routine protocols
May be useful in the future for therapy monitoring
Differentiation between demyelinating/inflammatory disorders and spinal cord ischemia
13.2.6 Spinal Cord Trauma
Knowing the limitations of conventional MRI techniques in estimation of the extent of Spinal cord injury (SCI), there is a need for the evaluation of new techniques. DWI has been first evaluated in animal studies showing promising results.37 The comparison of conventional T2-weighted images and DWI in acute SCI showed comparable detection rates for spinal cord damage in 18 patients within 24 hours postinjury.38 The detection rates of high signals on T2-weighted and DWI did not show significant differences, being 94 and 72%, respectively.38 In another study, conventional MRI and multishot, navigator-corrected DWI were performed in 20 patients with acute spinal cord trauma using 1.5 T MRI within 72 h after the onset of trauma.39 In that study 20 cases were classified into four types: edema type (10 cases), mixed type (6 cases), hemorrhage type (2 cases), and compressed type (2 cases). DWI hyperintensity was detected in edema cases, most probably representing cytotoxic edema, as well as in two patients with cord compression. Inhomogeneous signals were seen in patients with mixed-type injury. An important limitation of the study is the lack of axial DWI images, lack of normal controls, and low b values (400 and 500 s/mm2) used.39
Clinical Rationale for DWI/DTI in Traumatic Spinal Cord Injury
Not yet part of the routine protocols
Promising technique for evaluation of injury epicenter, differentiation of injured and normal spinal cord in the absence of T2-signal changes
13.3 Clinical Applications for DWI and DTI of the Vertebral Bodies
13.3.1 Vertebral Body Fracture
The normal bone marrow in an adult consists of approximately 50% fat and 50% water.
Osteoporotic fractured vertebra contains fat and edema. In malignant vertebral fractures, the tumor cells usually have replaced the bone marrow. The reliable imaging differentiation of benign and malignant vertebral body fractures remains a diagnostic challenge. In the first published study, all benign osteoporotic fractures were hypointense or isointense on steady-state free procession (SSFP)-based DWI, whereas malignant fractures showed hyperintensity.1 Malignant fractures show restricted diffusion due to dense tumor-cell packing (▶ Fig. 13.6). Acute osteoporotic fractures demonstrate increased diffusion caused by increased water proton mobility in the bone marrow edema (▶ Fig. 13.7). Important exceptions to that rule are sclerotic metastases and treated metastases, which will be hypointense (false-negative results).40 Reported ADC values of benign osteoporotic and benign traumatic fractures vary from 0.32 to 2.23 × 10–3 mm2/s, whereas the range in malignant fractures or metastases is 0.19 to 1.04 × 10–3 mm2/s.41 The calculated diffusion coefficients will depend on the technique applied and also on fat saturation. The diffusion weighted reversed fast imaging with steady state free precession (DW-PSIF) sequence (delta = 3 ms) was reported in a recent study to have the highest accuracy in differentiating benign from malignant vertebral fractures. Qualitatively assessed opposed-phase, DW-EPI, and DW single-shot turbo spin echo (TSE) sequences and ADCs (DW-EPI) cannot be used to accurately differentiate benign from malignant vertebral fractures.41
Fig. 13.6 Metastatic vertebral body fracture in a 71-year-old patient with a history of adenocarcinoma. (a) Sagittal short tau inversion recovery magnetic resonance imaging (STIR) shows high signal in the L3 vertebral body. Apparent diffusion coefficient (ADC) maps calculated from (b) diffusion weighted echo-planar imaging (DW-EPI) and (c) half-Fourier acquisition single-shot turbo-spin-echo diffusion weighted MR (DW-HASTE) images show average ADC values of 0.9 × 10–4 mm2/s (DW-EPI) and 1.38 × 10–4 mm2/s (DW-HASTE) (arrows). (Courtesy of Andrea Baur-Melnyk. From Magnetic Resonance Imaging of the Bone Marrow, 2013. With kind permission from Springer Science and Business Media.)
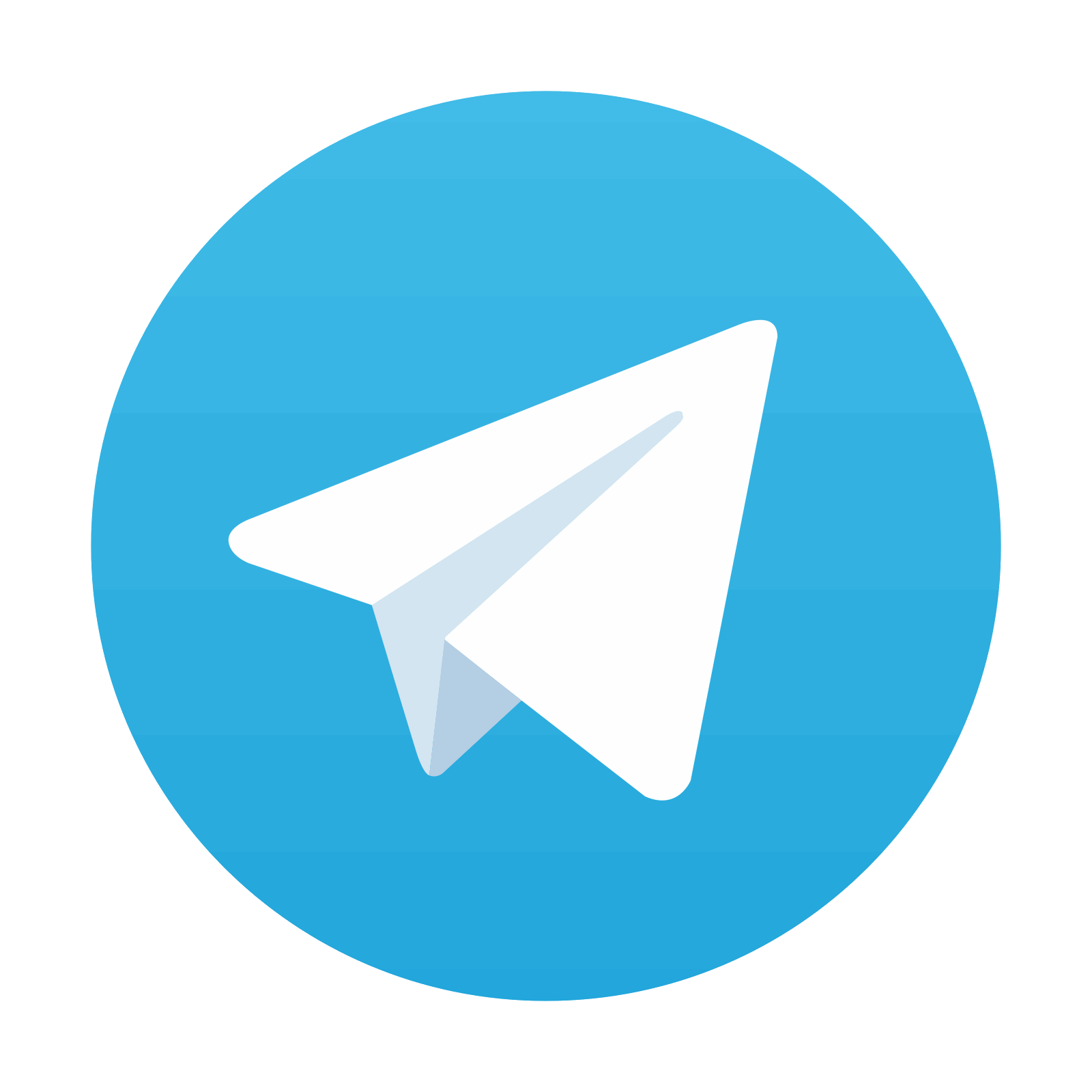
Stay updated, free articles. Join our Telegram channel

Full access? Get Clinical Tree
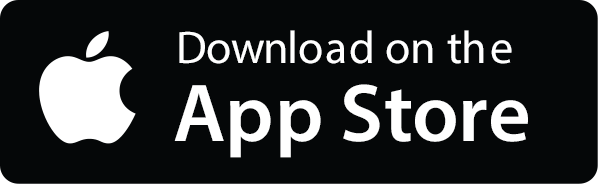
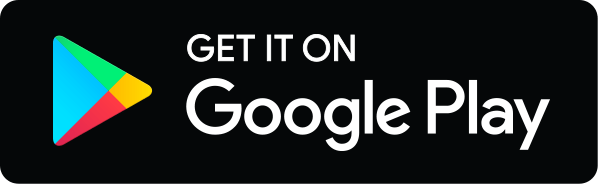