Fig. 11.1
Hydroureteronephrosis and deep venous thrombosis in a pregnant woman. (a, b) In the coronal (a) and axial (b) T2-weighted images (TR/TE ∞/95 ms), modest bilateral hydroureteronephrosis is visible, with dilated ureters that could be followed till the pelvis (arrows, b). A partial thrombosis of the left ovarian vein could be seen as a filling defect in the vascular lumen (dashed arrow, b)
Pyelonephritis is more common in pregnant than nonpregnant patients because of urinary stasis. While the diagnosis is usually made based on clinical parameters, MR imaging is useful to detect or exclude complications that require intervention such as perinephric abscess [8].
11.2.3 Gynecological Causes
Uterine leiomyomas demonstrate the same imaging characteristics during pregnancy as in the nongravid uterus. However, they may outgrow their vascular supply, resulting in degeneration, rapid growth, or torsion, leading to significant pain and premature labor. Most commonly, hemorrhagic infarction and necrosis will be seen with peripheral or diffuse high signal intensity on T1-weighted images and variable signal intensities with or without low signal intensity on T2-weighted images.
Subserosal fibroids can undergo torsion as well as degeneration during pregnancy.
When the gestational contents preclude accurate assessment with ultrasound, MRI is indicated. When multiple or large leiomyomas are present in the lower uterine segment, a decision may be made to perform cesarean section (Fig. 11.2) [9].
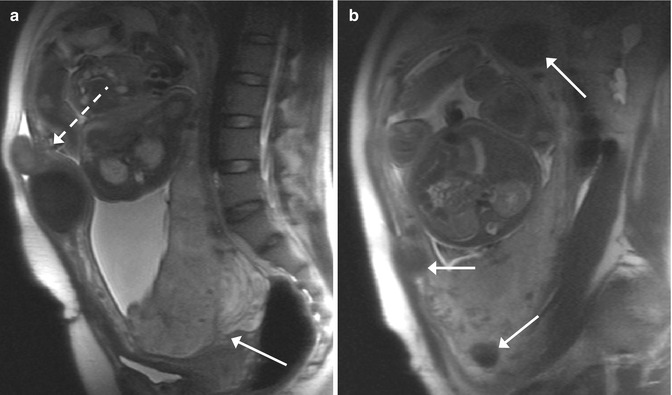
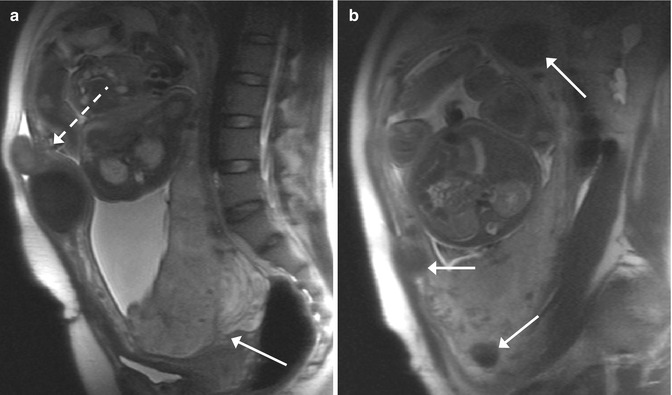
Fig. 11.2
Placenta previa and multiple fibroids in a pregnant woman (GW 32 + 4). (a, b) In the sagittal T2-weighted images (TR/TE ∞/95 ms), the placenta is inserted on the anterior and posterior wall of the uterus, and it covers the internal uterine orifice (arrow, a). Multiple fibroid intramural and submucosal tumors are visible (arrows, b). One subserosal pedunculated fibroid is localized in the ventral part of the body of the uterus (dashed arrow, a)
The diagnosis of an adnexal mass during pregnancy poses a diagnostic challenge. The most common adnexal mass in a pregnant patient is a corpus luteal cyst. It usually measures less than 6 cm in size and does not enlarge during pregnancy. MRI allows differentiation of simple cysts from more complex lesions, and the relationship between the mass and the pregnant uterus can be established. Because of the increased pressure in the pelvic cavity, adnexal masses may undergo extrinsic compression, hemorrhage, or torsion and lead to important abdominal pain (Fig. 11.3). Torsion is more common during pregnancy and may occur with or without an underlying mass. On MR imaging, a torsed ovary appears enlarged and edematous with increased stromal signal intensity on T2-weighted images [10].
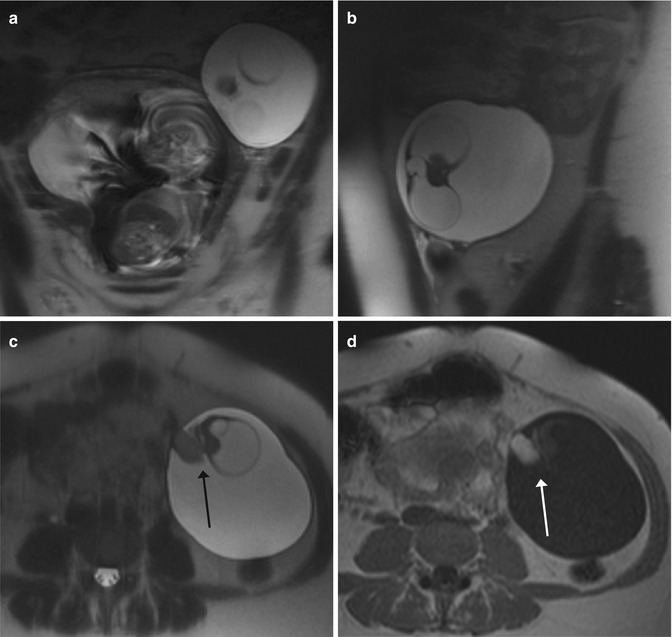
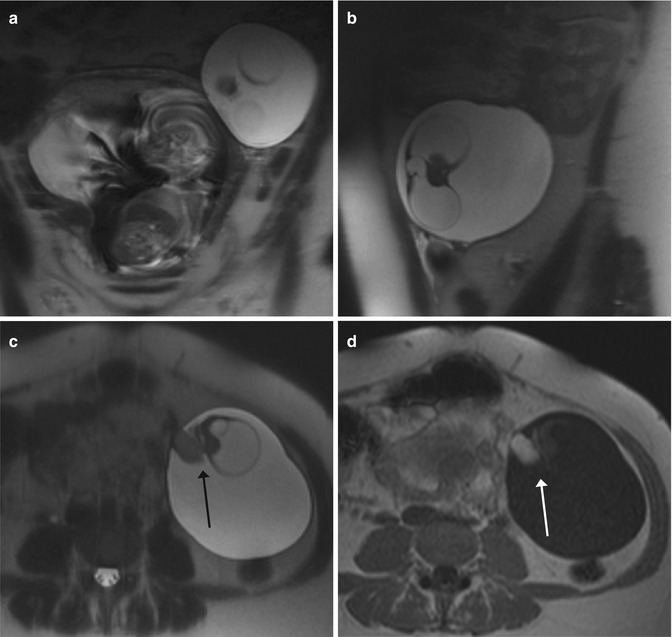
Fig. 11.3
Ovarian lesion in a pregnant woman. (a, b) Coronal (a) and sagittal (b) T2-weighted images (TR/TE 1,230/107 ms): the left ovary is entirely occupied by a cystic lesion. (c, d) Axial T2-weighted (c) and T1-weighted (d) images (TR/TE 141/4.7 ms): the lesion is well defined, with a prevalent cystic structure and an inner solid component (the Rokitansky nodule) (arrow, c) that presents the paramagnetic phenomenon (arrow, d). These characteristics permit the diagnosis of a dermoid cyst
11.3 Placental Imaging
MRI, because of its multiplanar capabilities, allows exact assessment of the placental position size and volume. It has been shown to be highly accurate in the diagnosis of placenta previa and might thus be an important adjunct to ultrasound in inconclusive cases. Placenta previa is diagnosed when the placenta covers a portion or all of the internal cervical canal and usually presents with painless vaginal bleeding during the course of the third trimester (Figs. 11.2 and 11.4). A related placental condition is placenta accreta, which is a leading cause of emergent peripartum hysterectomy. Placenta accreta is caused by lack of decidua basalis, which normally prevents villous invasion of the myometrium; any cause of uterine scarring, such as cesarean section or myomectomy, can lead to abnormal placental attachment. Prior cesarean section is by far the most common risk factor (Fig. 11.4). Three types of placenta accreta are described: placenta accreta vera (adherence to the myometrium), placenta increta (invasion into the myometrium), and placenta percreta (invasion of the uterine serosa). Because emergency hysterectomy is associated with substantial maternal morbidity and mortality, the prenatal diagnosis of invasive placenta is important. MRI is particularly helpful to determine the extent of placental invasion, which is critical for presurgical planning. Diagnosis depends largely on multiplanar T2-weighted images where the placenta demonstrates moderately high signal intensity and fine internal architecture. MR findings suggestive of placenta accrete vera include myometrial thinning, irregularity, or focal disruption, but the findings may be subtle, since no frank myometrial invasion is present. MR findings of the more severe placenta percreta include dark placental bands on T2-weighted images, focal thinning of myometrium, disorganized architecture of the adjacent placenta, focal exophytic mass, and in cases of anterior placental invasion involving the bladder, thinning of the uterine serosa-bladder interface [11, 12].
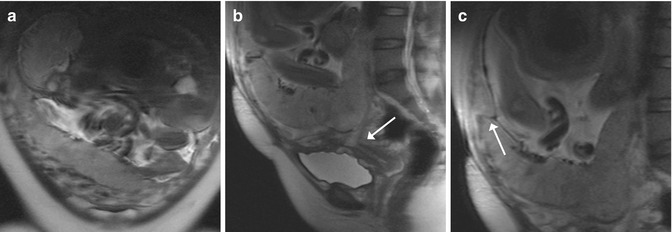
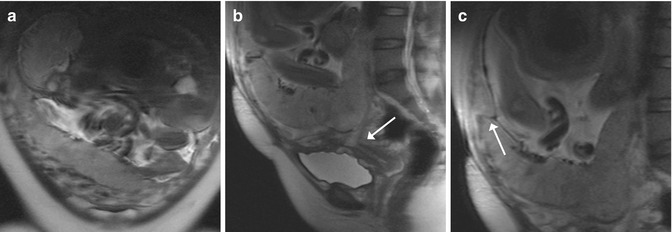
Fig. 11.4
Placenta previa and accreta (GW 30 + 5). (a–c) Coronal (a) and sagittal (b, c) T2-weighted images (TR/TE ∞/95 ms): unique fetus in podalic position and with his back turned toward the left side (a). The placenta is inserted on the anterior and posterior wall of the uterus, and it covers the internal uterine orifice (arrow, b). On the anterior wall of the uterus, the placenta shows an area of inhomogeneous signal (arrow, c) that corresponds to the site of previous caesarian sections. This is compatible with placenta accreta, confirmed after birth by the histological analysis of the uterus and placenta
11.4 Fetal Imaging
11.4.1 Central Nervous System
Because the fetal brain is a dynamic structure, it is important for radiologists to familiarize themselves with the normal appearance of it at different gestational ages in order to be better able to identify and characterize abnormalities with fetal MRI. Changes in fetal brain maturation proceed through different stages in a predictable fashion: by gestational weeks (GW) 12–23, the brain demonstrates a smooth surface except for the interhemispheric fissure. Sulci appear with this order: Sylvian and interhemispheric (GW 10–15); calcarine, parieto-occipital, and cingulate (GW 16–19); central and superior temporal (GW 20–23); precentral, postcentral, and superior frontal (GW 24–27); inferior frontal and inferior temporal (GW 28–31); insular, parietal, superior occipital, secondary frontal, secondary parietal, and secondary temporal (GW 32–35); and inferior occipital, tertiary frontal, and tertiary parietal (GW 36–39) (Fig. 11.5) [13]. Sulcation in the whole cerebral cortex is seen from GW 30 on, whereas infolding of the cortex and opercular formation will not be seen before GW 33. The developing nervous system has a multilayered appearance; till GW 28, from the surface to the depth, four layers could be recognized: the cortical plate, the subplate, and the intermediate and the ventricular zone that contains the germinal matrix. Afterwards, such distinction will fade away till the only distinction between white and gray matter (Fig. 11.6).
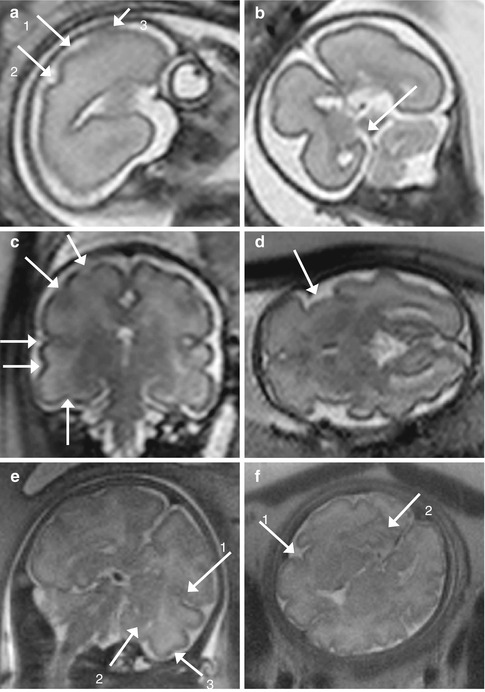
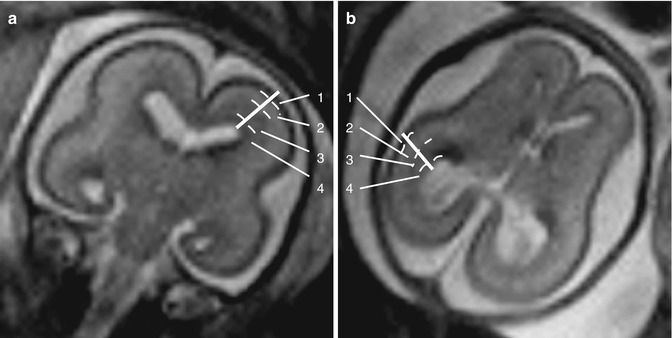
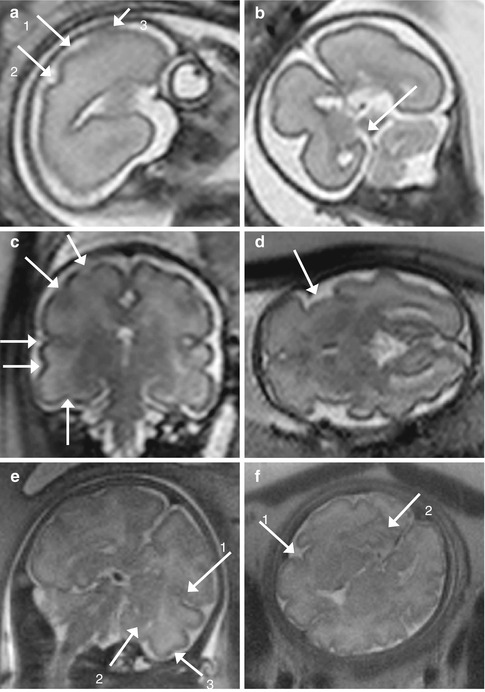
Fig. 11.5
Development of the sulci. (a, b) GW 24. In the sagittal (a) T2-weighted image (TR/TE ∞/95 ms), the central (1), the postcentral (2), and the precentral (3) sulci are visible. In the coronal (b) T2-weighted image, the calcarine sulcus (arrow) is visible. (c, d) GW 31. In the coronal (c) T2-weighted image (TR/TE ∞/95 ms), in counterclockwise direction, the precentral, the central, the lateral (of Sylvius), the superior temporal, and the occipitotemporal sulci are visible. In the axial (d) T2-weighted image (TR/TE ∞/95 ms), the Sylvian valley is visible. (e, f) GW 34. In the coronal (e) T2-weighted image (TR/TE ∞/95 ms), the inferior temporal sulcus is visible (3). The lateral (of Sylvius) (1) and the calcarine (2) sulci are also showed, as in the next axial (f) T2-weighted image (TR/TE ∞/95 ms)
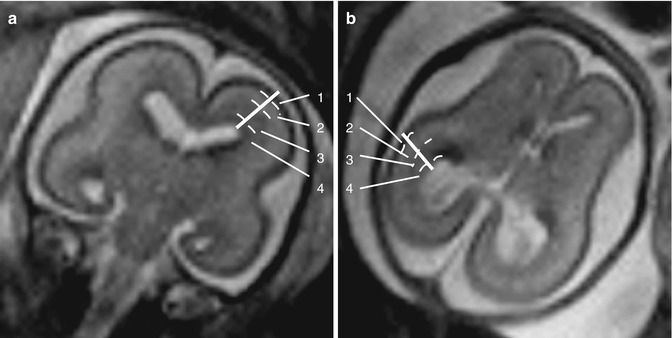
Fig. 11.6
Multilayered appearance of the developing nervous system at GW 24. (a, b) Coronal (a) and axial (b) T2-weighted images (TR/TE ∞/95 ms): from the surface to the depth, four layers could be recognized, the cortical plate (1), the subplate (2), the intermediate (3), and the ventricular zone (4) that contains the germinal matrix
The germinal matrix appears as a band of T2 hypointensity/T1 hyperintensity, for the high density of cells and microvessels, surrounding the ventricles in early gestation until the third trimester, when it gradually regresses to be present only in the caudo-thalamic groove at term. The cortical plate is seen as a band of T2 hypointensity/T1 hyperintensity at the periphery of the brain. The intervening parenchyma is of higher signal intensity on T2-weighted images but contains two visible layers as well, usually discernable between GW 20 and 28 [14].
By GW 23, cerebral ventricles appear relatively large, corresponding to the normal fetal hydrocephalus, gradually becoming smaller thereafter. The atria of the lateral ventricles remain relatively stable in size from GW 15 to 35, and the size should be less than 10 mm at the level of the atria when measured in an axial plane both by ultrasound and MRI. The cavum septi pellucidi is situated between the two lateral ventricles. It is a transient structure that remains visible till the end of pregnancy, and it has the same signal characteristics of the ventricles. Sonographic measurements of the third ventricle in the axial plane have revealed an upper limit of normal ranging from 1.0–1.2 mm by GW 12 to 3.5–3.6 mm at term, although no MR correlation has yet been performed. The cisterna magna may be difficult to visualize in the third trimester with ultrasound because of attenuation from the calvarium at the skull base; however, this is well visualized with MRI throughout the second and third trimesters. The subarachnoid space overlying the cortical convexities is slightly dilated at all gestational ages, most markedly by GW 21–26. The corpus callosum develops between GW 8 and 20. With MR, it is normally visible by GW 20 as a subtle T2 hypointensity. It forms in an orderly progression from genu to splenium, with the rostrum being formed last. The cerebellum, as the cerebral hemispheres, has a progressive development of the sulci. The first fissure that appears is the posterolateral one, by GW 12–13, and it divides the corpus cerebelli from the flocculonodular lobe. The development of the primary fissure that divides the anterior from the posterior lobe follows by GW 14–15, and subsequently, the prepyramidal, preculminate, and precentral fissures are seen by GW 15–16, and the horizontal fissure by GW 21. The foliation of the vermis starts in GW 14, and between 24 and 37 GW, the number of foliae in each lobule accounts for 50 % of the amount in adults. By GW 17, the primary fissure of the vermis should be visible, as should a normal fastigial point with an acute angle. The prepyramidal fissure is visible by GW 21, the preculminate fissure by GW 21–22, and the secondary fissure by GW 24. By GW 27, all vermian lobules and fissures are visible. The craniocaudal length of the vermis reaches that of the cerebellar hemispheres by GW 18–19. The vermis extends caudally to cover the roof of the fourth ventricle, completely covering it by GW 22–24.
The pons and the mesencefalus begin to differentiate themselves by GW 8. They appear hyperintense in T2-weighted images and hypointense in T1-weighted images in their ventral portion, but they become more and more hypointense in T2 and hyperintense in T1 in their dorsal portion, because of the progressive myelination of the ascending tract. The medulla oblongata contains ascending myelinated sensitive tracts, hypointense in T2-weighted images, and pyramidal tracts, whose myelination will begin in the postnatal period, which appear hyperintense in T2-weighted images [15].
The first role of fetal MRI is to explore the fetal brain anatomy to ensure that every structure is correctly developing and to make measurements on the fetal brain to ascertain that its development corresponds to the gestational age (Fig. 11.7). The obtained measurements could be compared with brain normal linear biometric values extrapolated by literature (Tables) [16, 17].
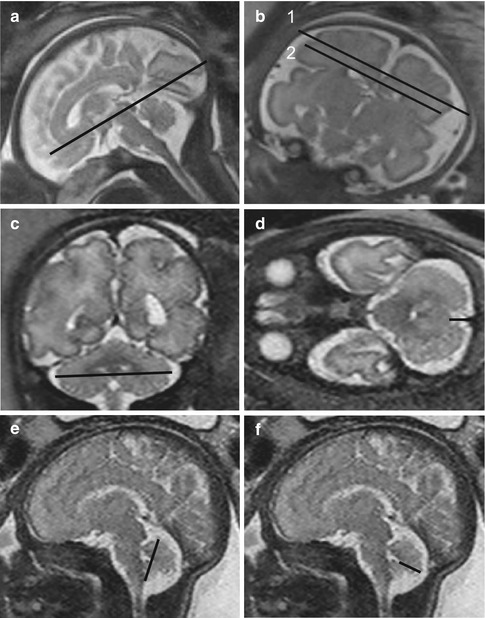
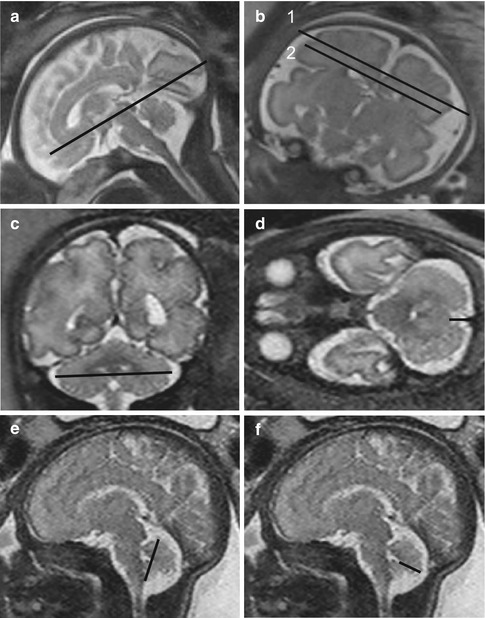
Fig. 11.7
Main linear measures useful to evaluate normal brain development. (a) Sagittal T2-weighted image (TR/TE ∞/95 ms): fronto-occipital diameter. (b) Coronal T2-weighted image (TR/TE ∞/95 ms): bone biparietal (1) and cerebral biparietal (2) diameter. (c) Coronal T2-weighted image (TR/TE ∞/95 ms): transverse cerebellar diameter. (d) Axial T2-weighted image (TR/TE ∞/95 ms): width of the cisterna magna. (e) Sagittal T2-weighted image (TR/TE ∞/95 ms): supero-inferior diameter of the vermis. (f) Sagittal T2-weighted image (TR/TE ∞/95 ms): anteroposterior diameter of the vermis
11.4.2 Ventriculomegaly
Ventriculomegaly (VM) represents the most frequent encephalic anomaly seen at fetal magnetic resonance imaging (MRI), with an incidence varying between 0.5 and 2 per 1,000 births per year. The frequency of associated malformations, both cerebral and extracerebral, varies between 41 and 78 % [18]. The causes of VM are as follows:
Anomalous turnover of cerebrospinal fluid caused by block to the ventricular system (i.e., expansive lesions, inflammatory and infective processes, hemorrhage) or by block to the reabsorption system (i.e., meningoencephalitis, subaracnoideal hemorrhage)
Anomalous cerebral development (i.e., corpus callosum agenesis) or neuronal disorders of proliferation or migration
Destructive processes (i.e., hemorrhages or infections)
Lateral ventricles are characterized by an anterior portion (frontal horn) and a posterior portion (atrium and occipital horn). In normal conditions, the ventricular cavity at the atrium is almost completely occupied by plexus choroid glomus. When a ventricle enlarges itself, the fluid accumulates between the choroid plexus and the medial wall, giving an image of dangling plexus. Ventricular measurement can be conducted on coronal images, placing calipers at the atrial level on the axis which is perpendicular to the ventricular one or on axial images and placing calipers at the luminal margins of ventricular atria, perpendicular to the long axis of the ventricle, at the level of the glomus of the choroid plexus. The measure is stable during the second and third trimester, with a medium diameter of 6–8 mm, and it is normal till a limit value of 10 mm.
VM can be both monolateral and bilateral; s can be both monolateral and bilateral, symmetric or asymmetric, and it is diagnosed when the atrial diameter (AD) of one or both ventricles is ≥10 mm (corresponding to 4 standard deviations (SD) above the mean) from 14 weeks of gestational age to term (Fig. 11.8). It is classified into three degrees of severity depending on the width of dilatation [19]:
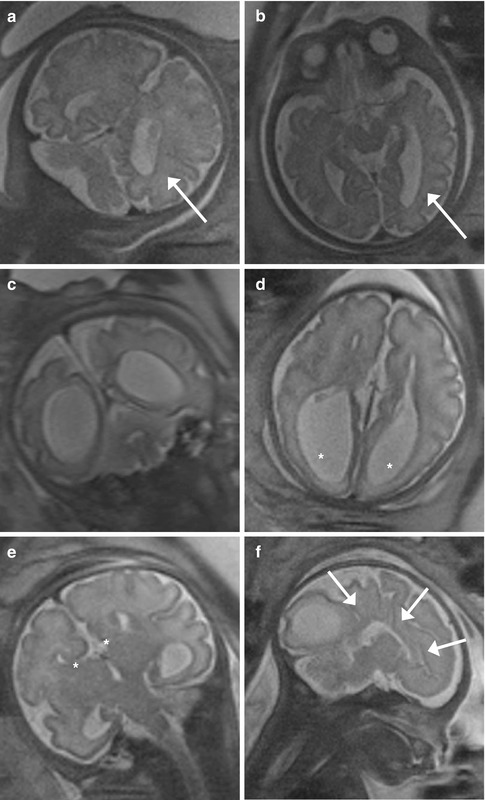
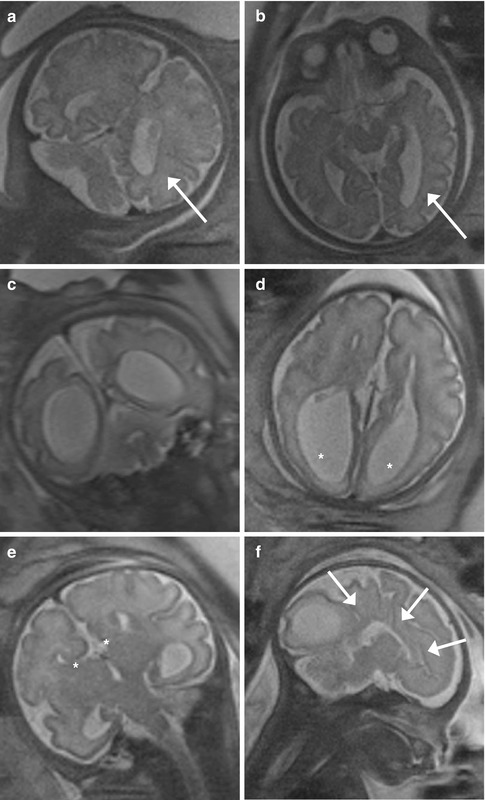
Fig. 11.8
(a, b) Mild ventriculomegaly (GW 35 + 2). In the coronal (a) and axial (b) T2-weighted images (TR/TE ∞/95 ms), mild unilateral dilatation of the left lateral ventricle is present (arrows), with an atrial diameter of 11 mm. The right one is normal, having a diameter of 9 mm. (c–f) Severe ventriculomegaly associated to complete agenesis of the corpus callosum (GW 32 + 1). Coronal (c, e), axial (d), and sagittal (f) T2-weighted images (TR/TE ∞/95 ms): severe bilateral dilatation of the lateral ventricles is present (c, d) with the right atrial diameter of 22 mm and the left one of 19 mm. The ventriculomegaly is associated to complete agenesis of the corpus callosum and of the cavum septi pellucidi, best demonstrable in the coronal image (e). Other secondary signs of corpus callosum agenesis are the parallel aspect of the lateral ventricles (d), the extreme distance between frontal horns (d) and their internal concavity (asterisks, e), the enlargement of atria and occipital horns (asterisks, d), and the radial disposition of the sulci on the mesial hemispheric surface (arrows, f)
Mild (or borderline): 10 mm ≤AD ≤12 mm
Moderate: 13 mm ≤AD ≤15 mm
Severe: AD >15 mm
The factors that most affect the prognosis of a fetus with VM are width of dilatation (mild, moderate, severe), presence of associated anomalies (structural, chromosomal), and an underlying etiological factor (i.e., infections, alloimmune thrombocytopenia) [20]. More than 60 % of cases of severe VM are associated with structural anomalies (Fig. 11.8). Prognosis for severe VM associated with other malformations is unfavorable, with a 2-year survival rate not exceeding 16 %. Prognosis of isolated severe VM is slightly better, with a 33 % survival rate at 2 years and normal neurological and physical development in 10–62.5 % of cases, depending on the study [21]. Moderate VM is associated with other anomalies in 10–50 % of the cases, whereas mild VM is associated in a very low percentage of cases. Prognosis is conditioned by the existing concomitant anomalies. Regarding the prognosis of isolated forms, a delay in neurological development can be found in 25 % of cases of moderate and in up to 7 % of cases of mild VM [22].
Another factor influencing the prognosis of isolated VM, either moderate or mild, is the evolution of dilatation over time. VM progression occurs in 16 % of cases, whereas stabilization is reported in 43 % of cases, and an in utero normalization occurs in 41 % of cases. Progression is associated with a 22 % rate of chromosomal anomalies and with a negative prognosis in 44 % of cases in comparison with 1 and 7 %, respectively, of the forms that do not progress (stabilization or regression) [23].
11.4.3 Medium Line Malformations
11.4.3.1 Holoprosencephaly and Absence of the Cavum Septi Pellucidi
Holoprosencephaly refers to a large group of cerebral malformation with a common embryogenesis, with an incomplete separation of the prosencephalus in right and left hemisphere. There are three principal forms:
Lobar holoprosencephaly (separated lateral ventricles)
Semilobar holoprosencephaly (partially separated lateral ventricles)
Alobar holoprosencephaly (lateral ventricles fused in one)
There is another form, syntelencephaly, that involves parietal and frontal lobes, and it seems to have a different embryonal origin.
RM is especially useful in the evaluation of difficult cases of holoprosencephaly and syntelencephaly, to identify a small fusion of frontal lobes or to make the differential diagnosis between holoprosencephaly and microcephaly. RM is also useful to identify eventual associated anomalies [24].
Absence of the cavum septi pellucidi is seldom isolated; it is usually acquired, secondary to a disruptive process such as endoventricular hemorrhage or infective process, or associated to a malformative process, such as holoprosencephaly or septo-optic dysplasia. In cases of no visualization of the folders of the septi pellucidi in US, MRI can confirm the absence of such structures, which can be appreciated in every plane. The inferior dislocation of the fornices, a consequence of the absence of septi pellucidi, is better seen in sagittal sequences. MRI is especially indispensable to search eventual associated anomalies or possible causes of the malformation [25].
11.4.3.2 Abnormalities of the Corpus Callosum
The prevalence of abnormalities of corpus callosum is 0.3–0.7 % in general population and 2–3 % in population with developmental delay. They can be associated to other malformations, either cerebral or extracerebral, including at least 46 malformative syndromes and metabolic disorders.
Fetal MR is useful in the evaluation of sonographically suspected callosal anomalies because the corpus callosum can be directly visualized in the sagittal and coronal planes as a curvilinear T2 hypointense structure located at the superior margin of the lateral ventricles, superior to the fornices. Direct visualization of the callosum by sonography, however, is more difficult, and thus, sonography typically relies on indirect signs of abnormal callosal development (such as absence of the cavum septi pellucidi, colpocephaly, which is a selective ventriculomegaly of the occipital horns more than the frontal or temporal horns of the lateral ventricles and inferior orientation of the medial hemispheric sulci) to identify abnormalities of callosal formation. These indirect signs can also be seen with fetal MRI (Fig.11.8) [26]. On fetal MR, the corpus callosum is best assessed using thin (3 mm) midline sagittal images. Its length can be measured on midline sagittal images, and measurements can be compared with published normative data, especially in cases of suspected callosal hypogenesis [27].
Fetal MR has been reported to have a greater detection of callosal agenesis as compared with prenatal US. In addition, fetal MR can identify an intact corpus callosum in approximately 20 % of cases referred for sonographically suspected callosal agenesis or hypogenesis, which has significant implications for patient counseling. Additional callosal abnormalities, including hypogenesis (or partial agenesis), dysgenesis, and hypoplasia, can also be diagnosed by fetal MRI. Because of the normally thin appearance of the fetal corpus callosum, callosal hypoplasia is more difficult to diagnose, especially during the second trimester.
Fetal MRI is important in detecting additional abnormalities in cases of callosal agenesis. Indeed, detection of associated brain anomalies by fetal MR is greater than by prenatal sonography in these cases, and additional anomalies are detected by fetal MR in up to 93 % of cases. The identification of additional findings by fetal MRI may suggest a specific disorder or syndrome associated with callosal agenesis; this has implications both for the current pregnancy and for the recurrence risk in future pregnancies. Thus, when fetal MRI is performed for cases of suspected callosal agenesis, the supratentorial structures as well as the infratentorial structures should be carefully examined [28].
11.4.4 Posterior Fossa Abnormalities
11.4.4.1 Chiari II
Chiari II malformation is an important indirect sign of open spinal dysraphism (myelomeningocele and myelocele) (Fig. 11.9). Each of these can occur with or without spinal cord splitting with an incidence of 0.5–1 in 1,000 live births. The position of the neural placode with respect to the level of the skin surface differentiates myelomeningocele from myelocele. When there is elevation due to expansion of the subarachnoid space, the lesion is called a myelomeningocele (MMC). Axial images demonstrate the posterior osseous and skin defect, through which the neural placode protrudes, secondary to expansion of the underlying subarachnoid space that is covered by a thin membrane.
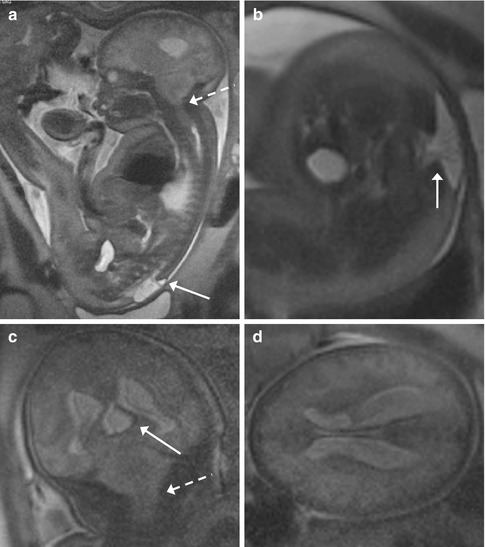
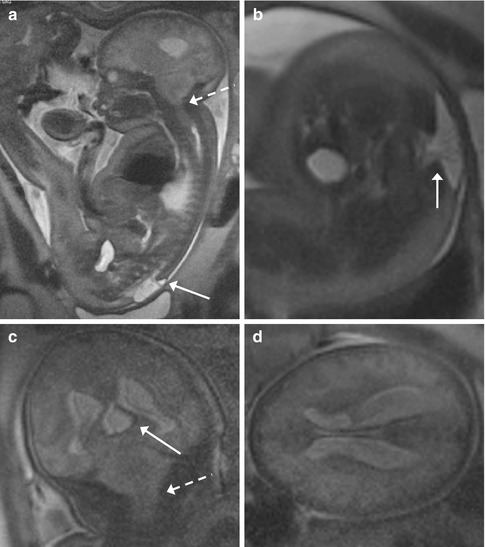
Fig. 11.9
Lumbosacral myelomeningocele with Chiari II and triventricular hydrocephalus (GW 35 + 3). (a–d) Sagittal (a), axial (b, d), and coronal (c) T2-weighted images (TR/TE ∞/95 ms): open spinal dysraphism at lumbosacral level, with herniation from the spinal canal of the meninges and nervous structures that come in touch with the cutaneous plane (arrows, a, b). A Chiari II malformation is also present, with herniation of the cerebellar tonsils and of the brainstem through the foramen magnum into the spinal canal (dashed arrows, a, c). This implies the reduction of the dimensions of the posterior cranial fossa, with more caudal insertion of the tentorium and disappearance of basal cisterns and of the fourth ventricle (a, c). An obliteration of the supratentorial subarachnoid spaces is also demonstrable (a, c, d). A secondary severe dilatation of the third ventricle (arrow, c) and of both the lateral ventricles is present
Myelocele (myeloschisis) is the term used when the placode is in plane with the level of the back without an overlying membrane-covered fluid-filled sac. This is much less common than the MMC but is embryologically similar. Only the expansion of the subarachnoid space distinguishes an MMC from a myelocele. The clinical manifestations and functional impairments are similar in these two entities. The localized failure of neural tube closure can occur anywhere along the spinal cord but is most common in the lumbosacral region [29].
The association between open spinal dysraphism and Chiari II is proposed to be due to the leakage of cerebrospinal fluid via the spinal defect that collapses the primitive ventricular system and prevents expansion of the rhombencephalic vesicle. This may result in a small posterior fossa with medulla, vermian, and cerebellar tonsil herniation. It is important to remember that in some cases, the findings may be mild and may progress; thus, close follow-up and correlation with alpha-fetoprotein and karyotype is important. With US, the so-called banana sign permits to discover the anomaly, but it cannot help to establish the entity of the problem. Through sagittal images, MRI is helpful to determine the entity of cerebellar herniation, which is directly correlated to the neurological outcome. MRI permits to identify other typical findings of Chiari II malformations, such as the lemon sign (which refers to the shape of the fetal skull when the frontal bones lose their normal convex contour and appear flattened in axial images at the level of the ventricles) and the American eagle sign (angled aspect of the lateral ventricles), to evaluate lateral ventricular dimensions and cerebral parenchyma. T2 signal is in fact increased in fetuses with Chiari II malformation, because of an increase of extracellular water, such as demonstrated by apparent diffusion coefficient calculation [30].
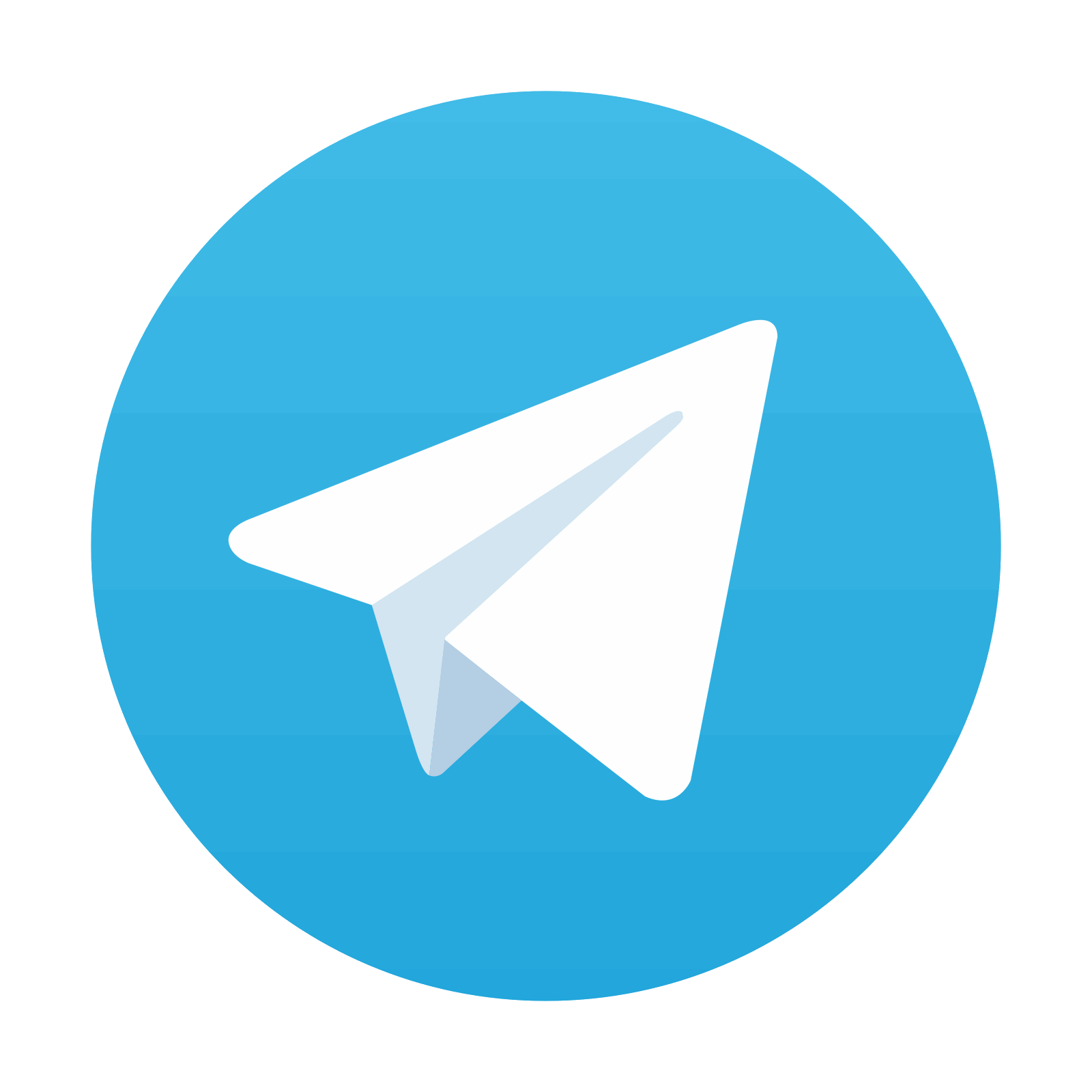
Stay updated, free articles. Join our Telegram channel

Full access? Get Clinical Tree
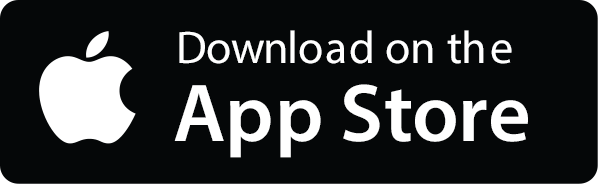
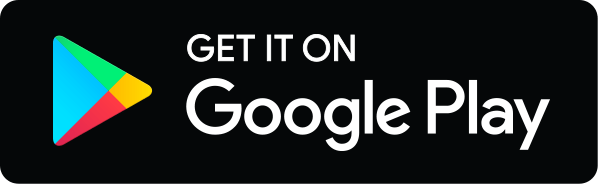