Key Facts
- •
Peripheral nerves are vulnerable to compression at sites of fibrous and fibroosseous tunnels.
- •
Early diagnosis is important because early nerve injuries may be reversible, whereas late injuries are not.
- •
Nerve compression can be caused by many different factors: (1) space occupying lesions (e.g., ganglion cysts, soft tissue tumors, bony spurs, bursitis, hematoma, callus, mass effect from inflammation of surrounding structures); (2) edema and tenosynovitis caused by overuse or inflammatory arthritis or edema and thickening of connective tissue caused by hormonal changes; (3) edema and fibrosis caused by mechanical friction about the nerve; and (4) dynamic compression of the nerve caused by normal or near-normal anatomic structures during certain movements.
- •
Initial diagnosis is based on presenting symptoms and physical exam. Electrodiagnostic testing helps to confirm the diagnosis and localize the site of injury. In difficult cases, imaging can help to define the site and etiology of nerve compression or to establish an alternative cause for the symptoms.
- •
Radiographs are the first step in the workup of nerve compression. They are useful for demonstrating osseous causes of nerve compression but are of limited utility in demonstrating the nerve and surrounding soft tissues. Specialized radiographic views and computed tomography scans can help to delineate bony anatomy of the carpal, cubital, and tarsal tunnels.
- •
Magnetic resonance imaging (MRI) and, in experienced hands, ultrasound are used to visualize nerves and surrounding soft tissue structures directly. On T1-weighted MR images, the normal nerve (seen in cross-section) is a smooth, rounded, or oval low signal structure, surrounded by bright T1 fat. In the median and sciatic nerves, individual fascicles are visible on MR images. When seen on ultrasound, normal nerves demonstrate a fascicular pattern of internal echoes. Both MRI and ultrasound can also be used to assess for post-denervation muscle edema and atrophy. As with electromyography, the distribution of muscle changes on MRI and ultrasound can be used to determine the site of nerve injury.
Peripheral nerves are vulnerable to compression at sites of fibrous and fibroosseous tunnels. Depending on the nature of the insult and the types of fibers that constitute the nerve at that site, nerve injury may result in sensory and/or motor symptoms. Early
* The author wishes to thank Dr. Elizabeth Raynor, Dr. Robert Kane, Dr. Stephen Farraher, Clotell Forde, and Ronald Kukla for their assistance in preparation of this chapter and to thank Steven Moskowitz for his assistance in preparation of the diagrams presented here.
diagnosis of nerve compression is important because early nerve injuries may be reversible, whereas late injuries are not. Initial treatment may consist of conservative therapy, but early surgical intervention (release) may also be indicated in order to help prevent permanent nerve injury.Initial diagnosis of nerve injury is based on presenting symptoms and physical exam. Electrodiagnostic testing is used to confirm the diagnosis and help localize the site of injury. Definitive diagnosis based on the clinical and neurophysiologic exam may be difficult when findings are nonspecific or inconsistent, when multiple nerves or multiple sites of compression are involved, or when another condition mimics the symptoms of nerve compression or coexists with the nerve compression. In these cases, imaging can play a role in helping to define the site and the etiology of nerve compression or in helping to identify an alternative etiology for the symptoms. Radiographs and computed tomography (CT) scan can help to identify bony lesions that can cause nerve impingement. Magnetic resonance imaging (MRI) and ultrasound provide direct visualization of the nerve and any surrounding abnormalities.
This chapter reviews compression neuropathies of the upper and lower extremities ( Box 14-1 ) and describes the role of imaging modalities in their diagnostic evaluation.
SHOULDER
Suprascapular notch (suprascapular nerve and its supraspinatus and infraspinatus nerve branches)
Quadrilateral space (axillary nerve)
ELBOW/FOREARM
Radial tunnel (radial nerve, PION)
Cubital tunnel (ulnar nerve)
Pronator teres syndrome (median nerve)
Kiloh-Nevin’s syndrome (AION)
WRIST
Carpal tunnel (median nerve)
Ulnar tunnel or Guyon’s canal (ulnar nerve)
PELVIS/HIP
Piriformis syndrome (sciatic nerve)
KNEE
Tibial nerve compression in popliteal fossa (tibial nerve)
Peroneal or fibular tunnel (common peroneal nerve and its superficial, deep, and recurrent nerve branches)
ANKLE
Tarsal tunnel syndrome (posterior tibial nerve and its medial and lateral plantar nerve branches)
Sinus tarsi syndrome (terminal branch of the deep peroneal nerve)
FOOT
Morton’s neuroma at metatarsal heads (plantar digital nerve)
NERVES AND NERVE INJURY
A peripheral nerve is composed of many nerve fibers bound together ( Figure 14-1 ). Depending on its function, the peripheral nerve contains varying combinations of motor, sensory, and autonomic fibers. Motor (efferent) fibers deliver impulses from the central nervous system (CNS) to skeletal muscles for voluntary activity. Sensory (afferent) fibers transmit impulses from skin receptors to the CNS. Autonomic fibers (efferent) control certain smooth muscle, glandular, and trophic functions.
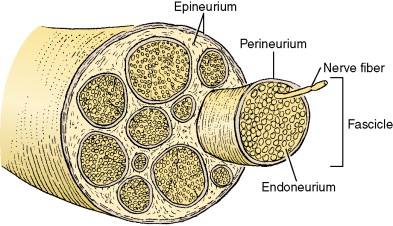
The nerve may be injured by direct trauma (crush or transection), traction, mechanical or functional compression, or repetitive local friction. When nerve conduction is interrupted, nerve function is impaired. When motor nerves are affected, weakness, paralysis, and muscle atrophy can occur. When sensory nerves are affected, subjective sensations of pain and paresthesia (e.g., numbness, tingling, crawling sensation) and objective findings of analgesia and anesthesia can occur. When autonomic nerves (to the skin) are affected, dryness, cyanosis, hair loss, brittle nails, ulceration, and slow wound healing can develop. Injury to a nerve may take the form of neurapraxia, axonotmesis, or neurotmesis. Although early nerve compression results in neurapraxic injury, severe or chronic compression can progress to axonotmesis or neurotmesis. Different grades of injury can coexist in the same nerve. Neurapraxia, the least severe category of injury, involves focal damage to the myelin sheath, without axonal disruption. The mildest form of neurapraxia results from transient ischemia rather than actual loss of myelin; an example is the symptoms that develop from crossing one’s legs. Uncomplicated neurapraxia usually resolves spontaneously and relatively quickly (sometimes hours to days and usually within 3 months) as the myelin regenerates. Axonotmesis, a more severe level of injury, results when the axon is interrupted, but the Schwann cell basal lamina and endoneurium remain intact. When focal axonal disruption occurs, the axon distal to the site of injury also degenerates after several days (Wallerian degeneration). Because the supporting structure surrounding the axon is not destroyed, the axon can regenerate along intact pathways, at a rate of approximately 1 mm/day. Prognosis for recovery from axonotmesis is good, but recovery can take weeks to months, depending on the rate of regeneration and the distance between the site of injury and the target organ. Neurotmesis is the most severe level of injury, characterized by complete axonal disruption and discontinuity of some or all of the surrounding connective tissue. Neurotmesis may occur due to mechanical disruption or due to severe scarring. Wallerian degeneration of the distal axon occurs, but because the lattice surrounding the axon is also disrupted, regeneration cannot occur. This severe degree of injury requires surgical repair and nerve reconstruction in order for function to be restored.
COMPRESSION-ENTRAPMENT NEUROPATHY
Compression neuropathy refers to nerve damage resulting from pressure on a nerve. Some authors distinguish entrapment neuropathy as a subset of compression neuropathy involving pressure on a nerve from an anatomic or pathoanatomic structure (e.g., flexor retinaculum in carpal tunnel syndrome). Almost any peripheral nerve in the body can be compressed, but nerve compression tends to occur at certain sites where the area around the nerve is more constrained, most often within known fibrous or fibroosseous tunnels. Compression injury can be acute, chronic, or intermittent.
Damage to a nerve from pressure is termed compression neuropathy ; entrapment neuropathy is a subset of this, caused by pressure due to anatomic structures or anatomic variations.
There are numerous causes of compression entrapment neuropathies; the relative incidence varies with location. A nerve may be predisposed to compression due to congenital narrowing of its osseous tunnel or abnormal thickening of its soft tissue roof. Fractures or joint instability can cause abnormalities of bony alignment that can narrow the fibroosseous canal and can result in nerve compression. Compression neuropathies can be caused by space-occupying lesions, such as ganglion cysts, soft tissue tumors, bony spurs, bursitis, hematoma, or callus, impinging on the nerve. Inflammation of structures surrounding the nerve can also produce mass effect. Edema and tenosynovitis due to overuse or inflammatory arthritis or edema and thickening of connective tissue caused by hormonal changes can result in nerve compression. Mechanical friction about the nerve can lead to local edema and fibrosis and can contribute to nerve compression as well. Dynamic compression of the nerve can occur when normal anatomic structures—fibrous, muscular, or tendinous bands overlying the nerve—result in compression during certain motions. This dynamic compression can occur in the setting of only minimal anatomic variation and can be especially problematic when associated with chronic repetitive activities. When two sites of compression occur in tandem along a single nerve, “double crush” syndrome may occur. The presence of the proximal lesion lowers the threshold for development of symptoms from the distal lesion. The classic example of double crush syndrome is nerve impingement in the cervical spine predisposing to development of carpal tunnel syndrome. A similar phenomenon is seen in patients with an underlying polyneuropathy and can be seen in a number of systemic diseases such as diabetes mellitus, rheumatoid arthritis, hyperthyroidism, alcoholism, malnutrition, renal failure, and hematologic disorders.
The presence of proximal compression on a nerve or of a polyneuropathy, such as that due to diabetes mellitus, lowers the threshold for a more distal second compressive lesion to become manifest.
The effect of compression on the nerve is mediated by ischemia and edema. Compression of the vasa nervorum can result in ischemia, disruption of the blood-nerve barrier, and venous congestion. This, in turn, leads to epineurial and endoneurial edema and increased pressure within the endoneurium. In early stages, symptoms may be intermittent, with interval recovery of intraneural circulation and resolution of intraneural edema. As the disease progresses, prolonged edema of the epineurium may result in fibrosis, further constricting the nerve. Long-standing compression causes damage to the myelin sheath and ultimately results in axonal interruption and distal degeneration, with permanent loss of nerve function and atrophy of the denervated muscles.
Signs and symptoms of compression neuropathy can be nonspecific. Patients may present with paresthesias and sharp burning pain in the distribution of the affected nerve; the symptoms are often worse with motion and worse at night. There may be muscle weakness, with or without associated cutaneous sensory loss. Compression of motor nerves can cause deep, diffuse, poorly localized pain. In advanced cases, muscle atrophy will be seen. Trophic changes due to autonomic nerve dysfunction may be present. The pattern of sensory and muscle involvement reflects the site of nerve compression. However, it may difficult to distinguish between two different sites of compression if their respective fields overlap.
Treatment of nerve compression depends on location, etiology, chronicity, and extent of existing damage. Initial treatment is generally conservative. When conservative measures fail, however, early surgical decompression is indicated in order to minimize permanent nerve injury. Conservative measures include eliminating aggravating activities, immobilization, heat, antiinflammatory medications, orthotics, and physical therapy. In some instances, local injection of corticosteroids can help alleviate symptoms and thereby also help confirm the diagnosis. Surgical treatment may include decompression of the nerve as well as nerve transposition, tenosynovectomy, arthrodesis, or osteotomy. Thorough decompression at every site of compression is a major goal and is important to prevent postsurgical recurrence of symptoms.
ELECTRODIAGNOSTIC EXAMINATION
Electrodiagnostic examination can help to confirm a diagnosis of peripheral nerve compression, localize a lesion, determine its pathophysiology, and grade its severity. The electrodiagnostic examination consists of nerve conduction studies, which can be performed on both motor and sensory nerves, and electromyography (EMG). Nerve conduction studies (NCS) can be performed using surface electrodes; EMG is performed using needle electrodes placed within the muscle being tested. NCS measures the velocity of nerve impulse conduction. Findings at EMG are dependent on the presence of axonal injury. Muscles studied at EMG examination are chosen specifically to localize the site of nerve injury.
Electrodiagnostic studies play an important role in the evaluation of nerve compression. Early nerve compression affects myelin and is associated with slowing of nerve conduction; compression injury can progress to involve axonal destruction, which results in correlative EMG changes. However, sensitivity and specificity of electrodiagnostic exam can be limited. Normal conduction time does not exclude the presence of compression. NCS can appear normal if slowing is mild or if a few fast-conducting fibers remain intact. Conduction can be slowed not only due to nerve compression, but also due to segmental demyelination from other forms of neuropathy. The sensitivity and specificity of the exam depends on the specific location and the clinical setting. It is not uncommon for results of NCS/EMG studies to be abnormal but not clearly localizing. Imaging studies can supplement electrodiagnostic exams by helping to localize and characterize a lesion at the site of suspected nerve compression or by demonstrating changes in the muscles innervated by that nerve.
OSTEOARTICULAR IMAGING FEATURES OF NERVES AND NERVE COMPRESSION
Radiographs and CT
Radiographs are an important first step in the workup of nerve compression. Radiographs best demonstrate osseous causes of nerve compression such as fracture, dislocation, callus, osteophyte, and exostosis. When necessary, specialized views have been developed to demonstrate the bony contour of certain fibroosseous tunnels. These targeted views are often now supplanted or supplemented by CT scans, which can better delineate bony contours and their relationship to nerve structures. Although CT has been used in the past for direct imaging of nerves, intrinsic soft tissue contrast on CT images is limited. Currently, MRI and, to a more limited extent, ultrasound are the methods of choice for direct imaging of nerves.
Magnetic Resonance Imaging
MR images have a high intrinsic soft tissue contrast that provides for direct depiction of normal and abnormal nerves, helps identify and characterize structures surrounding the nerve that might cause compression, and can also demonstrate post-denervation changes in muscles supplied by the affected nerve. The precise technique for imaging nerve entrapment depends on the specific site involved. In general, techniques are based on routine imaging protocols. For imaging about the shoulder, knee, ankle, or foot, standard shoulder and extremity coils can be employed. For imaging about the wrist, elbow, forearm, or forefoot, paired surface coils or a small flexible coil can be used. In order to image small structures, high-resolution techniques, based on relatively small fields of view and high image matrices, are optimal. Images obtained in the axial plane are important for identifying the nerve and detecting extrinsic compression. T1-weighted conventional spin echo or fast spin echo sequences depict the anatomy of the nerve and surrounding structures. Proton density weighted images are similar to T1-weighted images in that respect. T2-weighted conventional spin echo or fast spin echo images help to demonstrate edema within the nerve and to characterize surrounding masses. In order to detect subtle amounts of edema within the nerve or the surrounding soft tissues, fat-saturated T2-weighted images (of which short tau inversion recovery [STIR] images are one form) are employed. Intravenous gadolinium contrast may be used to determine whether a mass is cystic or solid and to help delineate vascular anatomy. Gadolinium is used commonly for many types of MRI examinations and is generally well tolerated, even in individuals with renal failure . (It should be noted that recent experience in a small group of patients primarily using a particular formulation of gadolinium contrast agent had introduced the possibility of development of nephrogenic systemic fibrosis as a potential complication of contrast administration in patients with diminished renal function. Additional data on this issue are currently being gathered. )
On T1-weighted images, fat is of high signal intensity and appears bright; muscles are lower in signal intensity and appear relatively dark. A normal nerve seen in cross-section on T1-weighted images is a smooth, round, or ovoid structure that is isointense to muscle and surrounded by high T1 signal perineural fat. On T2-weighted images, fluid is of high signal intensity or bright, and muscles appear relatively dark. Fat signal varies depending on technique and is generally higher in signal than muscle but not as bright as fluid. On T2-weighted images, the normal nerve is isointense or slightly hyperintense to muscle. In larger nerves, such as the sciatic and median nerves, the nerve in cross section demonstrates a fascicular pattern, with punctuate high signal intensity foci surrounded by lower signal intensity on both T1-weighted and T2-weighted images. Normal fascicles are uniform in size and shape. When the nerve is damaged, endoneural free water increases and the nerve becomes considerably hyperintense to muscle on T2-weighted images. The nerve may become enlarged, and nerve fascicles may become distorted. MR neurography refers to high-resolution MR sequences specifically tailored for depiction of the nerves.
On MRI, normal nerves are smooth, round or oval in cross section, and of intermediate signal intensity. Larger nerves may show separate bundles (fascicles). Damaged nerves typically are larger and higher in signal on T2-weighted images.
Signal from arterial and venous flow proximal and distal to the nerve segment of interest are suppressed using saturation bands. The significance of gadolinium enhancement of the nerve is controversial. Although some investigators associate nerve enhancement with nerve inflammation, others have reported poor correlation between enhancement and nerve pathology.
MRI can also be useful in assessing changes in muscles innervated by a compressed nerve. Muscle edema is an early postdenervation change that is seen as high signal intensity on T2-weighted and fat-saturated images. Eventually, muscle atrophy and fatty infiltration develop, producing a muscle that is smaller than normal in size with areas of high intramuscular signal on T1-weighted images. As with EMG, the distribution of muscle denervation can help to localize the level of nerve injury.
MRI depicts the fibroosseous canal surrounding a nerve. The bony canal may appear diminished in size. The fibrous portion may be thickened. Spurs and osteophytes can be seen on MRI but may be quite difficult to appreciate. Additional imaging with radiographs or CT can be helpful if subtle bony spurring or soft tissue calcification is suspected. MRI is particularly effective at depicting soft tissues structures surrounding or compressing a nerve. As a result, the site and etiology of nerve compression can often be conclusively established. A ganglion cyst appears as rounded, well-circumscribed, multilobulated structure adjacent to the joint, which is isointense to high signal (bright) on T1-weighted images and high signal on T2-weighted images. The ganglion cyst does not enhance internally, although a thin enhancing rim is usually visible. Lipomas are high signal intensity (bright) on T1-weighted images. A neurofibroma is typically seen as a cylindric mass that is not encapsulated. It often demonstrates a characteristic target appearance on T2-weighted images, with central low signal and peripheral high signal, and enhances centrally. A schwannoma is low to intermediate signal on T1-weighted images and markedly hyperintense on T2-weighted images. It may be heterogeneous on T2-weighted images and can show hemorrhagic or necrotic foci on contrast-enhanced images. It may also have a target-like appearance, similar to neurofibromas. In many schwannomas, the nerve can be identified on one side of the mass. Imaging features are not reliable for distinguishing between benign and malignant nerve sheath tumors. Heterogeneity and infiltrating margins are suggestive of malignancy but not specific for it.
Ultrasonography
Although MRI is considered the modality of choice for imaging peripheral nerves, ultrasound, in the hands of an experienced practitioner, can provide a useful alternative. The quality of ultrasound images depends heavily on the skill of the individual performing the ultrasound. It requires a relatively high level of expertise for successful nerve imaging. Ultrasound has the advantage of being noninvasive and low cost. It also facilitates imaging of the nerve over long segments and permits both static and dynamic assessment of the nerve. In the upper extremity, the radial nerve within the upper arm, the anterior osseous nerve within the forearm, the median nerve within the carpal tunnel, and the ulnar nerve within the cubital tunnel and within Guyon’s canal can be examined. In the lower extremity, the common peroneal nerve at the fibular neck, the posterior tibial and plantar nerves within the tarsal tunnel, and the interdigital nerves within the intermetatarsal spaces can be examined. Experience with ultrasound of the median nerve for evaluation of carpal tunnel syndrome is growing. Experience with ultrasound imaging of other peripheral nerves is more limited, but clinical utility of the technique is well documented.
Successful nerve imaging with ultrasound depends on optimized technique. Linear array transducers are preferred over sector transducers because of their wider near-field view. Sophisticated near-field focusing can eliminate the need for a stand-off pad. Recommendations regarding transducer frequency vary somewhat. In general, higher frequencies help to provide high spatial resolution and are desirable for identifying small structures and subtle differences in internal echotexture. However, lower frequencies are preferred when increased penetration is required in order to visualize deeper structures (e.g., the sciatic nerve). At our institution, we employ a compact linear array 7- to 15-MHz broadband transducer. The appearance of the nerve can be expected to vary with the resolution of the images, with the characteristic fascicular pattern becoming more apparent on higher-resolution images. Use of the lowest gain setting helps minimize spurious echogenicity. Echogenicity refers to the intensity of echoes generated by a structure imaged using ultrasound. Highly echogenic structures appear bright on ultrasound images; hypoechoic structures contain somewhat lower levels of echoes and are less bright; anechoic structures are devoid of internal echoes and appear uniformly dark. The nerve is examined systematically in both longitudinal and transverse planes to help distinguish it from other linear echogenic structures such as tendons and aponeuroses. The transducer must be maintained perpendicular to the structures of interest to avoid artifactual decrease in echogenicity related to anisotropy, a phenomenon observed in both nerves and tendons. Real-time examination during active or passive flexion and extension helps to distinguish the nerve from surrounding tendons and muscles, because the nerve remains relatively immobile. Comparison to the contralateral side helps to highlight abnormal size, echogenicity, and course of the nerve. Color Doppler imaging and power Doppler imaging, two techniques for demonstrating the presence of blood flow, can be helpful in identifying vascular landmarks and in assessing the vascularity of surrounding structures. The use of ultrasound to guide carpal tunnel release and also guide aspiration of cysts in the suprascapular notch and periacetabular region has been reported.
Certain nerves and portions of nerves can be imaged directly with ultrasound. Nerve size and shape, echotexture, and course can be demonstrated. Normal peripheral nerves are seen as markedly echogenic linear structures with multiple hypoechoic parallel but discontinuous linear areas separated by hyperechoic bands, surrounded by an echogenic envelope, when viewed on longitudinal scans. They are seen as oval to round structures with punctuate internal echoes, in a honeycomb-like pattern, when viewed on transverse scans. The hypoechoic foci seen interspersed between hyperechoic bands at higher frequencies correspond to neural fascicles. The normal range of values for the cross-sectional area of a nerve differs from nerve to nerve and also depends on the specific site along the length of the nerve. Inflammation of the nerve appears as either diffuse or segmental thickening, with decreased internal echogenicity and loss of the usual parallel linear echotexture.
Ultrasound examination can be used to assess causes of nerve entrapment. Tendons have an appearance similar to the nerves but are somewhat more echogenic, with a fibrillar echotexture. The normal tendon sheath appears as a very thin hypoechoic line around the tendon. In tenosynovitis, the tendon is surrounded by fluid, which may be anechoic or may contain internal echoes. A ganglion cyst appears as a round or oval anechoic lesion with thin regular margins and is often multilobulated. When inflamed, the ganglion cyst may demonstrate thicker walls, less well-defined margins, and internal echoes. A small anechoic “duct” may be seen extending between the ganglion cyst and a nearby joint. Anomalous muscles have ultrasound characteristics typical of all muscles. Scar tissue is typically seen as an area of increased echogenicity, reflecting the presence of fibrosis. Schwannomas and neurofibromas generally appear as hypoechoic masses with increased vascularity on color Doppler. Schwannomas tend to be round or oval, well defined, and eccentric to the nerve axis, with internal cystic areas and enhanced through-transmission. Neurofibromas tend to be lobulated and elongated along the nerve axis. The junction between the hypoechoic tumor and the normal echogenic nerve proximal and/or distal to it may be visible. However, the benign or malignant etiology of the tumor cannot be accurately determined by ultrasound. A traumatic neuroma appears as a focal rounded hypoechoic or hyperechoic mass, which may be encapsulated by a fibrous sheath or adhesed to surrounding structures. When there is edema in muscles surrounding a nerve, it may be seen as diffuse enlargement of the muscle, with preservation of internal architecture. Decreased internal echogenicity may or may not be appreciated. When subtle, these abnormalities may be highlighted by comparison to the contralateral limb. The use of ultrasound to demonstrate post-denervation changes in muscle has been described. Affected muscle may demonstrate decreased bulk and increased reflectivity (due to fatty replacement). Tendon rupture, a possible alternative etiology for muscle atrophy, can be excluded by ultrasound.
SPECIFIC SYNDROMES
Suprascapular Nerve Syndrome
Suprascapular nerve syndrome occurs due to compression of the suprascapular nerve either proximally within the suprascapular notch (incisura scapulae) or more distally within the spinoglenoid notch ( Figure 14-2 ). The suprascapular nerve (C5, 6) is a mixed motor and sensory nerve arising directly from the brachial plexus. The nerve passes deep to the trapezius muscle, parallel to the omohyoid muscle and then courses anteroposteriorly through the suprascapular notch. On the posterior side of the scapula, the nerve runs underneath the supraspinatus muscle, dividing into the supraspinatus nerve, which supplies motor branches to the supraspinatus muscle and sensory branches to the acromioclavicular and glenohumeral joints and the infraspinatus nerve. The infraspinatus nerve continues through the spinoglenoid notch into the infraspinatus fossa, where it supplies motor branches to the infraspinatus muscle and sensory fibers to the shoulder joint and scapula. The suprascapular notch is bridged by the superior transverse scapular ligament. In about 50% of people, the spinoglenoid notch is bridged by the spinoglenoid ligament. Compression of the nerve within the suprascapular notch will affect both the supraspinatus and infraspinatus muscles; compression in the spinoglenoid notch will affect only the infraspinatus muscle. The suprascapular artery and vein travel outside the fibroosseous tunnel, passing over the superior transverse ligament.
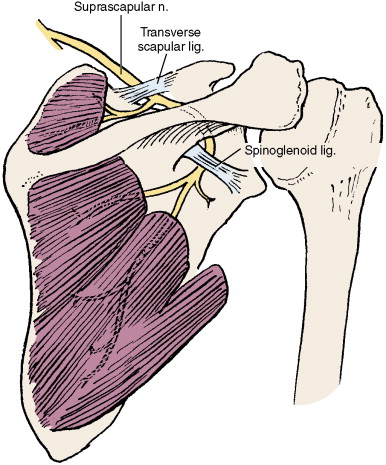
Compression of the suprascapular nerve at the suprascapular notch leads to atrophy of both the supraspinatus and infraspinatus muscles, whereas compression at the spinoglenoid notch leads only to infraspinatus atrophy.
Suprascapular nerve syndrome can result from compression by ganglion cysts, lipomas and tumors, anomalous or varicose veins, or traction injuries and secondary inflammation and fibrosis. Severe forward flexion of the shoulder, especially when the scapula is fixed, can tether the nerve in the suprascapular notch; this has been described as a mechanism of injury in pitchers. Stretching of the nerve can occur in activities with repetitive overhead motions involving the scapula, such as painting, tennis, volleyball, weightlifting, and gymnastics. Traction injuries of the nerve can occur acutely due to trauma and may also occur during rotator cuff surgery. Some individuals have a developmentally small or narrowed notch that predisposes them to entrapment. Patients with suprascapular nerve syndrome present with shoulder pain, which can mimic rotator cuff symptoms. After time, they may develop weakness and atrophy of the supraspinatus and/or infraspinatus muscles, which then limits their abduction and external rotation.
The suprascapular nerve is well visualized on routine shoulder MR images. The suprascapular notch is best seen on oblique coronal images oriented along the scapula. The spinoglenoid notch is best seen on axial images. The overlying ligaments are not typically visible. The area around the nerve should be carefully assessed for a mass. Lipomas (high signal or bright on T1-weighted images) and fibrosis (low signal or dark on T2-weighted images) can be relatively subtle. If a paralabral cyst or ganglion cyst is present, it will appear as well-circumscribed, rounded, or lobulated high T2 signal mass. These cysts often arise secondary to a glenoid labral tear, although the tear itself may or may not be apparent ( Figure 14-3 ). However, not all cysts will cause nerve compression. Veins in the region of the notch appear as tubular high T2 structures with a characteristic branching pattern. Prominent veins in the region of the notch are not uncommon, but anomalous and varicose veins have been implicated in nerve compression. Post-denervation edema and muscle atrophy may be seen. Muscle atrophy is often easiest to identify on oblique sagittal images. Post-denervation changes should be differentiated from other causes of shoulder girdle muscle atrophy including tendon tears and Parsonage-Turner syndrome.
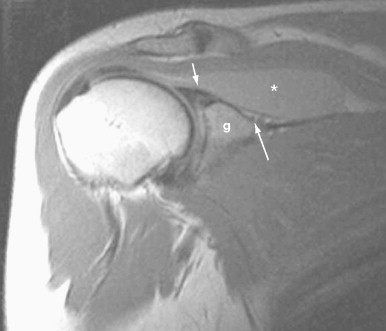
The use of ultrasound to identify ganglion cysts within the suprascapular notch has been described. Perilabral cysts can be treated temporarily via imaging-guided percutaneous aspiration and injection of corticosteroids. However, in the setting of a labral tear, a cyst will tend to reaccumulate unless the labral tear itself is repaired.
Quadrilateral Space Syndrome (Axillary Nerve)
Quadrilateral space syndrome (also known as lateral axillary hiatus syndrome ) occurs due to compression of the axillary nerve or one of its branches within the quadrilateral space. The quadrilateral space is demarcated by the long head of the triceps brachii muscle medially, the teres minor muscle superiorly, the teres major muscle inferiorly, and the proximal humerus laterally. The axillary nerve (C5, 6) originates from the brachial plexus, passes anterior to the subscapularis muscle, and then runs dorsally, together with the posterior humeral circumflex artery, through the quadrilateral space, continuing around the posterior aspect of the humeral surgical neck. The axillary nerve innervates the teres minor and deltoid muscles and the posterolateral cutaneous region of the shoulder and upper arm. The original description of the quadrilateral space syndrome referred to dynamic compression of the nerve due to extreme abduction. The nerve may also be stretched or contused by trauma, including recurrent glenohumeral dislocation, or be damaged or compressed by fractures of the proximal humerus or scapula, hematomas, axillary masses including paralabral cysts, hypertrophy of the teres minor muscle, or fibrous bands. Patients with quadrilateral space syndrome present with shoulder pain and paresthesia and may describe symptoms resembling intermittent claudication. When there is chronic injury to the nerve, it results in atrophy of the teres minor and, less commonly, the deltoid muscles. Symptoms of quadrilateral space syndrome may be mistaken for rotator cuff disease, cervical spine disease, or thoracic outlet syndrome.
MRI is the method of choice for imaging the axillary nerve and for detection of any surrounding soft tissue masses ( Figure 14-4 ). The quadrilateral space is best demonstrated on oblique coronal views. The nerve and vessels are well demonstrated on routine oblique coronal, oblique sagittal, and axial images of the shoulder. Under normal circumstances, the nerve shows no kinking or focal enlargement, the surrounding fat is intact, and no mass is present. In the subacute or chronic setting, edema and atrophy of the teres minor and, more rarely, the deltoid muscle, may be seen. Fatty atrophy of the teres minor is best identified on the oblique sagittal images.
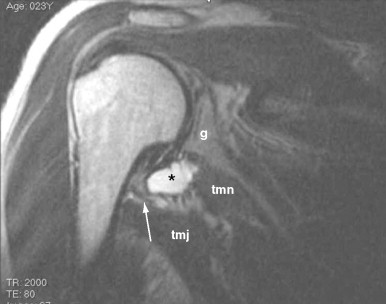
Quadrilateral space syndrome causes selective atrophy of the teres minor muscle and sometimes the deltoid muscle on MRI.
Radiography or CT can depict callus or bone tumors that may compress the axillary nerve. On conventional angiography and MR angiography, the diagnosis of dynamic compression of the nerve is supported by demonstration of a normal, patent posterior circumflex humeral artery that subsequently occludes with extreme abduction. The differential diagnosis for post-denervation changes in the teres minor and deltoid muscles includes Parsonage-Turner syndrome (acute brachial neuritis), an atraumatic self-limited condition, that causes severe shoulder pain radiating down the arm, followed by loss of motor function in the affected muscles and that lasts days to weeks. In Parsonage-Turner syndrome, the long thoracic and suprascapular nerves as well as the axillary nerve may be involved.
Radial Tunnel Syndrome and Posterior Interosseous Nerve Syndrome (Radial Nerve and Its Branches)
The radial tunnel refers to a space about the elbow approximately 5 cm long, extending from the capitellum to the distal edge of the supinator muscle. It is bounded posteriorly by the capitellum, anteromedially by the brachialis muscle, and anterolaterally by the brachioradialis (proximal) and extensor carpi radialis brevis (distal) muscles ( Figure 14-5 ). There are several potential sites of nerve compression within the radial tunnel : (1) fibrous bands that can tether the radial nerve or its branches to the elbow joint at varying locations; (2) the tendinous edge of the extensor carpi radialis brevis muscle; (3) the radial recurrent artery and its vascular branches (known as the leash of Henry ); (4) a fibrous band at the origin of the supinator muscle (known as the arcade of Frohse ); and (5) a fibrous band at the distal end of the supinator muscle.
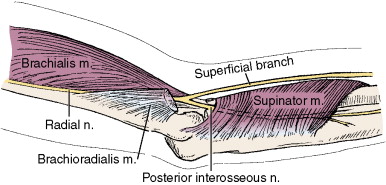
Within the radial tunnel, the radial nerve divides into the superficial branch of the radial nerve (SBRN) and the posterior interosseous nerve (PION). This division occurs distal to the lateral epicondyle of the elbow and proximal to the supinator muscle. The SBRN runs superficial to the supinator muscle and continues along the superficial aspect of the lateral forearm, providing sensation to the dorsoradial hand. The PION runs deeper in a plane between the deep and superficial heads of the supinator muscle. There the PION can be compressed by the arcade of Frohse, a fibrous band at the origin of the supinator muscle. The PION continues into the forearm, traveling deep, along the extensor surface of the interosseous membrane. The posterior interosseous nerve supplies motor innervation to the extensor-supinator muscles.
The terms radial tunnel syndrome and posterior interosseous nerve syndrome describe two distinct clinical syndromes that arise due to compression of the radial nerve or its branches in the region of the radial tunnel. Although these two syndromes can have similar causes, they may be distinguished by their differing clinical presentations. Radial tunnel syndrome presents predominantly with pain and occasional sensory disturbances but without significant weakness. Posterior interosseous nerve syndrome presents predominantly with motor weakness, but without significant sensory loss. The two syndromes can occasionally coincide, complicating the clinical diagnosis.
Patients with radial tunnel syndrome present with chronic aching pain over the lateral aspect of the elbow and proximal forearm. Symptoms can mimic lateral epicondylitis, and as a result, radial tunnel syndrome should be considered when suspected lateral epicondylitis fails to respond to treatment. The tenderness associated with radial tunnel syndrome typically occurs 6 to 7 cm distal to the lateral epicondyle. The differential also includes chronic extensor compartment syndrome and cervical radiculopathy. Some patients experience “double crush” phenomenon involving the cervical spine and radial tunnel. Patients with PION syndrome experience pain on the lateral side of the elbow, followed soon after by motor weakness. There is paresis or paralysis affecting extension of the metacarpophalangeal joints of the thumb and fingers, with inability to abduct the thumb. The wrist tends to deviate radially because of extensor carpi ulnaris paralysis.
Nerve entrapment leading to either syndrome may result from direct compression of the nerve by the structures of the radial tunnel detailed earlier. In this setting, activities that involve repetitive pronation and supination can lead to dynamic compression of the nerve; this phenomenon has been reported in violinists, music conductors, and swimmers. The radial nerve can also experience mechanical compression due to ganglion cysts, lipomas, vascular malformations, synovitis, bicipitoradial bursitis, swelling due to trauma, and radial head dislocation.
Radiographs can demonstrate fracture, dislocation, or bony abnormality contributing to radial nerve compression about the elbow. MRI can depict the nerves and surrounding musculature, as well as surrounding masses contributing to nerve compression, such as ganglion cysts, bicipitoradial bursitis, or synovial hemangioma ( Figure 14-6 ). The use of high-resolution ultrasound to depict PION impingement was described in a group of four patients with forearm weakness and pain. In that study, ultrasound successfully demonstrated enlargement of the deep branch of the radial nerve on the affected side in all four patients, a finding that was confirmed by electroneurographic testing and surgical testing. In this study by Bodner et al., the mean nerve diameter was 4.2 mm (transverse) by 3.3 mm (anteroposterior) in the symptomatic group, compared with 2.13 mm (transverse) by 1.3 mm (anteroposterior) in volunteers.
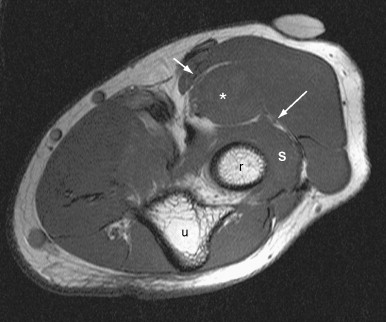
Cubital Tunnel Syndrome (Ulnar Nerve)
Cubital tunnel syndrome is the second most common entrapment neuropathy, after carpal tunnel syndrome. Within the cubital tunnel, the ulnar nerve (C8, T1) may be compressed at two separate, but nearby and related, locations ( Figure 14-7 ). The first site is within the sulcus on the posterior surface of the medial humeral epicondyle (the sulci nervi ulnaris). The arcuate ligament of Osborne (or cubital tunnel retinaculum) spans the sulcus between the medial epicondyle and the olecranon, creating a fibroosseous tunnel. The second site lies approximately 1 cm distal to the sulcus, where the nerve runs and between the two heads (ulnar and humeral) of the flexor carpi ulnaris muscle and the medial surface of the humerus. The two heads of the flexor carpi ulnaris originate from and are connected by the arcuate ligament of Osborne.
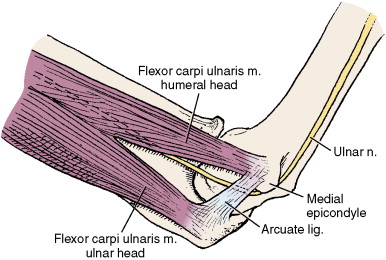
Symptoms of cubital tunnel syndrome may be caused by acute or chronic bony deformity, ganglion cysts, and other soft tissue tumors, synovitis, and nerve enlargement. Chronic repetitive microtrauma associated with elbow flexion/extension can cause edema and perineural scarring, resulting in nerve compression. Although subluxation of the nerve with flexion can occur and may predispose to friction neuritis, it is seen in 10% to 16% of asymptomatic normals and its actual role in giving rise to cubital tunnel syndrome is unclear. Recurrent traction on the ulnar nerve can lead to symptoms and can be seen in baseball pitchers, boxers, jackhammer operators, and assembly line workers. Dynamic compression of the nerve can occur because of marked decrease in the volume of the cubital tunnel in flexion. With flexion, the aponeurotic roof of the cubital tunnel is stretched and the medial collateral ligament bulges into the tunnel, reducing volume of the canal up to 50% of baseline and generating increased pressures within the canal. In some people, the ligament overlying the tunnel may be replaced by an accessory muscle—the anconeus epitrochlearis muscle—that can contribute to nerve compression. Because of its superficial location at the elbow, the ulnar nerve is also susceptible to external compression in this location. Causes of external compression include leaning on the elbow for long periods of time, application of a tourniquet, or positioning during surgery.
Patients with cubital tunnel syndrome present with paresthesias in the fifth and medial fourth fingers and can progress to muscle weakness and atrophy, with clawing of the fourth and fifth fingers. Symptoms can develop acutely in the setting of trauma. Physical exam may reveal tenderness over the medial epicondyle and a palpable enlarged ulnar nerve. Tinel’s sign may be elicited over the cubital tunnel. Symptoms of cubital tunnel syndrome may be confused with symptoms due to C8 radiculopathy, superior sulcus tumors, or thoracic outlet syndrome. Medial epicondylitis is also associated with localized tenderness to palpation along the medial elbow but can be distinguished because it is not accompanied by paresis or sensory changes.
Anteroposterior (AP) and lateral radiographs of the elbow can demonstrate bony changes about the cubital tunnel. The cubital tunnel view, taken with the humerus externally rotated and the elbow fully flexed and resting on the plate, depicts the cubital tunnel itself but is no longer commonly obtained. CT scans are used more routinely now for imaging the bony contours of the cubital tunnel.
The ulnar nerve is well seen on routine axial MR images of the elbow ( Figure 14-8 ). In cross section, the nerve appears as a round or oval structure that is isointense to muscle and that is surrounded by normal fat. Enlargement of the nerve and abnormal elevated T2 signal within the nerve at the level of the cubital tunnel suggests cubital tunnel syndrome ( Figure 14-9 ). These findings should be interpreted in conjunction with clinical history, however, as other causes of neuropathy can create this appearance. The arcuate ligament is visible on MRI and can be assessed for thickening. The recurrent ulnar artery also lines within the cubital tunnel, posterior to the nerve. Ganglion cysts, soft tissue masses, olecranon bursitis, or the normal variant anconeus epitrochlearis muscle can be diagnosed. The nerve may lie outside the canal due to subluxation or postoperative transposition. If ulnar subluxation is suspected but is not apparent on the conventional MR images, additional images with the elbow flexed can be obtained using a flexible or local surface coil.
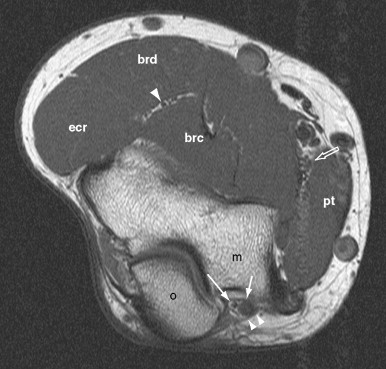
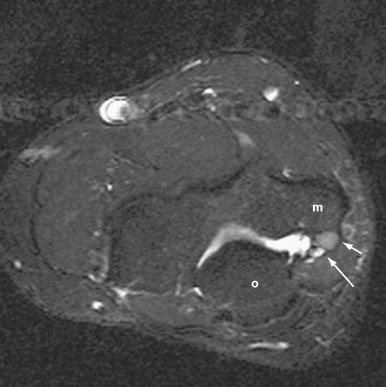
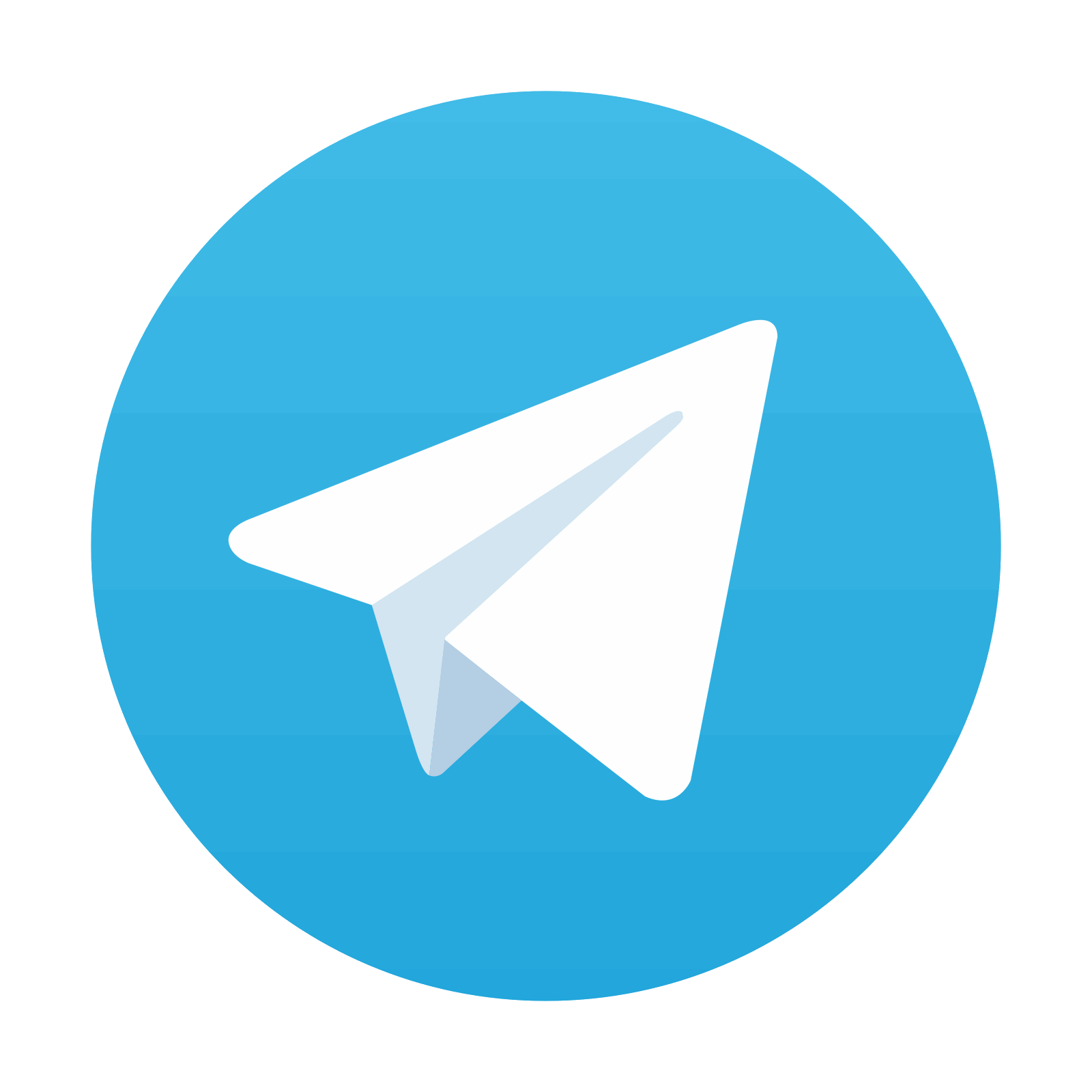
Stay updated, free articles. Join our Telegram channel

Full access? Get Clinical Tree
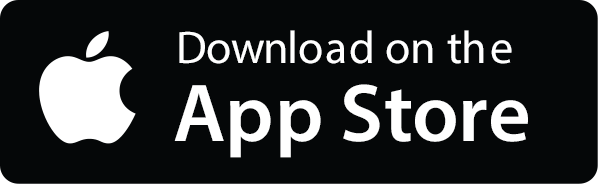
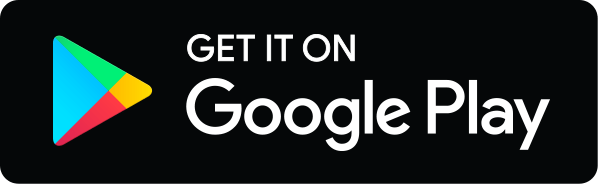
