An initial three-plane localizer sequence should be performed. This will demonstrate the cranial and caudal extent of the uterus, and can be followed with T2-weighted Half Fourier Single-Shot Echo (SSFSE/Haste/ssTSE/Fast FE) (Table 6.1-2), large FOV,36–48 thick (5 to 10 mm) sections through the entire uterus, coronal to the mother. This can be followed by T2w SSFSE or SSFP sagittal and/or axial planes of the maternal pelvis. These sequences will allow assessment of fetal lie, placental position and signal, cervix, and fetal situs. These sequences also help determine whether the surface coils need to be repositioned to get the best signal from the fetal anatomy in question.
At this point, there are several ways to get into a fetal orthogonal plane. Advanced technology allows user selection of three points within the images that will appear on the same plane in the next sequence. For example, two points can be selected in the lumbar spinal canal, and one at the umbilicus, which would result in a perfect sagittal stack. If this technology is not available, it is possible to pick two points that are symmetric on either side of the body, for example, the orbits and place the center “eye” of the stack on the one point, then page through the images until the second point is visible and rotate the stack so that the center line passes through the second point. This will result in images between axial and coronal, from which a perfect sagittal can then be obtained.50
Because the fetus continues to move throughout the study, each sequence is typically planned using a plane perpendicular to the prior sequence. Images of the head then body or vice versa should be protocoled depending on which anomaly needs to be evaluated first. In the sagittal and coronal planes, the entire head, chest, and abdomen can often be included particularly in earlier gestation. Dedicated axial sequences of the fetal brain and abdomen, however, are typically needed for optimal image interpretation. At times, the fetus will move but then quickly return to its initial position. Therefore, it can be helpful to simply repeat the sequence without changing parameters. If the fetus truly has moved into another position, the above techniques can be used to rapidly get another appropriate orthogonal plane.
Quiet maternal breathing is typically adequate; however, breath-hold techniques are needed for certain series, particularly T1w gradient-echo (GRE) sequences. Breath-holding can be useful if the fetus is breech, when the maternal diaphragmatic motion is more readily transmitted to the fetus. Breath-holding is successful only if the length of the sequence is relatively short, less than 20 seconds. Allowing the mother to gently release the breath-hold toward the end of the series can help if the sequence is longer than this.
Phase oversampling is often used in fetal MRI. It has the advantages of reducing the field of view to include just the fetus and exclude maternal anatomy by eliminating phase-wrap, and it also increases the SNR, which can be particularly important early in gestation when the fetus is small. The disadvantage is that it increases the scan time according to the number of phase-encoding steps. If this is problematic, the phase oversampling ratio can be reduced or turned off completely. This will result in phase-wrap, but with careful positioning and sizing of the field of view, often the maternal structures will only phase-wrap into the maternal structures on the opposite side of the body, and not overlie onto the fetal anatomy.
When scanning the fetus, a smaller 24- to 30-cm FOV should be used. Slices as thin as 2 to 3 mm can be obtained; however, the smaller the slice thickness, the lower the SNR. In larger fetuses, slice thicknesses of 4 to 6 mm work well, decreasing time per series and improving signal.
A typical matrix size is 256 px. Counterintuitively, increasing the matrix size in the attempt to increase in-plane resolution will typically have the opposite effect because of loss of signal from smaller voxels. This can be partly countered by increasing the slice thickness but results in more volume-averaging.
T2-weighted half Fourier single-shot echo sequences (SSFSE/HASTE/ssTSE/SsFSE/FASE) (Table 6.1-2) provide the highest SNR and resolution, and are fast.50 Slices as thin as 2 to 3 mm with no skip can be obtained. These T2-weighted images are most useful for anatomic detail, particularly of the fetal surface structures at the interface with amniotic fluid and the various body cavities that are fluid-filled such as the brain, ventricles, eyes, nasal cavities, oral cavity, airway, GI tract, and GU tract. Typically, axial, sagittal, and coronal images angled to the brain and then body should be obtained.
Heavy T2-weighted hydrography takes longer to acquire, but produces excellent SNR contrast. Slice thickness choices are dependent on gestational age; 3 mm works well at <24 weeks, and 4 mm at >24 weeks decreases the length of this longer sequence.51–53
Steady-state free precess (SSFP/FIESTA/TruFISP/Balanced FFE/Balanced SARGE/True SSFP) images demonstrate bright blood imaging. The cardiac chambers and great vessels may be discreetly identified. Images can be obtained in all three planes at 3- to 4-mm increments. This sequence is also quite useful for the assessment of fluid-filled structures such as dilated renal collecting systems or cystic masses. Because of its high contrast, inner ear anatomy, clefts of the palate, and fluid surrounding the spinal cord are particularly well demonstrated. Extremities are outlined by the amniotic fluid with fingers and toes often visualized. There is less contrast between gray and white matter in the brain and meconium in the abdomen due to lower flip angles and banding artifacts.
Thick slab T2w are useful for assessing fetal surface contours. Thick slab acquisitions of 40 to 60 mm give an overall three-dimensional (3D) effect. Transparency is increased using shorter echo times or by using SSFP sequences that can similarly be acquired with thick slices. To maximize SNR, overlapping slices of 5- to 6-mm thickness can be used; however, this approach typically makes this sequence less useful for volumetry. These images can be useful for parents and health care workers not used to evaluation of two-dimensional (2D) anatomy.54
T1-weighted sequences are fast multiplanar spoiled gradient recall acquisitions in the steady state (SPGR/FLASH/T1-FFE/Fast FE) sequences (Table 6.1-2) require breath-hold with thicker slices (5-7 skip 0) for sufficient SNR. It can be useful to perform a T1 sequence immediately after a T2 sequence with the same slice parameters so they can be directly compared. T1-weighted images are particularly useful for detecting hemorrhage, calcification, meconium, thyroid tissue, and fat.
Dynamic SSFP sequences (Cine, real time) can be used to assess fetal movements. Multiple frames per second can be obtained at each slice with slice thicknesses ranging from 7 to 50 mm. Assessment of fetal breathing, swallowing, cardiac motion, and gastrointestinal activity is possible. When there is a burst of fetal activity that precludes use of other imaging sequences, it can be a useful backup sequence. When the fetus settles down, other sequences can then be acquired.
Echoplanar imaging (EPI) is useful in assessment of the musculoskeletal system with high-signal cartilage and low-signal bone. The fetal liver has significant hypointense signal, while other organs are intermediate in signal. Flow voids make vessels and heart hypointense. Susceptibility-weighted imaging can be used to detect hemorrhage and calcification.
Long-Tau inversion recovery sequences can be useful to further characterize fluid that appears high-intensity on T2-weighted imaging, but that may contain proteinaceous fluid such as hemorrhage.
Diffusion-weighted sequences (DWI) can be acquired with three phase-encoding directions. The area of interest should be as centered as possible. DWI can demonstrate ischemic lesions in white matter. It can be used in the identification of renal tissue, particularly if kidneys are not visible sonographically or by routine MRI.
Advanced imaging with spectroscopy and diffusion tensor imaging is becoming available and is discussed in Chapter 6.3.
Whatever the indication for the fetal MRI, the entire fetus from head to toe should be examined. Fetal pathologies and malformations frequently involve multiple organs. Thus, identification of other anomalies can further elucidate an underlying genetic or chromosomal syndrome.
Because of the risk of maternal fatigue, anxiety, or discomfort, it is important to complete the study as quickly and efficiently as possible. Protocols should be well thought out prior to placing the mother on the scanner. If a brain anomaly is present, planes angled to the brain should first be obtained, then sequences angled to the chest and abdomen. Most protocols benefit from a combination of T2-weighted sequences (SSFSE and /or SSFP) in all three planes and T1-weighted imaging in at least one plane.
Postprocessing
Volumetry of fetal organs by MRI has become a useful tool, particularly in the determination of fetal lung volumes (see Chapter 17). A region of interest can be manually segmented and its total area multiplied by the slice thickness to calculate volumes. Continuous motion-free images are needed for accurate volumetry. Sequences may need to be repeated to ensure continuity of images if volumes are to be obtained. Spatial resolution of reformatted images can be limited owing to large slice thickness. Advanced methods to develop 3D volume images are being developed so that even when motion occurs, accurate data can be generated (see Chapter 6.3).6
Three-dimensional virtual model reconstruction using techniques such as stereolithography or fused deposition modeling can create life-size physical models using T2w MRI data.55,56
3 Tesla MRI
As the push for improved resolution and increased signal continues, and more 3 T scanners become available for use, centers have begun to address the use of 3 T scanners for fetal imaging. The use of 3 T magnets, however, represents another technical challenge with its increased susceptibility to motion artifact and the need to closely follow SAR limits.35,37
The US FDA approved the use of 3 T for human use in 2002. Advantages include improved SNR at higher strengths as more protons are available to increase magnetization. The gain of SNR may be up to 1.7 to 1.8 times that of 1.5 T.37,57 Image quality, temporal and/or special resolution can thus be improved. With greater SNR, parallel imaging can be implemented to speed SSFSE protocols, reduce echo time, and potentially decrease RF heating by decreasing the number of pulses required.37
With 3 T, unfortunately, more artifacts are encountered. RF field inhomogeneity is a major challenge, with the larger the field of view, the worse the artifact. RF shielding artifact “conductivity effect” from amniotic fluid results in blackout areas often where the fetus is lying. Dielectic pads, RF cushions, or saline pads can help decrease these dielectric resonance artifacts.37,58 Multichannel transmission coils, RF shimming with parallel imaging may also help decrease RF field inhomogeneity.
Magnetic susceptibility artifacts and chemical shift artifacts are also accentuated with 3 T requiring additional modifications. Changing the field of view, scan orientation, frequency, or bandwidth may move the artifact away from the fetal area of interest.
The US FDA limits of exposure to changing magnetic fields are independent of magnetic field strength. FDA safety limits prevent exposure to changing magnetic fields (dB/dt) of more than 60 T per second with failsafe software limiting scanners from exposures above these limits. With the fetus near the center of the FOV exposure, dB/dt changes are usually low with slew rates similar at both 1.5 and 3 T.37
RF pulses deposit energy into the imaged subject. The energy deposition is measured by the SAR reported in Watts per kilogram. The amplitude of applied RF fields increases with increasing magnetic field strength and quadruples from 1.5 to 3 T. Decreasing number of slices, lowering flip angles, increasing repetition time, and shorter echo train length can decrease SAR. The FDA safety limits are set at 4 W per kg independent of magnetic field strength with failsafe controls prohibiting additional exposure. Studies by Victoria et al.37 have demonstrated SAR well below whole body exposure limits for both 1.5 and 3 T.
Focal SAR hotspots are of concern because of RF field inhomogeneity, dielectric, and standing wave effects. Modeling of fetal environment by Hand et al.25,59 suggests that maximum energy deposited to the fetus is a fraction of that received by the mother. The fetus may be exposed to a peak of 50% to 70% of maternal SAR at 3 T. In their study, average temperature of the fetus remained below 38°C. However, they noted that continuous exposures over 7.5 minutes can exceed the 38°C limit, so long continuous exposures should be avoided.59
While 3 T has inherent artifact issues and potential increased safety risks, limiting SAR levels to those associated with 1.5 T and developing methods to decrease inhomogeneity appear to be feasible and will likely continue to progress.
Indications (Table 6.1-3)
Fetal MRI is a valuable complement to prenatal US. Because of higher contrast resolution, large field of view, and ability to image both sides of the fetus at once, fetal MRI can be more precise than US for anatomic evaluation. There are multiple indications for performing a fetal MRI, some of which are listed in Table 6.1-3. As MRI technology advances, additional indications will continue to develop, many described in the chapters in this book.
Fetal MRI Indications
Brain | Congenital anomalies such as ventriculomegaly, agenesis of the corpus callosum, holoprosencephaly, posterior fossa anomalies, cortical dysplasias, tuberous sclerosis, lissencephaly, microcephaly, family history |
Vascular abnormalities such as malformations, infarctions, hydranencephaly, twin-to-twin complications | |
Craniosynostosis Tumors Infection | |
Spine | Neural tube defects/vertebral anomalies |
Sacrococcygeal teratoma | |
Caudal regression/sirenomelia | |
Face and neck | Facial and palate clefts, micrognathia, dacrocystocele, anophthalmia |
Masses—lymphatic malformations, hemangioma, teratoma, goiter | |
Airway obstruction | |
Thorax | Masses—congenital pulmonary airway malformations, congenital diaphragmatic hernia, effusions |
Lung hypoplasia—oligohydramnios, skeletal dysplasia | |
Cardiac—heterotaxy | |
Abdomen | Masses/cysts |
Complex ventral wall defects | |
Genitourinary anomalies/cloaca | |
Bowel anomalies | |
Musculoskeletal | Limb anomalies |
Muscle abnormalities | |
Soft tissue masses—lymphangiomas, hemangiomas | |
Skeletal dysplasia | |
Twins | Monochorionic, twin-to-twin complications |
Conjoined | |
Fetal intervention/surgery Delivery planning Postnatal surgical planning | EXIT procedures for airway obstruction Congenital diaphragmatic hernia repair Myelomeningocele repair Complex lesions requiring immediate neonatal surgery |
Maternal | Placenta implantation abnormalities Poor evaluation due to obesity/oligohydramnios |
Maternal
Initial large FOV images are an opportunity to identify maternal abnormalities such as fibroids and ovarian pathology (Figs. 6.1-1 and 6.1-2). At times, maternal renal or skeletal anomalies are identified that should be described if noted. Pelvic inlet measures can be obtained as needed, but require large field of view pelvic images in all three planes for complete assessment of sagittal inlet and outlet, transverse inlet diameter, and interspinous distance.60–63
FIGURE 6.1-1: Fibroid. Sagittal SSFSE image of a gravid uterus demonstrates an anterior fibroid compressing the fetal head (curved arrow). Straight arrow points to the closed cervix.
FIGURE 6.1-2: Fibroid. Sagittal SSFP image of a gravid uterus demonstrates a large cervical fibroid (curved arrow) deep in the pelvis.
Placenta/Umbilical Cord
Placental location and thickness are well delineated by MRI (Fig. 6.1-3). When a placental anomaly is present, all three planes of the uterus should be obtained for adequate assessment.64,65 The placenta appears as an intermediate signal soft tissue structure along the margin of the uterus. The myometrial decidual interface is visible as a low-signal intensity line deep to the placenta.
FIGURE 6.1-3: Placenta previa. Sagittal SSFSE image of a gravid uterus demonstrates the placenta completely covering the cervix (curved arrow).
MRI has been used to evaluate placenta accreta with an overall sensitivity of 80% to 88% and a specificity of 65% to 100%.66–68 MRI features include uterine bulging, heterogeneous placental signal, and dark intraplacental bands.67,68 Some studies have used intravenous gadolinium contrast to assess for accreta, though the use remains controversial due to safety concerns. If contrast is being considered, the study should be timed as close to delivery as possible.64
Cervical length can be measured in the sagittal plane with location of placenta in relation to the cervix best noted on this view.
While MRI volumetry of amniotic fluid can be calculated, simple estimation of amniotic fluid is usually adequate.69 Uterine synechia and amniotic sheets may be well visualized in all three planes70 (Figs. 6.1-4 and 6.1-5).
FIGURE 6.1-4: Uterine synechia. Coronal SSFSE image of a gravid uterus at 21 weeks’ gestation demonstrates a thick band (curved arrow) along the lateral inferior uterus. No fetal entrapment was noted.
FIGURE 6.1-5: Amniotic bands. Numerous thin sheets (curved arrow) are adjacent to the fetal face. There were limb deformities, but no facial cleft was noted.
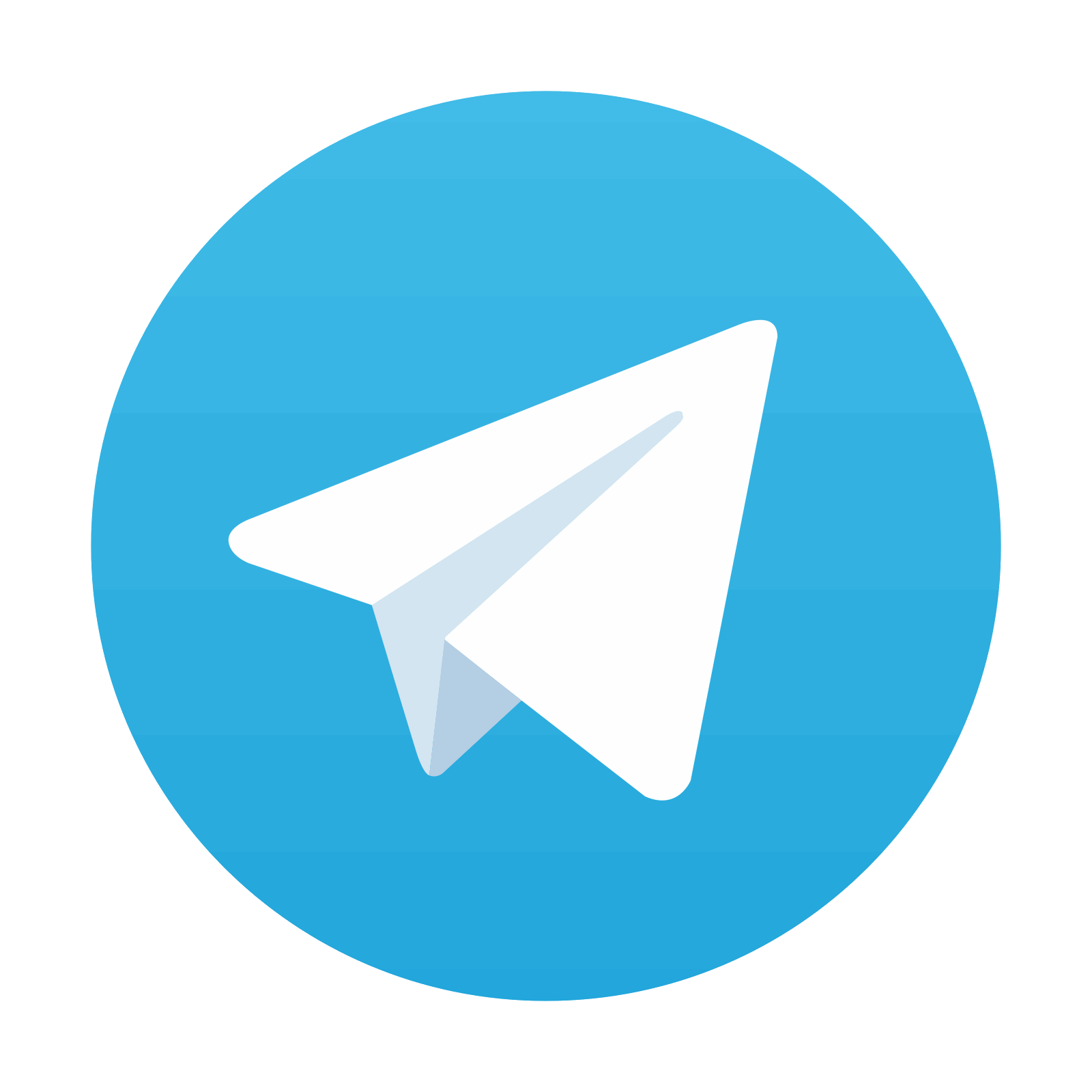
Stay updated, free articles. Join our Telegram channel

Full access? Get Clinical Tree
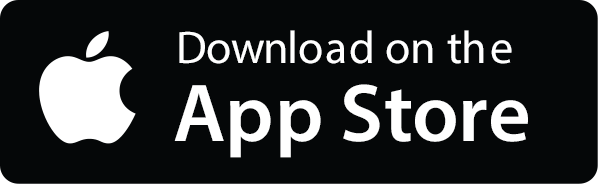
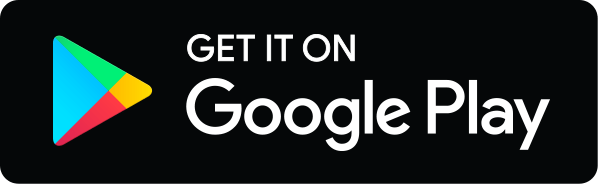