Fig. 9.1
A schematic diagram of a gamma camera. PHA pulse-height analyzer; PM photomultiplier
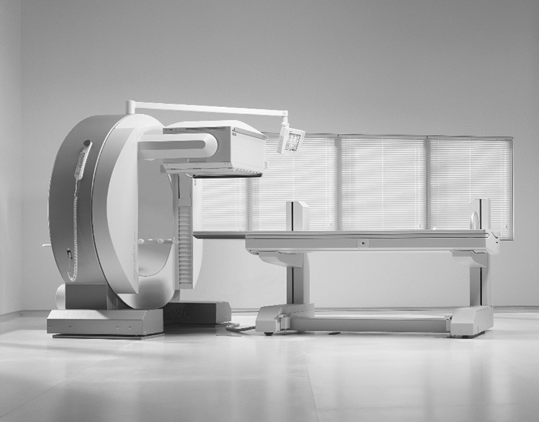
Fig. 9.2
A typical single-head gamma camera. (Courtesy of Siemens Medical Solutions USA, Inc.)
The operational principles of a gamma camera are identical to those of solid scintillation counters described in Chap. 8. Basically, γ-rays from a source interact with the NaI(Tl) detector, and light photons are emitted. The latter strike the photocathode of PM tubes, and a pulse is generated, which is then amplified by an amplifier and sorted out by a PHA. Finally, the pulse is positioned by an X-, Y-positioning circuit on the recording device or stored in the computer, corresponding to the location of γ-ray interaction in the detector (see later).
The functions of PM tubes, preamplifier, amplifier , PHA, and recording devices are the same as described in Chap. 8, and therefore only essential features pertaining to the use of gamma cameras will be highlighted.
Detector
NaI(Tl) crystals are the common detectors used in gamma cameras . In older cameras, circular detectors of a dimension of 25–35 cm in diameter were used, whereas in modern cameras, most manufacturers use rectangular detectors of the size of approximately 45 × 60 cm, which provide a practical field of view of 40 × 55 cm. Although the thickness of the detector used varies from 0.63 to 1.84 cm, the optimum thickness of 0.95 cm is used by many manufacturers in the current cameras. Thinner crystals (0.64 cm) are sufficient for low energy radionuclides such as 201Tl , 99mTc and 123I and used in portable gamma cameras for cardiac studies.
NaI(Tl) crystals are hygroscopic, and absorbed water causes color changes that reduce light transmission to the PM tubes. For this reason, these crystals are hermetically sealed in aluminum containers. Also, the entrance and side of the crystals are coated with a reflective substance (e.g. magnesium oxide) so that light photons are reflected towards the photocathode of the photomultiplier tube.
Increasing the thickness of a detector increases the probability of complete absorption of γ-rays and hence the sensitivity (defined in Chap. 10) of the camera. However, the probability of multiple Compton scattering also increases in thicker detectors, and therefore the X, Y coordinates of the point of γ-ray interaction can be misplaced. This results in poor resolution of the image of the area of interest. For this reason, thin NaI(Tl) detectors are used in gamma cameras, but this decreases the sensitivity of the camera, because many γ-rays may escape from the detector without interaction.
Collimator
In gamma cameras, a collimator is attached to the face of the NaI(Tl) detector to limit the field of view so that γ-radiations from outside the field of view are prevented from reaching the detector. Collimators are normally made of material with high atomic number and stopping power, such as tungsten and lead, of which lead is the material of economic choice in nuclear medicine. Two methods are employed to make collimators—the cast method and foil method. In the cast method, holes are made through a single slab of lead, whereas in the foil method, corrugated lead strips are glued together, through which holes are made. They are designed in different sizes and shapes and contain one or many holes to view the area of interest.
Collimators are primarily classified by the type of focusing, although other classifications are also made based on septal thickness and the number of holes . Depending on the type of focusing, collimators are classified as parallel-hole, pinhole, converging , and diverging types ; these are illustrated in Fig. 9.3. Pinhole collimators are made in conical shape with a single hole and are used in imaging small organs such as the thyroid glands to provide magnified images. Converging collimators are made with tapered holes converging to an outside point and are employed to provide magnified images when the organ of interest is smaller than the size of the detector. Images are magnified by converging collimators . Diverging collimators are constructed with tapered holes that are divergent outward from the detector face and are used in imaging organs such as lungs that are larger than the size of the detector. The images are minified with these collimators. Parallel-hole collimators are made with holes that are parallel to each other and perpendicular to the detector face and have between 4000 and 46,000 holes depending on the collimator design. These collimators are most commonly used in nuclear medicine procedures and furnish a one-to-one projected image. Because pinhole and converging collimators magnify and the diverging collimators minify the image of the object, some distortion occurs in images obtained with these collimators. Because large field of view (LFOV) cameras are readily available now, diverging collimators are not used in routine nuclear medicine studies.
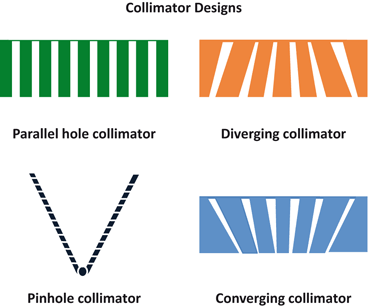
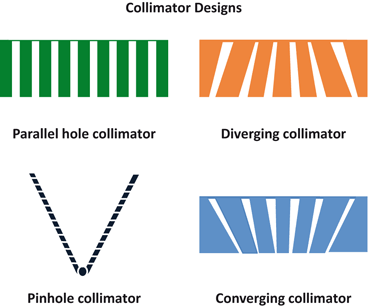
Fig. 9.3
Different designs of collimators
Parallel-hole collimators are classified as high-resolution , all-purpose, and high-sensitivity type, or low-energy, medium-energy, or high-energy type, depending on the resolution and sensitivity they provide in imaging. High-sensitivity collimators are made with smaller thickness than all-purpose collimators, whereas high-resolution collimators are thickest of all. These characteristics are discussed in detail in Chap. 10.
Several collimators are available that are designed for some specific purposes. Fan-beam collimators are designed with holes that converge in one dimension but are parallel to each other in the other dimension. These collimators are primarily used for imaging smaller objects and hence magnify the images. Cone-beam collimators are similar to fan-beam collimators and magnify the images except that the holes are designed such that they converge in two dimensions.
In earlier collimators, the holes were made circular, but current designs have square, hexagonal, or even triangular holes with uniform thickness of lead around the opening. These collimators provide better spatial resolution than the circular-hole ones.
Photomultiplier Tube
As in scintillation counters , PM tubes are essential in gamma cameras for converting the light photons in the NaI(Tl) detector to a pulse. Instead of one PM tube, an array of PM tubes are mounted on the back of the detector with optical grease, or in some instances, using lucite light pipes between the detector and the PM tubes. In older cameras, the number of PM tubes used were 19 or 37, whereas most manufacturers utilize 59 PM tubes in modern cameras. In modern gamma cameras, square or hexagonal PM tubes are used for better packing. The output of each PM tube is used to define the X, Y coordinates of the point of interaction of the γ-ray in the detector by the use of an X-, Y-positioning circuit (see later) and also is summed up by a summing circuit to form a pulse known as the Z pulse. The Z pulse is then subjected to pulse-height analysis and is accepted if it falls within the range of selected energies.
X-, Y-Positioning Circuit
Each pulse arising out of the γ-ray interaction in the NaI(Tl) detector is projected at an X, Y location on the image corresponding to the X, Y location of the point of interaction of the γ-ray . This is accomplished by an X-, Y-positioning circuit in conjunction with the PM tubes and a summing circuit. Fig. 9.4 illustrates the principles of X, Y positioning of pulses arising from γ-ray interactions in the detector employing seven PM tubes. All PM tubes are connected through capacitors to four output leads representing four directional signals, X +, X −, Y +, and Y −. The capacitance values are assigned in direct proportion to the location of the PM tube relative to the four signals. Suppose a γ-ray interacts at a location (*) near tube 7. The largest amount of light is received by tube 7, and other tubes receive light in proportion to their distances from the point of interaction. The output signals of PM tubes are weighted by the appropriate capacitance values and then summed to form each of the X +, X −, Y +, and Y − signals individually. In this case, X − will be greater than X +, and Y + will be greater than Y −, because the interaction occurred in the upper left quadrant. The X-, Y-designating pulses, X and Y, and the Z pulse are then obtained as follows: