Knowledge of functional neuroanatomy is essential to design the most appropriate clinical functional MR imaging (fMR imaging) paradigms and to properly interpret fMR imaging study results. The correlation between neuroanatomy and brain function is also useful in general radiologic practice, as it improves the radiologist’s ability to read routine brain examinations. Functional MR imaging is used primarily to determine the areas involved in functioning of movements, speech, and vision. Preoperative fMR imaging findings also play a key role in the neurosurgeon’s decision to perform a biopsy, a subtotal resection, or a maximal resection using awake craniotomy.
Key points
- •
Knowledge of functional neuroanatomy is essential to design the most appropriate clinical fMR imaging paradigms and to properly interpret fMR imaging study results.
- •
The correlation between neuroanatomy and brain function is also very useful in general radiologic practice, as it improves the radiologist’s ability to read routine brain examinations.
- •
Functional MR imaging is used primarily to determine the areas involved in functioning of movements, speech, and vision, which are of the greatest interest in presurgical studies.
- •
Preoperative fMR imaging findings also play a key role in the neurosurgeon’s decision to perform a biopsy, a subtotal resection, or a maximal resection using awake craniotomy.
Introduction
Knowledge of functional neuroanatomy is essential to design the most appropriate clinical functional MR imaging (fMR imaging) paradigms and to properly interpret fMR imaging study results. This article reviews the foundations of brain anatomy by discussing the functional areas of the brain, focusing on the regions involved in movement, language, and vision, which are of the greatest interest in presurgical studies.
The correlation between neuroanatomy and brain function is also very useful in general radiologic practice, as it improves the radiologist’s ability to read routine brain examinations. Research has demonstrated that experience in fMR imaging improves accurate identification of anatomic structures, and that familiarity with fMR imaging reports enables radiologists to provide valuable information in conventional MR imaging reports. Identifying the precise locations of lesions and understanding their relation to major functional areas and the involvement of the eloquent cortex contribute to good surgical planning and positive postoperative outcomes. Basic skill in functional brain imaging may particularly improve the quality of work performed by radiologists involved in cancer care. Conventional brain scans of oncologic patients frequently show edema and postoperative changes that may lead to distortion of expected brain anatomy. In addition, it is important to remember that the presence of pathologic conditions such as seizures or tumors can affect the functional organization of the brain (see separate article, elsewhere in this issue).
Primary sensorimotor cortex
Specific Anatomic Structures
The primary motor and sensory cortices occupy the pericentral region of the brain, which consists of 2 parallel gyri: the precentral gyrus (preCG) and postcentral gyrus (postCG), which are separated by the central sulcus (CS) ( Fig. 1 ). The CS extends superiorly toward the medial interhemispheric surface of the brain and inferiorly toward the Sylvian fissure. At the upper end of the CS, the preCG and postCG fuse together to form the paracentral lobe. At the lower end of the CS, the preCG and postCG also fuse together to form the subcentral gyrus, which is located just above the Sylvian fissure.
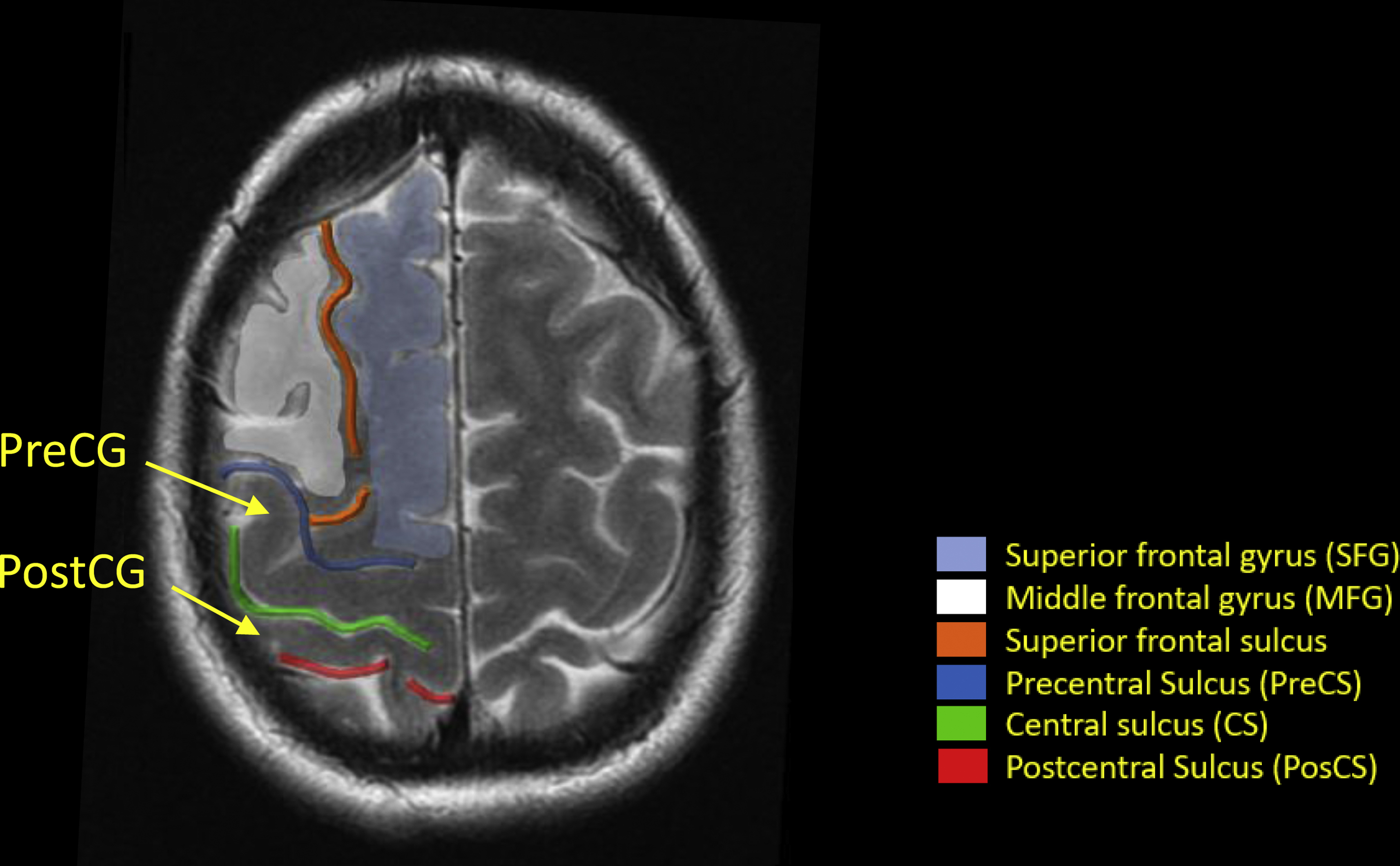
Fig. 1 presents anatomic landmarks of the frontal and parietal lobes that facilitate the localization of the preCG and postCG on conventional MR imaging images , :
- 1.
Superior frontal gyrus (SFG): Horizontally oriented, roughly rectangular, forms the uppermost margin of the frontal lobe.
- 2.
Middle frontal gyrus (MFG): Horizontally oriented, undulant shape, usually merges with the anterior face of the preCG.
- 3.
Superior frontal sulcus (SFS): Separates the SFG from the MFG; at its posterior end, the SFS bifurcates to form the superior precentral sulcus.
- 4.
Precentral sulcus (PreCS): Anterior margin of the preCG.
- 5.
Postcentral sulcus (PosCS): Posterior margin of the posCG.
It is important to bear in mind that these sulci are frequently discontinuous and imagining the path they generally follow may help radiologists to identify important regional structures.
Anatomic Organization
The sensory and motor systems are both organized topographically at the precentral and postcentral gyrus, respectively. This organization means that each portion of the body has a specific location on the cortex, , which is represented in a topographically organized map known as the “homunculus.” Because the amount of cortex represented in the homunculus is proportional to the degree of precision or discrimination necessary for optimal function of its representative body part, the resultant maps are distorted to emphasize the face, lips, fingers, and hands.
The classic version of the motor and sensory homunculus places the foot and leg in the superior aspect of the preCG directly adjacent to the interhemispheric fissure with a small region for the trunk lateral to the legs, followed by regions representing the fingers and hands. The most inferior and lateral segments represent the lips and face. , The hand motor area is another important anatomic reference for localization of the primary motor cortex, and is located at the most posterior aspect of an area with an upside-down omega shape in the preCG known as the “reverse omega” (Ω) ( Fig. 2 ). Combining localization of the hand motor area with previous knowledge of the specific parts of the motor homunculus helps radiologists to predict which motor function may be compromised when a lesion is present in the preCG and to choose which fMR imaging paradigm should be performed on each patient.
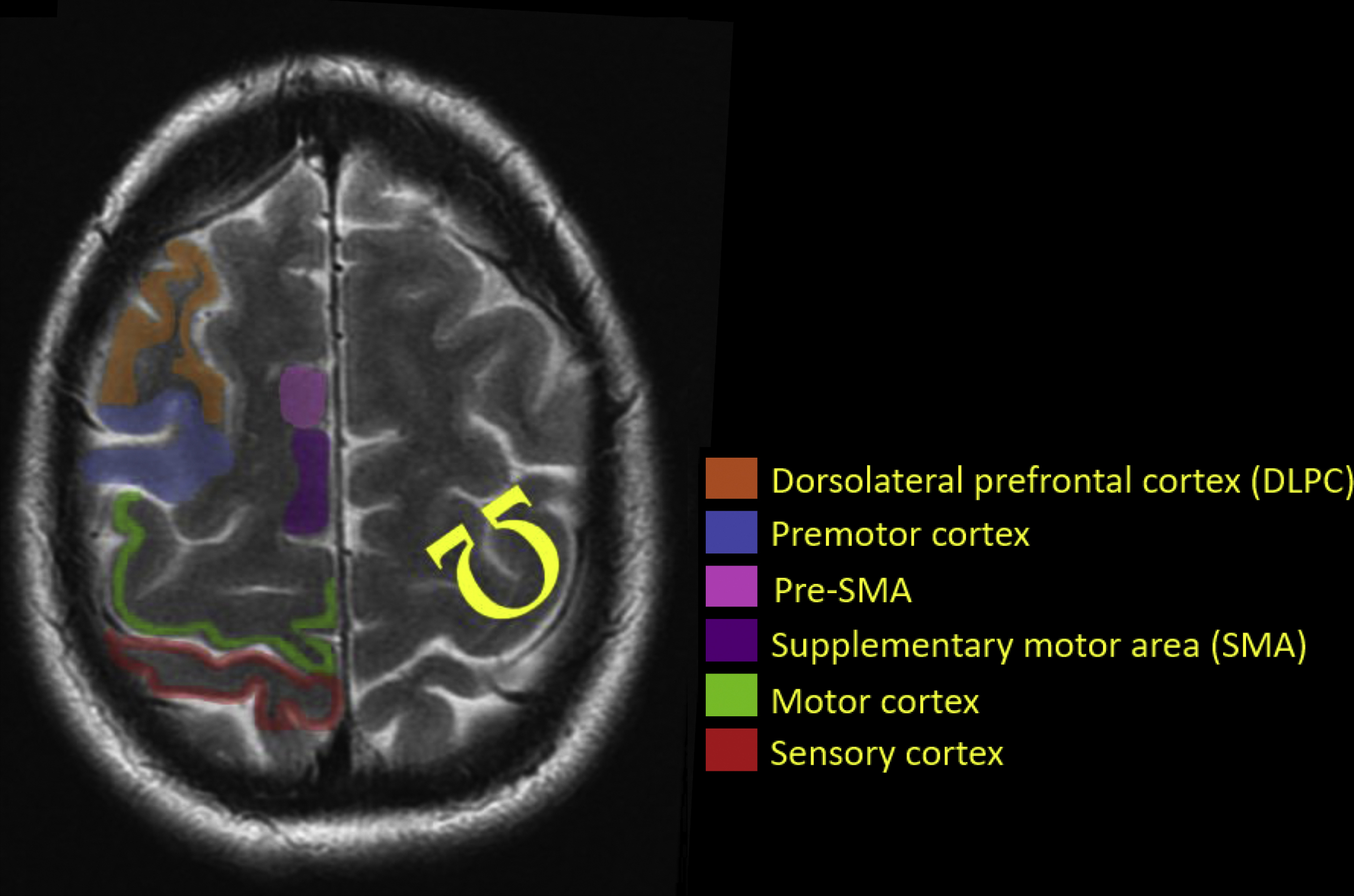
In cases in which anatomy is significantly distorted due to edema, mass effect, or postoperative changes, accurate identification of the “reverse omega” may be extremely difficult ( Fig. 3 ). In such cases, visualization of functional activation in the presumed hand motor area plays a key role in defining which motor tasks may be compromised and should be presurgically evaluated. It is also important to understand that the “functional” part of the preCG (the part that is actually involved in generating movement) is located on the posterior bank of the preCG. This true both physiologically and in fMR imaging ( Fig. 4 ).
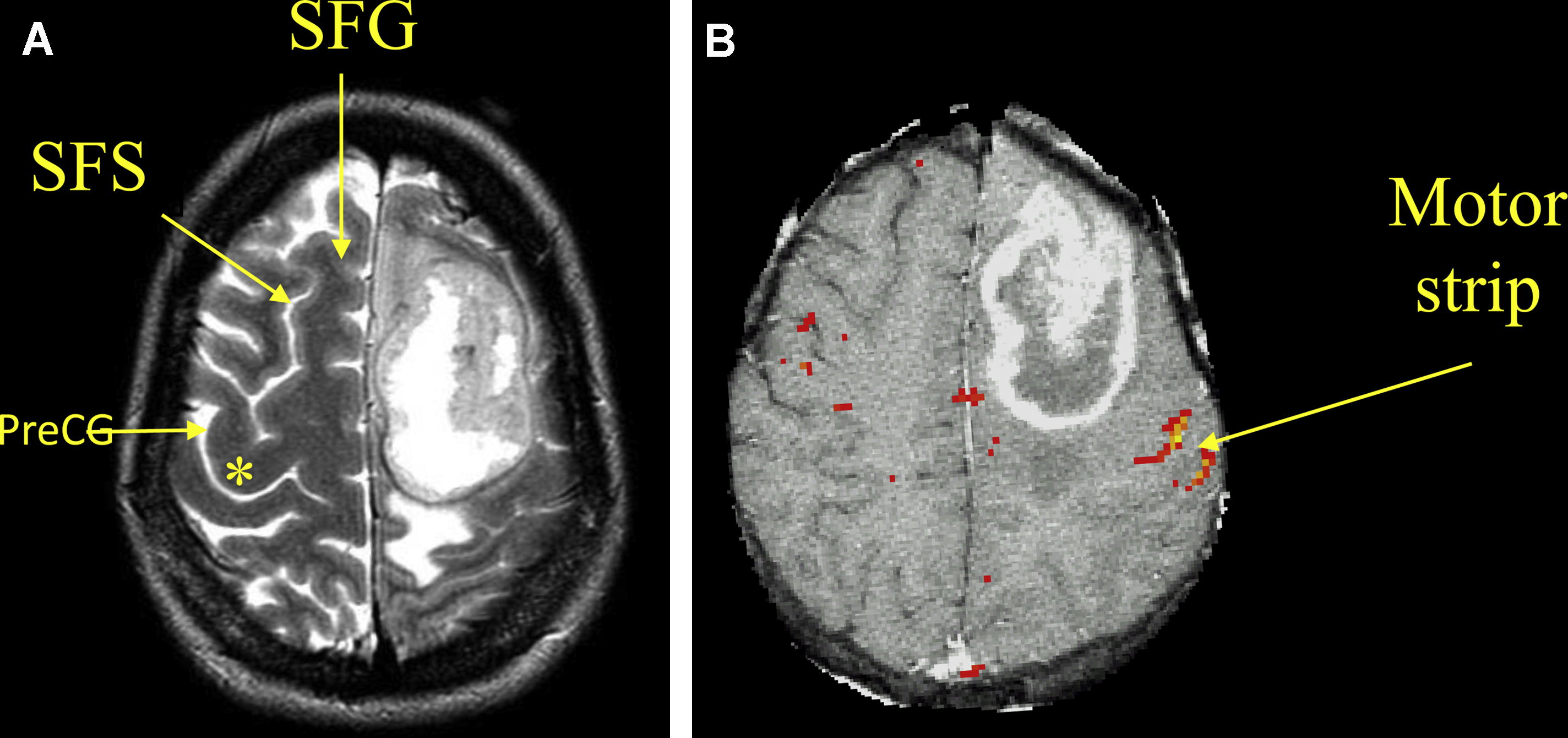
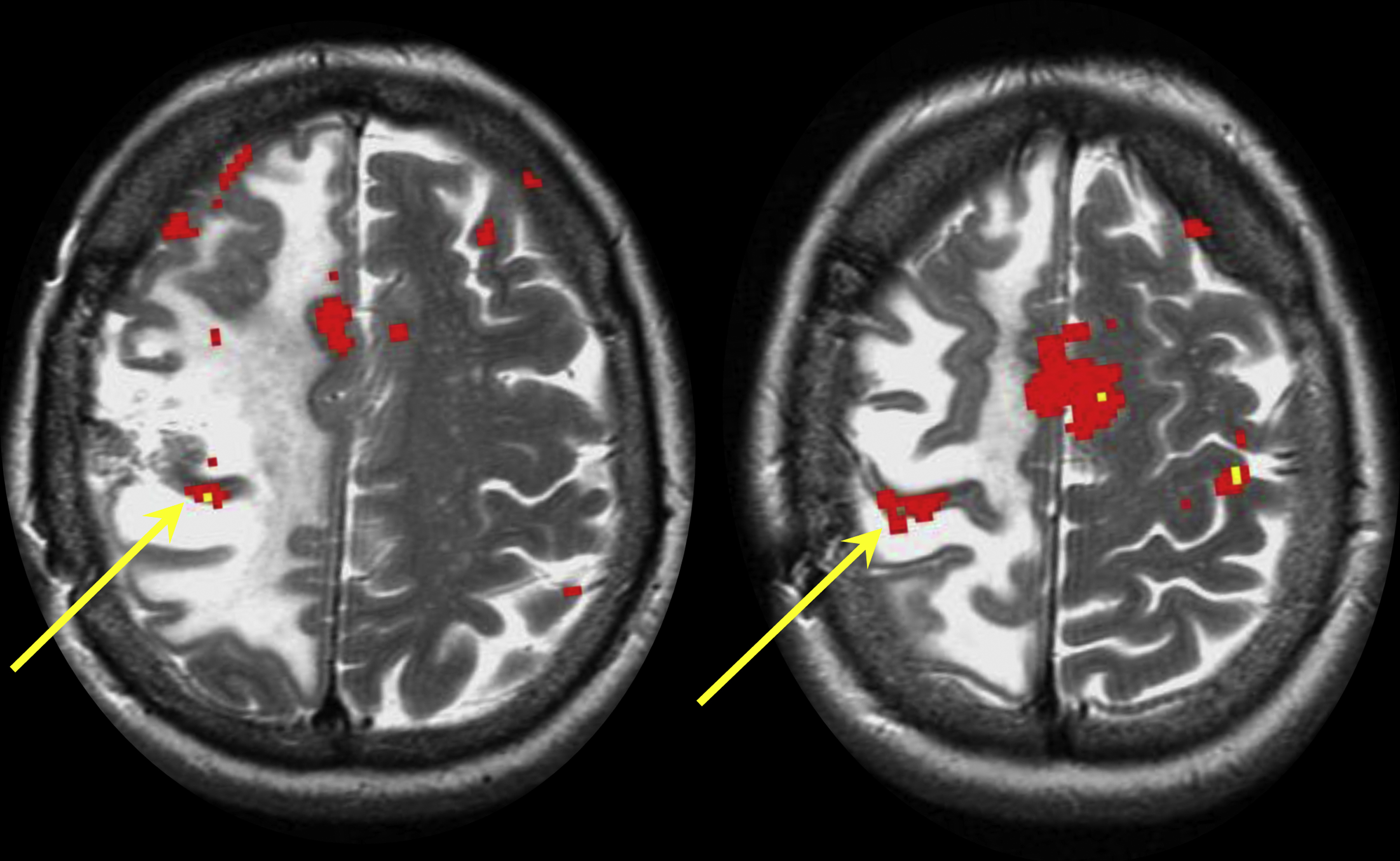
Primary motor cortex
The primary motor cortex initiates voluntary movement through the corticospinal tract, which also receives contributions from the premotor cortex, supplementary cortex, and somatosensory cortex. A large percentage of corticospinal tract fibers cross in the pyramidal decussation and connect to motor neurons in the contralateral spinal cord to trigger movement. The motor cortex also connects to the cerebellum and brain stem. Through this network, subcortical regions and other cortical areas influence the input and output of the primary motor cortex. A lesion in the primary motor cortex generally results in contralateral motor weakness.
Primary somatosensory cortex
The central processing of tactile and nociceptive stimuli takes place in the primary somatosensory cortex. Fine-touch and proprioception inputs reach these cortical areas through the dorsal column–medial lemniscus pathway, and information regarding pain, temperature, and touch reach these cortical areas through the lateral and ventral spinothalamic tracts. , Early areas of activation after tactile and nociceptive stimuli are located in the postcentral gyrus ( Fig. 5 ). The primary somatosensory cortex simultaneously receives information from other cortical regions and subcortical areas that modify the output response from this region, similar to the operation of the primary motor cortex. ,
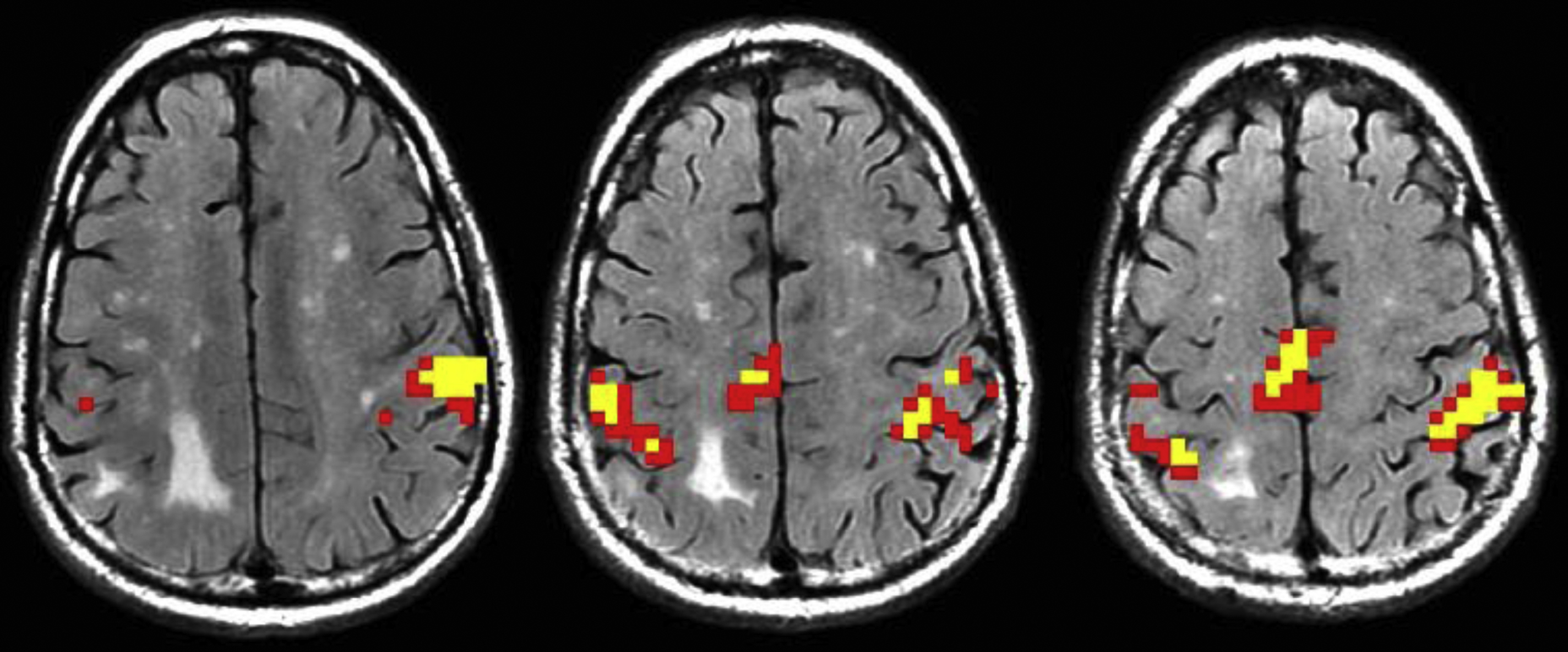
Although a complex and hierarchical organization within the somatosensory cortices occurs in response to tactile stimuli, nociceptive impulses activate a single area in the somatosensory cortex and are immediately redirected to the primary cortex and to temporal lobe limbic structures. This difference in the organization of both modalities may reflect that pain perception requires reactions to and avoidance of harmful stimuli rather than sophisticated sensory capacities.
Foot motor area
The localization of the foot motor homunculus in the presurgical setting is of extreme clinical importance, especially in patients with brain tumors in the frontoparietal region. Iatrogenic damage to the foot motor area and resultant paresis of the leg can render a patient wheelchair-bound or bed-bound. These conditions may be more debilitating than paresis of the nondominant hand or arm, which are commonly mapped by fMR imaging for surgical planning. By contrast, corticobulbar fibers from the contralateral hemisphere often compensate for iatrogenic compromise of the face and tongue homunculus.
The foot motor homunculus is located at the high frontoparietal convexity of the brain in the most medial aspect of the preCG and directly adjacent to the interhemispheric fissure. As this area is usually tucked under the superior sagittal sinus, it is quite difficult to approach intraoperatively and to confirm the exact area of foot motor activation by direct cortical stimulation. Therefore, fMR imaging plays a key role in correctly identifying the foot motor area in the preoperative setting ( Fig. 6 ).
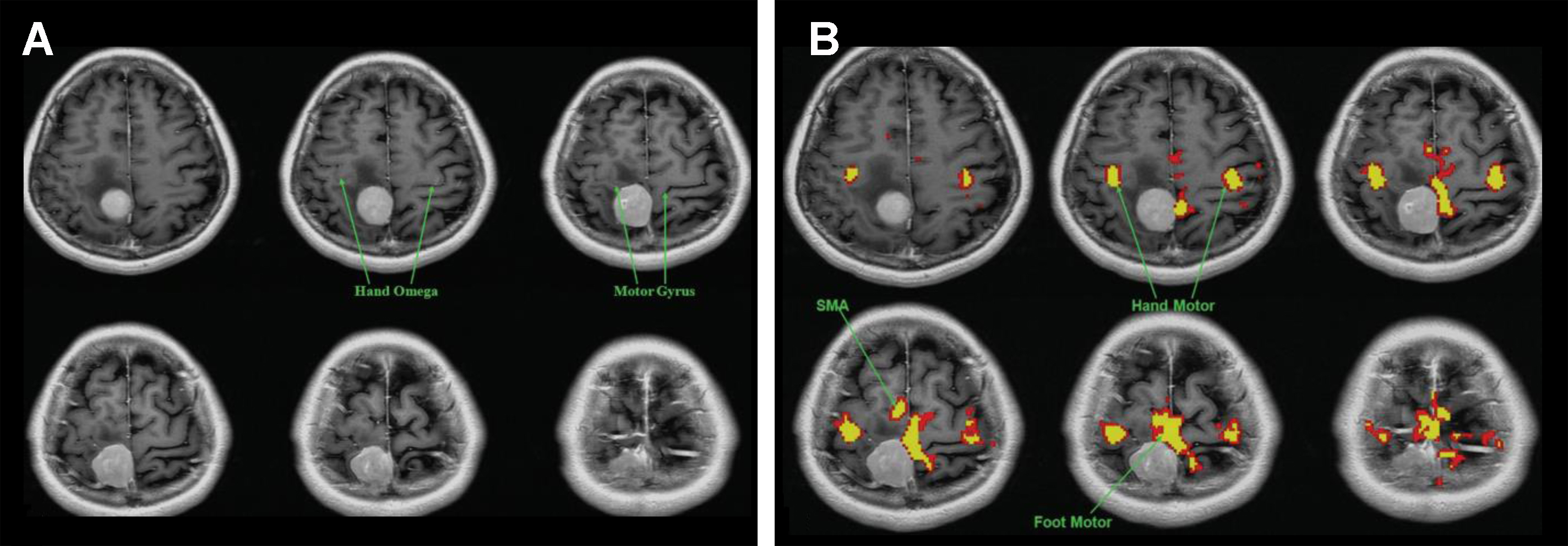
Accurate delimitation of the foot motor area on conventional MR imaging is limited due to a lack of a discernible anatomic landmarks. The CS, which may help guide readers, often does not reach the hemispheric fissure. Furthermore, there is no sulcus between the foot motor homunculus and the supplementary motor area (SMA), which makes the distinction of the foot motor area from the SMA even more difficult on routine MRI.
Another great difficulty in determining the location of the foot motor area is related to its high position in the brain, close to the convexity. Small displacements of the patient’s head during image acquisition often result in significant differences in the position of regional anatomic references that guide readers, such as the “reverse omega” ( Fig. 7 ). Finally, in patients with cancer, the presence of edema imposes an additional challenge. Even if an expansile lesion is not located in the foot motor area, the surrounding edema in the white matter can compromise foot motor function ( Fig. 8 ).
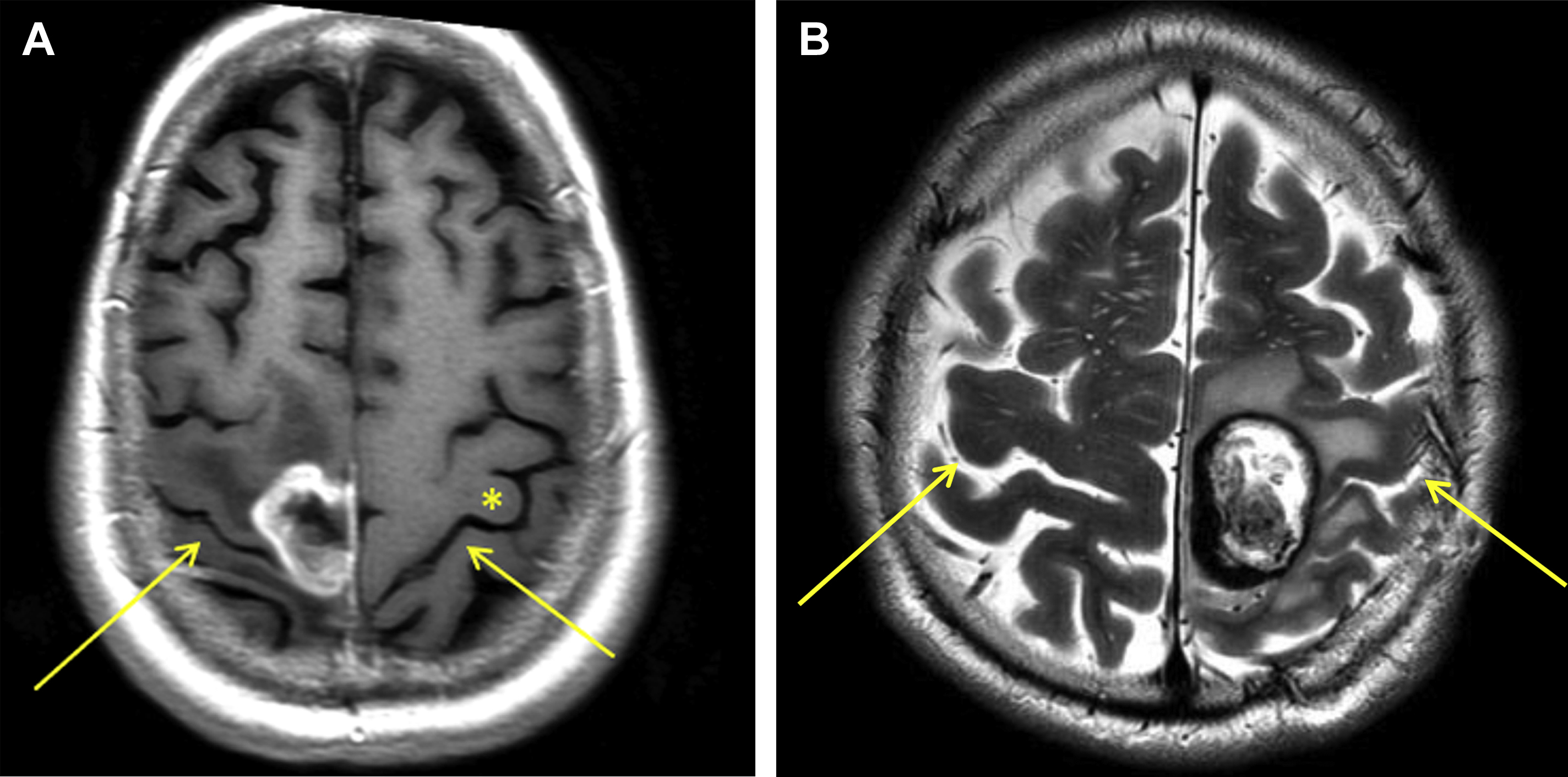
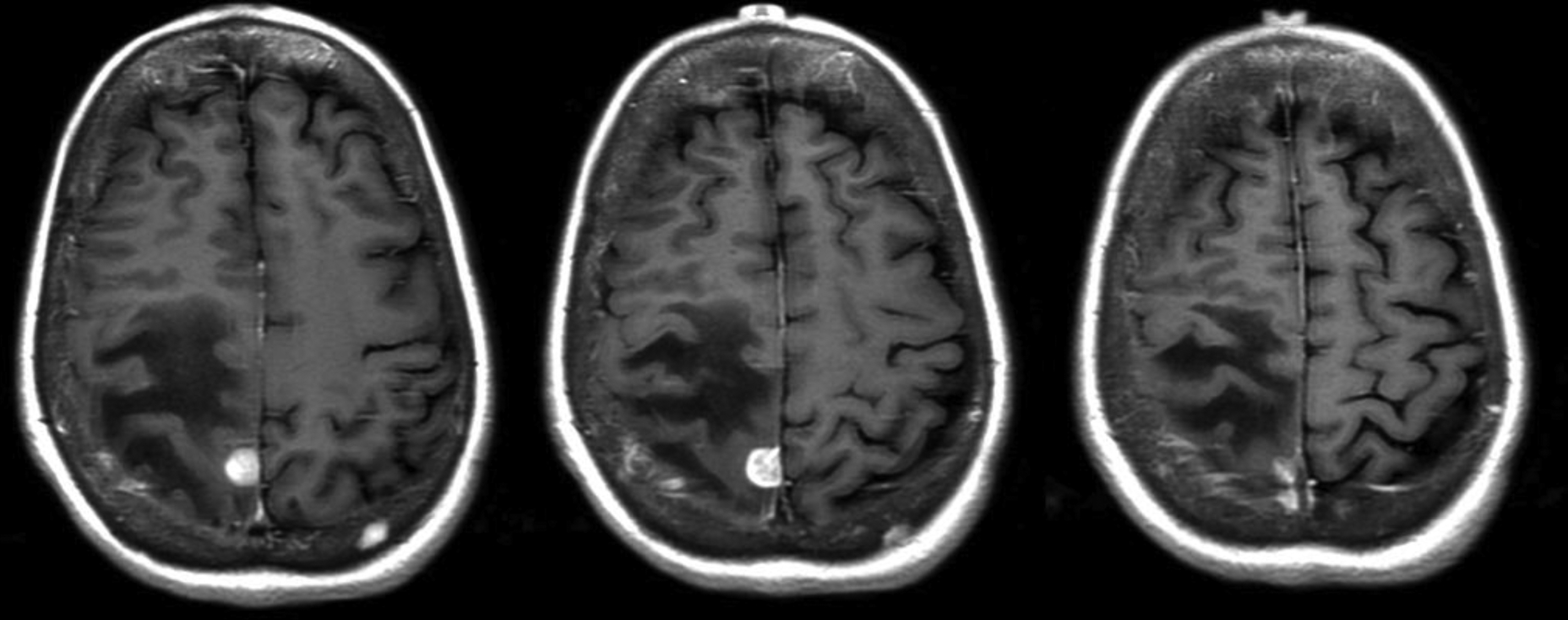
Fisicaro and colleagues demonstrated that precise localization of the foot motor area is difficult even among experienced readers. In a study of neuroradiologist and nonradiologist readers, they found that identification of the foot motor homunculus on conventional MR imaging of patients with brain tumors was correct only 77% of the time. None of the readers, including highly experienced neuroradiologists, were correct 100% of the time, which suggests the utility of preoperative fMR imaging. In addition, readers with prior fMR imaging experience and neuroradiologists fared significantly better than readers without such expertise.
Facial motor area (tongue and lips)
The classic version of the motor homunculus places the facial motor area at the most lateral level of the preCG, in a region known as the inferior ventral precentral gyrus. , Tongue and lip movements are frequently involved in facial motions such as spoken language, mastication, and swallowing, which may be compromised in patients with injuries or lesions in the frontotemporal junction.
Preoperative tongue motor-mapping with fMR imaging is of particular interest in patients with speech apraxia and difficulty finding words. In these cases, identification of the tongue motor area is important to guide intraoperative awake language mapping, in which surgeons stimulate the motor cortex to establish a current threshold and map language localization. The primary aim of the fMR imaging in such cases is in fact language-related.
The bilateral angular gyrus, supramarginal gyrus, and parts of the operculum in the frontal and temporal lobes have been demonstrated to be common areas of activation for facial and tongue movements. Other brain areas are also involved in facial movement within a complex facial motor network, such as the primary motor cortex (M1), the SMA, the insula, and the cerebellum. These areas seem to be highly activated during facial motor tasks and to modulate facial motor output.
Supplementary motor area
Not all patients with lesions in the perirolandic cortex are referred for motor strip localization. Many patients with medial frontal lesions undergo preoperative fMR imaging to localize the SMA. This area is located in the posterior aspect of the SFG; however, its anatomic boundaries are vague, making preoperative localization with fMR imaging essential. Even with fMR imaging, accurate identification and lateralization of the activated SMA can be challenging, as its midline position, its lack of specific anatomic structure, and the smoothing of fMR imaging data impose additional difficulties in mapping this area.
The SMA has traditionally been divided into 2 major areas: the SMA proper (motor SMA) and the pre-SMA (language SMA), which is located just anterior to the SMA ( Fig. 9 ). However, it is important to bear in mind that their functions are nuanced, their borders are indistinct and functionally variable, and they likely represent a continuum rather than discrete anatomic areas. ,
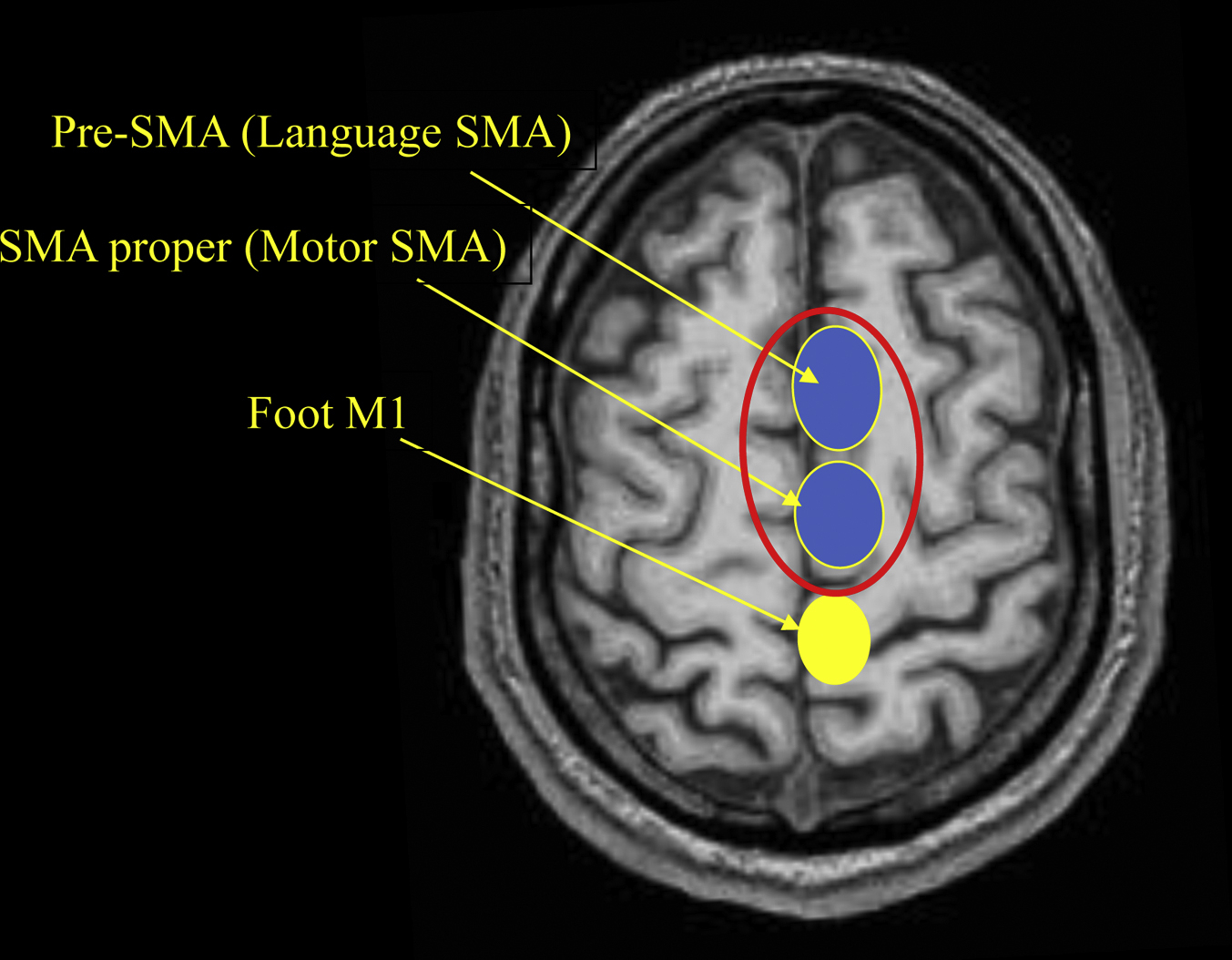
The SMA proper is responsible for planning motor movements, and the pre-SMA is responsible for linguistic planning, which is further discussed in the “language” section of this article. Evidence indicates that the SMA also plays a role in other functions, such as sensation, speech expression, listening comprehension, posture, and working memory.
General Anatomic Borders
The anatomic boundaries of the dorsomedial functional regions are poorly defined. The SMA occupies the dorsomedial aspect of the SFG, which is located immediately anterior to the foot motor representation. The SMA is bounded medially by the interhemispheric falx, laterally by the premotor cortex, and inferiorly by the cingulate sulcus and cingulate gyrus. The lateral and posterior borders of the SMA consist of the PreCS, which separates the SMA from the leg area in the primary motor cortex. There are no sharp borders between the medial posterior aspect of the SMA and the foot motor region. The anterior margin of the SMA is also less demarcated and is defined by a perpendicular line through the corpus callosum rostrum. A vertical line through the posterior aspect of the anterior commissure roughly differentiates the rostral from the caudal SMA.
Anatomic Organization
The relationships between distinct functional subregions within the SMA and the topographic distribution of these subregions have been intensely investigated. Recent studies have suggested a somatotopic organization in which, from anterior to posterior, the SMA represents the head, trunk, upper extremity, and lower extremity.
Using various fMR imaging motor, sensory, and language tasks, Chung and colleagues demonstrated the tendency of the anterior and rostral aspects of the ipsilateral SMA to activate during word generation and working memory tasks. By contrast, the contralateral SMA tended to activate during motor and heat sensory tasks, particularly in its caudal and posterior portions.
Supplementary motor area proper
The SMA proper receives inputs from the motor, premotor, and sensory cortices and provides a wide array of outputs, including outputs to the primary motor cortex, basal ganglia, thalamus, subthalamic nucleus, brain stem, contralateral SMA, and cervical motor neurons (primarily contralaterally). Projections from the SMA join those from the premotor cortex and compose approximately one-third of the corticospinal tract. The SMA proper is involved in planning, coordination, laterality, and initiation of movement, particularly of complex hand motions and action sequences that involve both sides of the body.
The SMA is usually the first area to be activated in motor tasks performed in fMR imaging studies. Bilateral finger-tapping primarily results in precentral and SMA activations, whereas toe tapping primarily activates the medial aspects of the motor gyrus ( Fig. 10 ). ,
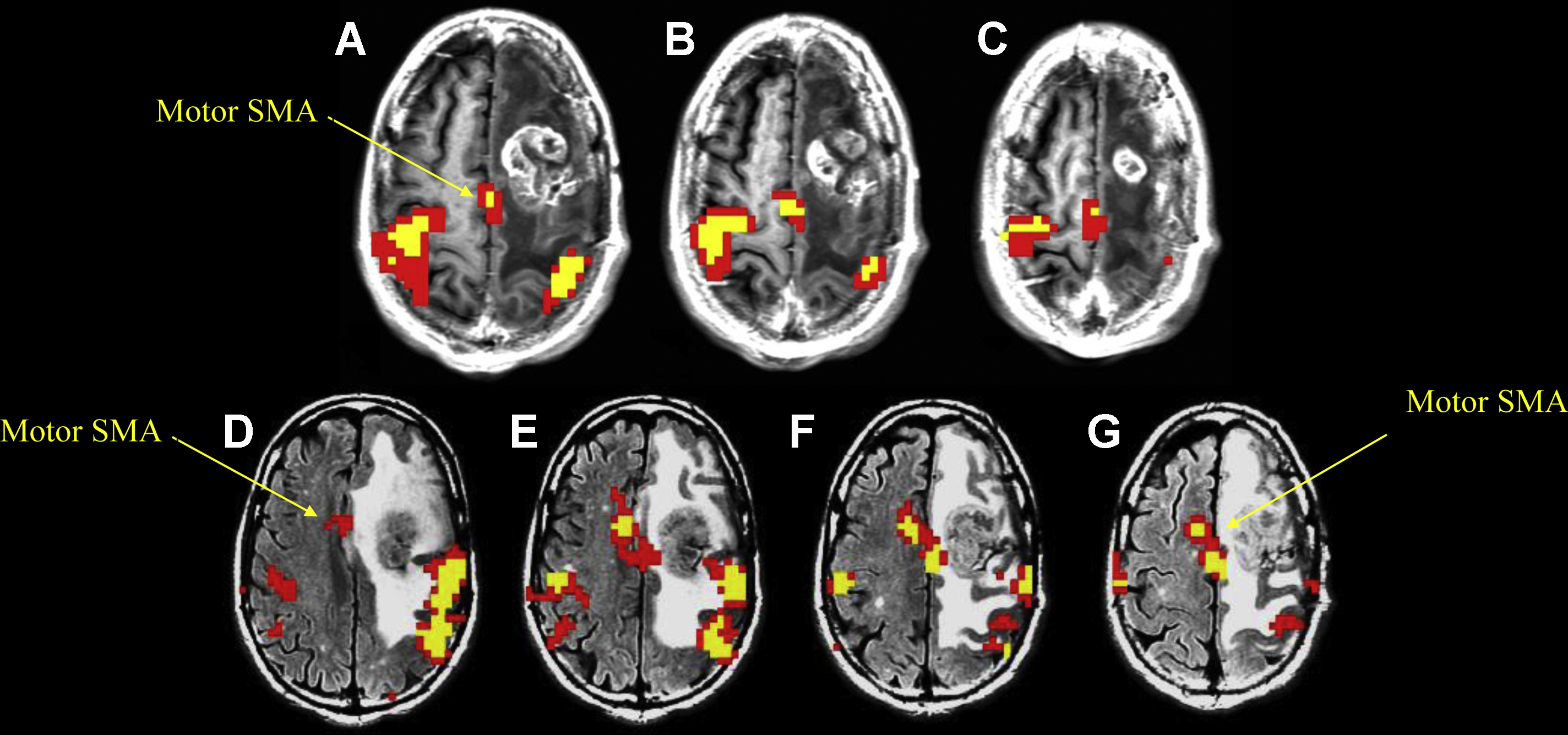
Unilateral resection of the SMA may result in “SMA syndrome,” which is characterized by contralateral global akinesia with normoflexia or hyporeflexia and a normal tonus with preservation of extremity muscle strength. Mutism is also associated with SMA syndrome. A notable feature of SMA syndrome is that the symptoms are temporary and usually completely resolve within weeks or months, leaving only a disturbance in alternating bimanual movements.
Lesions of the dominant SMA such as tumors or anterior cerebral artery infarctions result in abulia, gait apraxia, and transient weakness. , SMA lesions ( Fig. 11 ) can also result in “alien limb” syndrome, in which spontaneous movements of the limb, such as grasping, are uncontrollable by the patient, often countering the patient’s attempted goal-directed motion.
