Electricity and Magnetism
Objectives
At the completion of this chapter, the student should be able to do the following:
• Discuss the roles of the following: Franklin, Coulomb, Tesla, Faraday, and Oersted.
• Describe the nature of the electric field.
• List the four laws of electrostatics.
• Describe the nature of magnetism.
• Distinguish between magnetic susceptibility and magnetic permeability.
• Examine the role of electromagnetism in magnetic resonance imaging (MRI).
Key Terms
Both electric and magnetic fields and electromagnetic radiation are used in magnetic resonance imaging (MRI). These are not unlike the physical agents used in x-ray imaging, although their method of use is vastly different.
Electricity is used to produce the primary static magnetic field (B0) and the secondary gradient magnetic fields (BX, BY, BZ). These magnetic fields interact with the intrinsic nuclear magnetism of tissue, the proton dipoles. The tissue is excited with a radiofrequency (RF) pulse produced by an electrically stimulated coil, which usually also receives the magnetic resonance (MR) signal emitted from the body.
A modest understanding of the fundamental physical concepts of electricity and magnetism is necessary for an understanding of MRI. A logical sequence for dealing with such subjects is to discuss electrostatics and electrodynamics to develop an understanding of electricity. This is followed by a discussion of the phenomenon of magnetism, which leads into electromagnetism and electromagnetic radiation.
Electrostatics
The smallest unit of electric charge is contained in the electron, and it is negative. The proton likewise contains one unit of positive electric charge. Electric charge, unlike other fundamental properties of matter such as mass, cannot be subdivided. Furthermore, larger quantities of charge can only be multiples of the unit charge.
Although the magnitude of the electric charge of an electron and proton is the same, the mass of the proton is approximately 1840 times the mass of the electron. Protons are relatively fixed by virtue of their position in the nucleus of an atom, whereas electrons are free to migrate from atom to atom under some circumstances.
It was not until the 1750s that Benjamin Franklin first described the nature of electric charge. Franklin’s experiments have been popularly associated with flying kites, which is indeed true (Figure 2-1). However, he was and is also credited with being a laboratory scientist. Nevertheless, Franklin erroneously assumed that positive charges were migrating down his kite string.
Franklin called this migration of charge electricity and therein lies the origin of a confusing convention: that electric current (I) in a conductor flows opposite to electron movement. Franklin is also credited with much of the current terminology dealing with electricity: charge, discharge, battery, shock, positive, negative, plus, and minus among others. Not until the work of J.J. Thompson in the 1890s was the electron identified as the fundamental charged particle responsible for electricity.
The Coulomb
The unit of electric charge is the coulomb (C), with 1 C consisting of 6.24 × 1018 electronic charges, a sizable number of electrons. This definition, adopted in 1910, is such a strange number because the system for electrical measurement had already been established before the discovery of the electron. Ideally, the electron should be the smallest unit of electric charge. Instead, the charge on an electron is 1.6 × 10−19 C.
The coulomb is an unfamiliar quantity to most people. The lightning associated with the thunderstorm shown in Figure 2-2 ranges from perhaps 10 to 50 C. The shock experienced when grasping a doorknob in the dry air of winter is measured in microcoulombs (µC), a mere 1012 electrons.
Electrification
Whenever electrons are added to or removed from material, the material is said to be electrified. Electrification can occur by contact, friction, or induction.
Electrification by contact occurs when an object having an excess number of electrons contacts a neutrally charged object or an object with a deficiency of electrons. Rubbing a balloon over your hair to make it stick to the wall is an example of electrification by friction. The loosely bound electrons of hair are mechanically transferred so that the balloon becomes electrified.
Electrification by induction occurs when a highly electrified object comes close to a neutral object so that the electrons are transferred by spark. Lightning bolts jump from cloud to cloud or cloud to earth to shed themselves of excess electrons. The earth is the ultimate sink for excess electrons and is called an earth ground or just ground in engineering terms.
The Electric Field
Charge cannot be destroyed by conversion to another form. In the universe, the total number of negative charges equals the total number of positive charges, and the net charge is always the same—zero. Furthermore, charge is quantized; it comes in discrete bundles rather than in a continuum of values.
A force field called the electric field (E) is associated with each charge. The proof of the electric field is much the same as that of a gravitational field—the exertion of a force.
The gravitational field produces a force that causes one mass to be attracted to another. Similarly, the electric field creates a force between one charge and another. Although the gravitational force is always attractive, the force of the electric field can be attractive or repulsive, depending on the nature of the charges involved.
The electric field is most easily visualized as imaginary lines radiating from an electric charge (Figure 2-3). The intensity of the electric field is proportional to the concentration of lines, and therefore the electric field decreases as the square of the distance from the charge.
The electric field is a vector quantity; that is, it has not only magnitude but also direction. The direction of the electric field is determined by the movement of a positive charge in the electric field. In the presence of an electron, the positive charge is attracted, and therefore the imaginary lines of the electric field are directed toward the electron.
Alternatively, if the positive charge is in the field of a proton, the positive charge will be repelled, and the imaginary lines will radiate from the proton (see Figure 2-3). Neutral objects, such as a neutron, do not have an electric field.
The magnitude of the electric field is defined as the force on a unit charge in the field as follows:
Although electric charge is discrete, its associated electric field is continuous, and this is a fundamental characteristic of field theory. Particles add in a quantized fashion; fields add in a continuum by superposition. For example, the force on an electric charge is determined by not only the movement of that charge but also the electric fields produced by all other electric charges near and far. That is field superposition.
Electrostatic Laws
The nature and intensity of the electric field form the basis for the four principal laws of electrostatics.
Unlike versus Like Charges.
Because of the vector nature of the electric field, like electric charges repel, and unlike electric charges attract. Particles having no electric charge, such as neutrons, are not influenced by an electric field.
Coulomb’s Law.
The force of attraction or repulsion between electrostatic charges was first described by Charles Coulomb in the 1780s. Coulomb noted that the force was proportional to the product of the two charges and inversely proportional to the square of the distance separating them. Coulomb’s law can be stated as follows:
The electrostatic force is one of the five fundamental forces in nature. It is 1037 times stronger than the gravitational force, 1011 times as strong as the weak interaction, about equal to the magnetic force, and about of the strong nuclear force.
An electrified balloon will attract paper or repel a small stream of water. The electrostatic force of attraction holds electrons in orbit in an atom. The electrostatic force of repulsion among protons is countered by the attraction between neutrons and protons by the strong nuclear force but ultimately limits the size of the nucleus.
Charge Distribution.
Because protons are fixed, whereas electrons are free to move, the remaining discussion of electrostatic phenomenon concerns electrons. Because the electric field associated with each electron radiates uniformly, electrons on any electrified object tend to be separated uniformly and to the maximum dimensions possible (Figure 2-4).
Charge Concentration.
If the electrified object is regularly shaped, such as a sphere or wire, the distribution of electrons on the surface will be uniform. On the other hand, if the surface is irregularly shaped (for example, has a point as in an electrified cattle prod or a microfocus field-emission x-ray tube) electrons will be concentrated at the sharpest region of curvature.
Electric Potential Energy
When two like charges are pushed together or two unlike charges are pulled apart, work is required. The resulting system has the ability to do work and therefore has electric potential energy.
The work done to create electric potential energy comes from an energy source, and the work obtained from the potential energy is deposited in an energy sink. A hydroelectric generator and a steam generator are common energy sources that convert mechanical energy into electric potential energy. Energy sinks, such as motors, lamps, and heaters, abound.
The electric potential energy of a charge, q, is converted to kinetic energy when the charge is in motion and work is done. The electric potential energy of a charge influenced by the electric field of other charges is given by the following:
Electric potential has units of joule per coulomb, and it is not a force at all but rather a force used to do work. Therefore electric potential is given a special name, volt, and is commonly called voltage.
Electrodynamics
Electrodynamics involves what is commonly called an electric current or electricity. Electricity deals with the flow of electrons in a conductor.
A Houston freeway at rush hour is analogous to an electric current (Figure 2-5). Normally, about 10,000 cars (electrons) each hour will pass any given point on a six-lane freeway. If there is a wreck or construction, the speed of each car is reduced (resistance) at that point and the flow of traffic restricted. At an interchange, some cars may exit onto alternative routes. This allows those cars remaining on the main freeway and those that exited to travel faster (parallel circuit). The traffic flow in each branch is reduced because there are fewer cars in each route, but the total flow will remain constant.
Electric Current
The electrons flowing in a conductor behave like the automobiles on the freeway. For an electric current to exist, a closed circuit is necessary. Each electron must have a place to go. If there is a barricade in a conductor, such as an open switch, electron flow ceases.
The reason for investigating electricity is that work can be extracted from the kinetic energy of electrons moving in a conductor. The number of electrons involved is given a special name, the ampere (A), which is equal to the flow of 1 C each second (1 A = 1 C/s).
An ampere is a rather large electron flow, 6.24 × 1018 electrons each second. Electrons cannot be counted that fast, so electric current is measured by the associated magnetic field.
Electric currents range from thousands of amperes in lightning bolts to picoamperes in electronic equipment. Household current can be up to approximately 30 A on any circuit. A current of only 100 milliamperes (mA) at 110 volts is almost always fatal, which is the reason grounded circuits and ground fault circuit interrupters are necessary elements in home wiring.
Direct Current.
If the energy source propels electrons in only one direction, as with an automobile battery, the form of electricity is direct current (DC) (Figure 2-6). At time zero, when the switch is open, no current flows. The instant the switch is closed, electrons flow—but only in one direction.
Alternating Current.
On the other hand, if the energy source is of an alternating form (Figure 2-7), alternating current (AC) is produced. When the switch is open, no current flows. When the switch is closed, electrons first flow in one direction and then reverse direction and flow in the opposite direction.
When electrons begin flowing in the positive direction, they begin slowly and speed up to a maximum, represented by the first peak of the current waveform (see Figure 2-7). Then they begin to slow down, still traveling in the same direction, until they momentarily come to rest. This moment of rest occurs 120 times each second and is represented by the zero crossing of the waveform. Then the electrons reverse direction, first speeding up to a maximum, then slowing down to zero again.
The number of electrons in the circuit remains constant, and their net movement is zero. Both AC and DC are used to great advantage in MRI.
Phase.
The current waveform shown in Figure 2-7 illustrates that at any instant, all electrons are moving in the same direction with the same velocity. They are said to be in phase, and the electricity is commonly called single-phase current.
Actually, commercial electric power is generated and transmitted as three-phase current. A three-phase waveform is shown in Figure 2-8. At any instant, not all electrons are moving in the same direction, and those that are have different velocities. They are out of phase with one another. The concept of phase is important to the understanding of the way an MR image is produced.
Ohm’s Law
Electrons do not flow unimpeded in a circuit. They behave much like an individual walking along a crowded sidewalk, bumping into people. Electrons bump into other electrons of the conductor. This property of any electric device is called impedance, and it is a function of the size, shape, and composition of the conductor or circuit element.
There are three types of electric impedances: capacitive, inductive, and resistive. If the work done on the device changes the electric energy into heat, the impedance is resistive, and it is equal to the electric potential divided by the current.
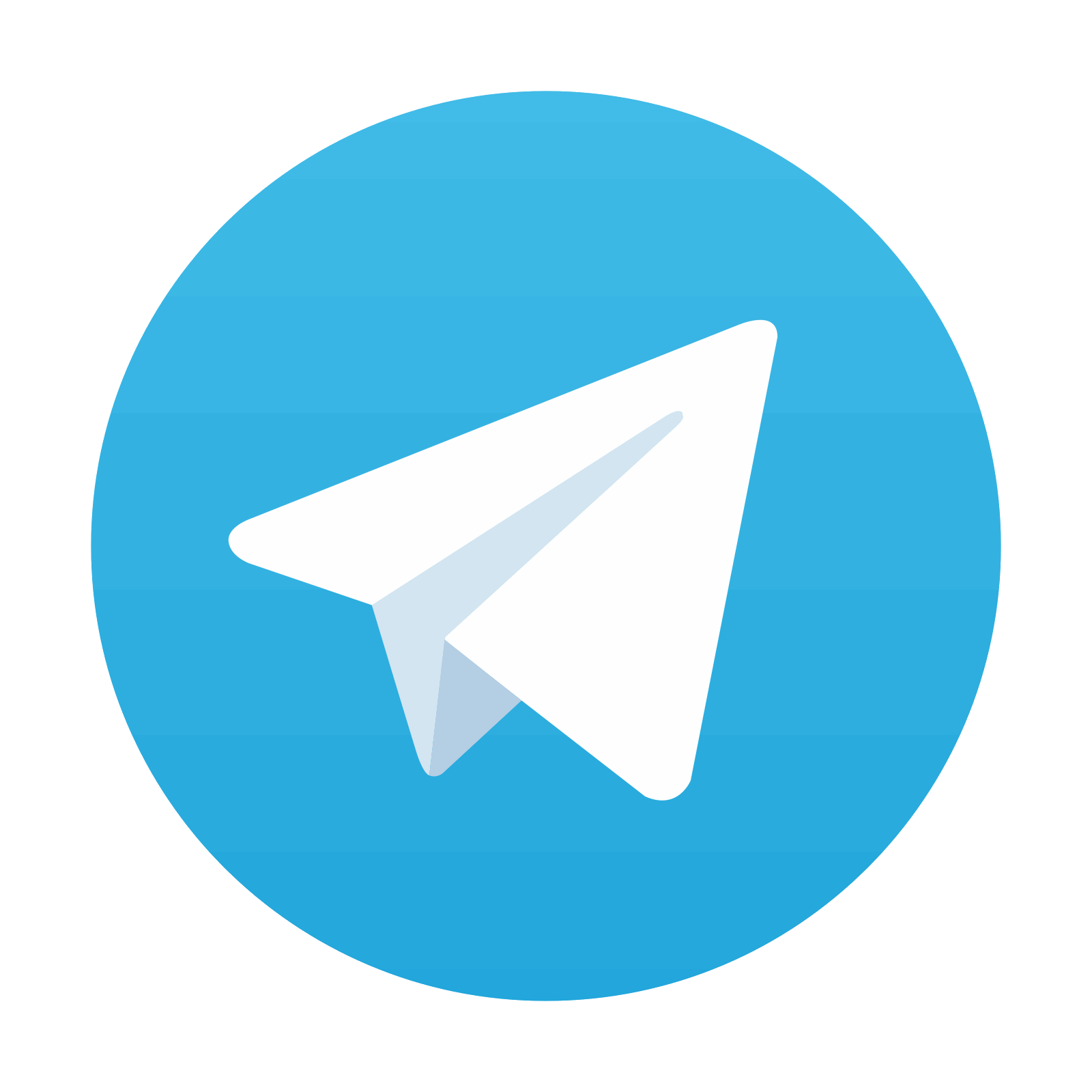
Stay updated, free articles. Join our Telegram channel

Full access? Get Clinical Tree
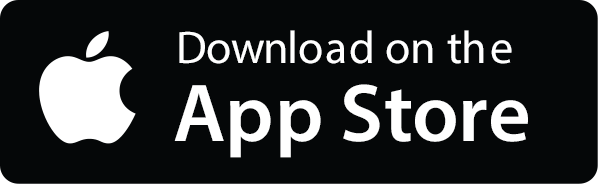
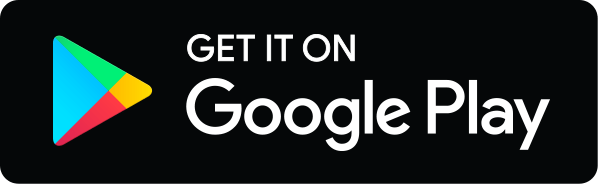