Fig. 1
Publications describing rhenium-188 radiopharmaceutical development and clinical trials (data from MedLine)
From a clinical perspective, radionuclide therapy has a high impact in the treatment of benign diseases, such as thyroid disease and chronic joint inflammation. Radionuclide therapy is also important in the treatment of different oncological diseases, for instance in the management of thyroid cancer, metastatic neuroendocrine tumors, pain induced by bone metastases, and primary liver cancer or liver metastases. A wide range of applications has been reported using commercially available radionuclides, such as 89Sr (Pecher 1942; Robinson et al. 1989), 32P (Friedell and Storaasli 1950), 131I (Meier et al. 2002; Sherman 2003), 186Re (Dafermo et al. 2001), 153Sm (Enrique et al. 2002), and 90Y (Schneider et al. 2005; Kennedy et al. 2006). However, radioprotective concerns, limited availability (especially in developing countries), and high cost have prevented widespread use of many radionuclide treatments.*****
Similar to the in-house use of the 99Mo/99mTc generator in radiopharmacy for radiolabeling a wide variety of diagnostic agents, generator-derived therapeutic radioisotopes would contribute to the further development and implementation of radionuclide therapies (Lambert and de Klerk 2006; Knapp et al. 2004). The introduction of the alumina-based chromatographic-type 188W/188Re generator at some centers has increased the therapeutic options for oncological patients and has reduced the cost of treatment, especially because of its long shelf-life (several months) (Knapp et al. 1994, 1997a, 1998, 1998a; Callahan et al. 1989; Kamioki et al. 1994). The physical characteristics of 188Re favor routine use of this radionuclide for treatment. The high-energy beta emission (maximum energy 2.12 MeV) leads to deep penetration of radiation in target tissues, resulting in high-radiation “cross-fire” and a more homogeneous tumor dose distribution, especially for bone pain palliation and for treatment of liver metastases or primary liver cancer (Liepe et al. 2009). The 155 keV gamma emission, with an abundance of 15 % and relatively short half-life of 16.9 h, limit radioprotection problems and represent a distinct advantage over the current widespread use of 131I for various therapeutic radiopharmaceuticals (Lambert and de Klerk 2006). Although a variety of other 188W/188Re generator prototypes are being evaluated, including electrochemical separation (Chakravarty et al. 2009) and the use of the W/Zr “gel” system (Ehrhardt et al. 1992), 188Re from the alumina-based system has been exclusively used for the clinical trials described in this chapter.
Radiolabeling of various agents with 188Re provides a variety of different therapeutic options, including bisphosphonates for the treatment of bone pain palliation, such as hydroxyethylidene diphosphonate (HEDP) (Palmedo et al. 2000), 4-amino-1-hydroxy-1,1-butylidene-bisphosphonate (ABP) (Arteaga de Murphy et al. 2001), or dimercaptosuccinic acid complex (DMSA) (Blower et al. 1998). Both lipiodol (Lambert et al. 2009; Lambert and Van De Wiele 2009; Lambert et al. 2006a; Lambert and de Klerk 2006b; Lambert 2005a; Lambert et al. 2005b, 2003) and microspheres (Liepe et al. 2005) in patients with liver metastases or primary liver cancer and with colloids for radiosynovectomy (Liepe et al. 2007a). The 188Re-labeled α-melanocyte stimulating hormone peptide analogues have also been evaluated in animal models for melanoma therapy (Miao et al. 2002a, b, 2003, 2005). Rhenium-188-labeled antibodies have been introduced for the treatment of patients with leukemia (Okuda et al. 1985; Bunjes et al. 2001), and a variety of 188Re-labeled agents have been successfully applied in intracoronary brachytherapy, including with 188Re perrhenate obtained directly by saline elution of the generator (Woehrle et al. 2007; Reynen et al. 2004) or the 188Re-labeled renal scintigraphic diethylenetriaminopentaacetate (DTPA) pharmaceutical kit (Cho et al. 2004) and 188Re-labeled mercapto-acetyltriglycyl (MAG3) (Lin et al. 2000a). In experimental studies, 188Re has also been attached by direct labeling with melanin-binding IgM antibody to treat human melanoma cells (Dadachova et al. 2008, Schweitzer et al. 2007), with hyaluronic acid for primary liver cancer (Melendez-Alafort et al. 2009), the anti-CD52 monoclonal antibody alemtuzumab for radioimmunotherapy of B cell chronic lymphocytic leukemia (De Becker et al. 2008), the P2045 somatostatin peptide analog in advanced non-small cell lung cancer (Edelman et al. 2009), and an IgG anti-carcinoembryonic antigen monoclonal antibody (Mab) in patients in advanced gastrointestinal cancer (Juweid et al. 1998). The 188Re-C595 antibody has been evaluated for treatment of bladder cancer (Murray et al. 2001). Other examples include a 7-amino acid analog of bombesin for therapy of small-cell lung cancer (Safavy et al. 1997) and a 188Re-liquid-filled balloon catheter strategy for inhibition of hyperplasia in benign airway (Kim et al. 2008) or esophageal strictures (Shin et al. 2008). In addition, studies using 188Re-perrhenate to probe the iodide symporter have been reported (Dadachova et al. 2002. Table 1 summarizes a variety of other 188Re-labeled agents which have been developed but have apparently not yet entered the clinical trials.
Table 1
Examples of development and pre-clinical studies with rhenium-188-labeled radiopharmaceuticals
188Re-labeled agent | Reference | Potential Application |
---|---|---|
Glass microspheres | For radioembolization | |
MAG3 | Guhlke et al. 1998 | For peptide radiolabeling |
Magnetic particles | Hafeli et al. 2001a | Cancer targeting |
Microspheres | Hafeli et al. 2001b | Cancer targeting |
Cisplatin-hydrogel | Kim et al. 2008 | Locoregional delivery for breast and prostate cancer therapy |
Although these and many other studies which have probed the use of 188Re-labeled radiopharmaceutical agents are described in the literature, the goals of this chapter are to briefly describe the practical details for routine use of the 188W/188Re generator system in a radiopharmacy/clinical setting, and to review the results and status of several recent and current successful therapeutic clinical applications of 188Re in nuclear medicine, oncology, and interventional cardiology/radiology. Particular applications that are discussed include the use of 188Re agents for bone pain palliation, synovectomy, arterial restenosis therapy, and treatment of non-melanoma skin cancer. The successful results of these studies are expected to provide the basis for further development and routine introduction of this important generator system for cost-effective routine clinical use. The fact that most 188Re-labeled radiopharmaceuticals are administered as unsealed radioactive sources, use of these agents represents an important opportunity for interaction between nuclear medicine physicians and colleagues in oncology, interventional cardiology/radiology, and other clinical specialties.
3 Tungsten-188/Rhenium-188 Generator
3.1 Overview
The 188W/188Re generator provides a convenient in-house radioisotope production system in which 188Re can be conveniently obtained on demand by elution with 0.9 % saline solution. Tungsten-188 is reactor produced, and following processing (Knapp et al. 1997a, 1998a), the generator is fabricated by the adsorption of tungstic acid on acid-washed alumina (Fig. 2).
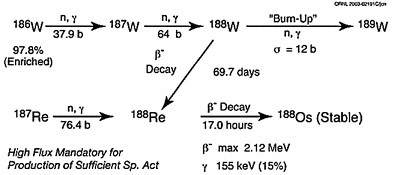
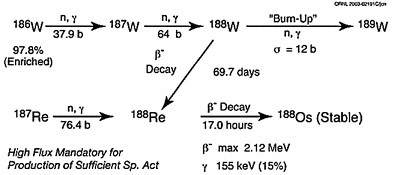
Fig. 2
Production and processing of tungsten-188 and availability of no-carrier-added rhenium-188 from the tungsten-188/rhenium-188 generator system. (Figure from Authors–RK)
The convenient use of the generator system to obtain daily elutions of 188Re is illustrated in Fig. 3. Following removal of the available 188Re, about 62 % of the equilibrium levels of 188Re is available after only 24 h, illustrating the great benefits of daily elution to obtain high activity levels of the therapeutic 188Re radioisotope. For example, a generator containing 1 Ci of 188W would initially provide about 600 mCi of 188Re by daily elution. Four repetitive elution-in-growth cycles are illustrated in Fig. 4, and estimated 188Re activity levels that would be available as the 188W parent (69 day half-life) decays over a 3 month period are illustrated in Fig. 5.
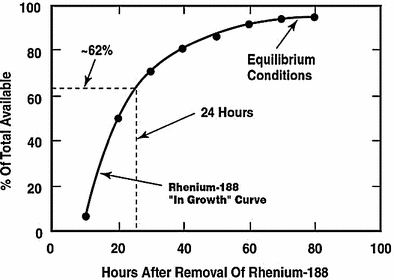
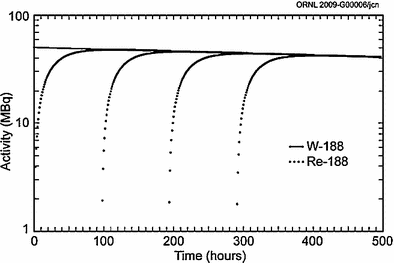
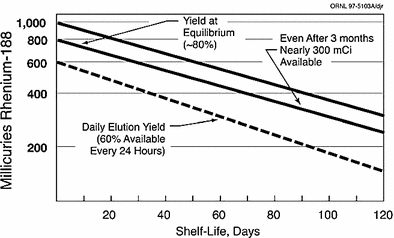
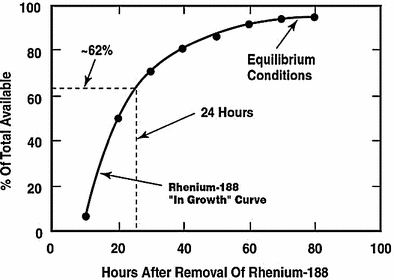
Fig. 3
Illustration of the opportunity for daily elution of the tungsten-188/rhenium-188 generator system (Figure from Authors–RK)
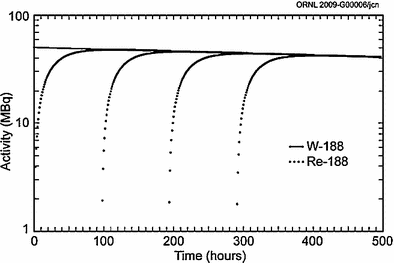
Fig. 4
Example of four in-growth/elution cycles for the tungsten-188/rhenium-188 generator system (Figure from Authors–RK)
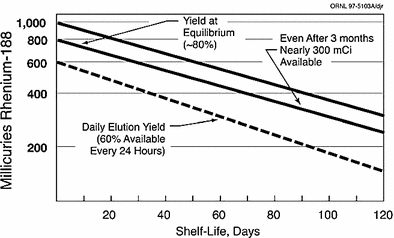
Fig. 5
Estimated activity levels of rhenium-188 available over a 3-month period from elution at equilibrium (Figure from Authors–RK)
The 188W/188Re generator is similar to other adsorption-type generator systems, such as the 99Mo/99mTc, 82Sr/82Rb, and 68Ge/68Ga generators, used in nuclear medicine in which the daughter is conveniently removed in the radiopharmacy by simple elution with the appropriate solution (Roesch and Knapp 2003). The generator is housed in a lead shield that is shipped from the manufacturer to the clinical site. Entry elution lines allow connection to the eluant supply (usually physiological saline), and the exit line allows collection of the carrier-free 188Re saline solution in the appropriate system. The generator provides consistently high 188Re yields with low 188W parent breakthrough, as illustrated in Fig. 6. Because of the long 69 day 188W physical half-life, the generator essentially has an unlimited shelf-life and is only limited by the 188Re activity levels required for a particular application, and any regulatory requirements.
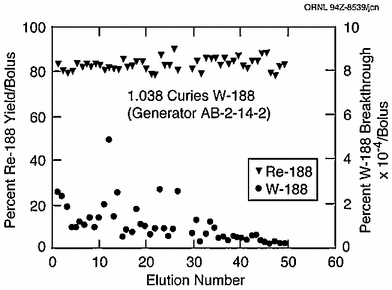
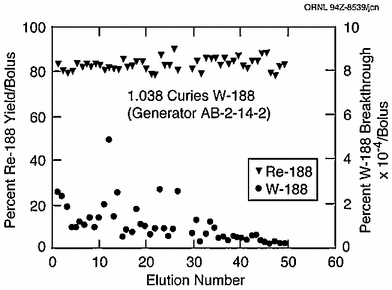
Fig. 6
The repeated high rhenium-188 activity yields and concomitant low tungsten-188 breakthrough values per bolus obtained over a 2-month period illustrated for a typical alumina-based generator from ORNL loaded with 1 Ci of tungsten-188 (Figure from Authors–RK)
In comparison to the operation of the well-established 99mTc/99Mo generator system, saline elution of 188Re from the adsorptive-type 188Re/188W generator system provides a much more dilute eluant solution. For this reason, post-elution 188Re concentration strategies can optimize use of the generator and can extend the shelf-life considerably. Such efficient and reproducible methods have been developed, widely used in clinical trials, and can be readily installed and used in the radiopharmacy setting. Although details of radiochemistry/radiopharmacy use of the generator are beyond the scope of this discussion, they have been discussed in the literature (Knapp et al. 1997a; 1998; 1999; Blower et al. 2000). Currently, the 188W/188Re generators are available from manufacturers at the Oak Ridge National Laboratory and Polatom (Table 2). Recently, unit doses of 188Re have become available from ITG-Munich, Germany, and IRE-Fleurus, Belgium, where plans are evidently being finalized for routine cGMP generator production.
Table 2
Availability of tungsten-188/rhenium-188 generators–January 2010
Organization | Activity level | Comments |
---|---|---|
ORNL, USA | Up to 3 Ci | Over 500 generators provided worldwide since 1986. DMF registered with FDA and cGMP quality program implemented for radiochemical product www.ornl.gov/isotopes/catalog.html |
Polatom, Poland | Limited to <500 mCi | Non cGMP |
ITM Munich, Germany | Currently providing up to >1 Ci Re-188 | Generator installed in central processing center; Non cGMP |
IRE, Fleurus, Belgium | N/A | Manufacturing facility under construction |
Maintaining good quality control with long-term, continued use of the generator system may be most effectively addressed in a centralized radiopharmacy setting where routine distribution of unit doses for onsite radiolabeling or preparation of 188Re-labeled agents and subsequent hospital delivery are available.
3.2 Rhenium-188 Radiolabeling Chemistry
The similarity between the chemical strategies developed for introducing 188Re into radiopharmaceuticals (Blower et al. 2000) is often subtle when compared to 99mTc chemistry, but can also be quite different. Nonetheless, radiochemical methods used to introduce 99mTc into diagnostic agents can generally be used to as a framework in the preparation of analogs agents radiolabeled with 188Re for therapeutic applications.
3.3 Radiation Protection
Radiopharmacy use of high-activity 188W/188Re generators (>1 Ci) for the preparation of 188Re-labeled unsealed and sealed radiopharmaceuticals represents a unique challenge, since there are no other examples in which such high activity levels of a therapeutic radioisotope are routinely obtained from an in-house generator. Generator use requires careful procedural and ALARA controls to minimize any radiation exposure to radiopharmacy and clinical staff. As shown in Table 3, generator elution and 188Re bolus concentration steps are taken, which can be readily and effectively semi-automated or fully automated (Lee et al. 2006; Luo et al. 2006; Jaeckel et al. 2005; Oh et al. 2001, 2003; Wunderlich et al. 2008). If care is not taken when handling 188Re, extremity radiation dose readings to the fingers can be quite high. Some groups have carefully measured exposure levels during routine clinical use of 188Re. One example involves the use of 188Re-liquid-filled balloons for the inhibition of hyperplasia following coronary angioplasty. In one study (Andreef et al. 2005) investigators found that the extremity exposure could be reduced to acceptable levels by implementing well-choreographed procedures. In a more recent study, investigators were able to repeat and verify similar measurements, and found maximum skin dose values for the interventionist and attending physicist of 76 and 50 mSv per treatment, respectively, which were dramatically reduced to values of about 2 mSv in the angioplasty suite using the new tungsten-shielded “FlowMedical Application System” (Barth et al. 2009). Because of the relatively short 16.9 h half-life of 188Re, outpatient treatment regimens—or at least reduced hospitalization—would be expected in some applications, as compared with longer lived therapeutic radioisotopes such as 90Y and 131I, based on local regulations.
Table 3
Automated and semi-automated systems developed for elution and concentration of rhenium-188 obtained from the tungsten-188/rhenium-188 generator system
Institution | Comment | Reference |
---|---|---|
Department of Nuclear Medicine, University Hospital, Dresden, Germany | System incorporated for routine radiopharmacy and clinical use concentrated 188Re perrhenate solutions | Wunderlich etal. 2008 |
Institute for Nuclear energy Research, Taoyuan, Taiwan | Concentration of 188Re perrhenate solutions for radiolabeling | Lee et al. 2006 |
Paul Scherrer Institute, Wuerinlingen, Switzerland | Unit used for elution and concentration of high 188Re perrhenate activity levels for R&D | Jaeckel et al. |
Department of Nuclear Medicine, Ferrara, Italy | Comecer unit used in clinical trials for perrhenate concentration and 188Re -DEDC synthesis | Duatii et al. |
Department of Radiology, Asan Medical Center, Seoul, Korea | System for elution and concentration, and 188Re-MAG3 synthesis for intracoronary irradiation | Oh et al. 2001 |
3.4 Practical Issues for Gamma Camera Imaging with Rhenium-188
While 188Re decays with the emission of a gamma photon with an energy of 155 keV which permits ready imaging with traditional static and SPECT imaging methods, the emission of much less intense but high-energy photons (Fig. 7) requires the selection of optimal energy windows for imaging. In one study, the effects of energy window calibration were evaluated to assess image quality and dosimetry implications using static and SPECT imaging in a phantom study (Hambye et al. 2002). Because of the emission of the high-energy photons, a LEAP collimator cannot be used for imaging of the 155 keV gamma photon, and high-energy collimators with the appropriate window settings (Hambye et al. 2002) must be used to visualize 188Re distribution.
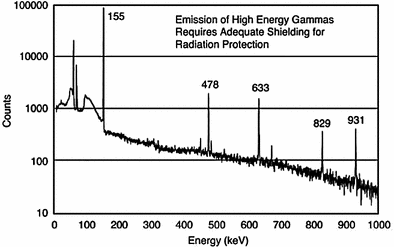
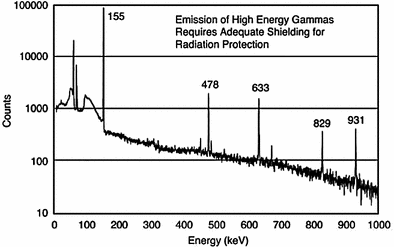
Fig. 7
Gamma spectrum of rhenium-188 obtained with a high purity Ge crystal (Figure from Authors–RK)
The opportunity for static or SPECT imaging of the 155 keV gamma photon emitted during the decay of 188Re also offers an increasingly important opportunity for patient-specific dosimetry, to assess the pharmacokinetics and excretion kinetics following administration of 188Re-labeled radiopharmaceutical agents (Hambye et al. 2002).
4 Therapy with Rhenium-188
4.1 Therapy of Metastatic Bone Pain
Skeletal metastases occur in many patients with different malignant tumors, especially in advanced stage of prostate, breast, and non-small cell lung cancer. Resulting bone pain interferes with quality of life and requires effective treatment. Unfortunately, various traditional non-radiotherapeutic modalities, such as analgesics, hormone therapy, orchidectomy, cytostatic and cytotoxic drugs, bisphosphonates, and surgery are not effective in all cases, especially in the late stage of the diseases (McEwan 1998; Debes and Tindall 2002; Gilligan and Kantoff 2002). External-beam radiotherapy is suitable only for well-defined localized bone metastases. Extended field radiation may be useful in patients with diffuse metastases but is often accompanied by serious side effects (Lewington 1993). Therefore, systemic radionuclide therapy must be considered as a valuable and effective method of treatment in patients with widespread skeletal metastases.
This treatment option is effective in delivering high absorbed doses to widespread metastatic lesions in bone while limiting the absorbed dose to normal tissue (Breen and Battista 2000). The first treatment with 89Sr in humans was described by Pecher (Pecher 1942) in 1942. (Friedell and Storaasli (1950) published the first results using 32P in the treatment of metastatic breast cancer. In subsequent reports of treatments with 32P-phosphate by other researchers, the higher bone marrow uptake was reported to deliver a higher bone marrow toxicity of this radionuclide (Silberstein 1993; Nair 1999; Fettich et al. 2003). This agent has primarily been used in developing countries (e.g., India) because of its lower cost as compared with the other commercially available radiopharmaceuticals. Many studies have reported the therapeutic efficacy of 89Sr in metastatic bone pain (Lewington et al. 1991; Robinson et al. 1995), but only one report each has described the use of 90Y (Kutzner et al. 1982) and 131I-alpha-amino-(4-hydroxybenzylidene)-disphosphonate (BDP3) (Eisenhut et al. 1986) in bone pain palliation. More recently, several other radiopharmaceuticals using radioisotopes with shorter physical half-lives have been developed, such as 153Sm-ethylenediaminetetramethylene phosphoric acid (EDTMP) (Turner et al. 1989) and 186Re-HEDP (Maxon et al. 1988).
The most extensive study using 89Sr was described by Robinson et al. (1995) in which 622 patients were treated and an overall response rate of 81 % was reported, with a 15 % rate of complete response. A double-blind, crossover, placebo-controlled trial using 186Re-HEDP reported a response rate in 80 % of patients with a mean duration of response of 7 weeks (Maxon et al. 1991). The only major toxicity was a mild reversible thrombocytopenia. In a dose escalation study by de Klerk et al. (1994), a fixed applied activity of 1,295 MBq (35 mCi) 186Re-HEDP was compared with escalating activity that was increased incrementally by 555 MBq (15 mCi) to a maximum of 3,515 MBq (95 mCi). In two large studies using 153Sm-EDTMP with more than 550 patients, a response rate of 73 and 86 % was reported (Tian et al. 1999; Enrique et al. 2002). Enrique et al. documented pain relief in 50 % of patients with a duration of response between 4 and 8 weeks, and 50 % in more than 8 weeks (Enrique et al. 2002). In 227 patients with an administered activity between 18.5 and 55 MBq/kg (0.5 and 1.5 mCi/kg), 19.7 showed a thrombocytopenia grade I or II and 6.9 % a grade III or IV. More recently, preliminary patient studies with 117mSn-DTPA (Atkins et al. 1995) and 188Re-DMSA (Blower et al. 2000) have been reported to be effective for bone pain palliation, but further clinical use of these agents has not been reported. In contrast, use of 188Re-HEDP has been more widely reported in several clinical trials and has several advantages which indicate this agent may hold promise for broader clinical use (Palmedo et al. 2000; Liepe et al. 2003a, b; Savio et al. 2004).
Rhenium-188 is of special interest in clinical applications because of its excellent availability and cost-effectiveness as a generator product (Liepe and kotzerke 2005). Disphosphonates such as methylene disphosphonate (MDP) and hydroxymethane disphosphonate (HDP) are well-known bone-seeking agents for imaging with 99mTc. However, for unknown reasons 188Re-labeled HDP and MDP do not show sufficient uptake in the skeleton, with high soft-tissue uptake (Hsieh et al. 1999). In contrast, HEDP showed significant higher skeletal uptake and similar results in radiolabeling procedures as 186Re-HEDP (Maxon et al. 1998; Lin et al. 1999; Li et al. 2001). The first clinical data, reported by Palmedo et al. (2000), showed the results of a dose escalation study using 1.3 GBq (35 mCi), 2.6 GBq (70 mCi), 3.3 GBq (90 mCi), and 4.4 GBq (120 mCi) of 188Re-HEDP in a small group of prostate cancer patients (22 men). The first hematotoxic results were noted in those patients with an administered activity of 2.6 GBq 188Re-HEDP. In the 3.3 GBq group, one patient exhibited reversible Grade 1 and 2 thrombocytopenia. In the 4.4 GBq group, thrombocytopenia of Grades 3 and 4 was observed in one and two patients [baseline platelet counts <200 × 109/l], respectively. With respect to bone marrow toxicity, the authors postulated an activity of 3.3 GBq as the standard activity of this agent, with the exception of patients with a baseline platelet count level above 200 × 109/l, which might also be tolerable at a higher activity of 4.4 GBq. Pain palliation was reported by 64 % of patients, with a mean duration of 7.5 weeks. The response rate seemed to increase with higher doses, reaching 75 % in the 4.4 GBq group.
Another group from Dresden (Liepe et al. 2003b) focused on the impact of dosage on the general status of the patient. In 27 patients with prostate cancer who were given 3.3 GBq (90 mCi) of 188Re-HEDP, an increase in Karnofsky performance scale from 74 ± 7 % before therapy to 85 ± 9 % at 12 weeks after therapy was obtained (p = 0.001) (Fig. 8). In addition, pain relief (≤25 % on the VAS for at least 2 consecutive weeks without an increase in analgesics intake) was achieved in 76 % of patients and 20 % of patients were pain free. The pain score showed a maximum decrease from 4.4 ± 1.8 to 2.7 ± 2.0 in the third to 8th weeks after therapy (p = 0.009) (Fig. 9). In one case, evidence of a curative effect of the therapy was demonstrated, with a significant decrease of the bone turnover in the bone metastases, as indicated by a 99mTc-HMDP bone scan (Fig. 10).
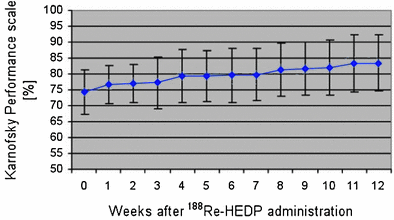
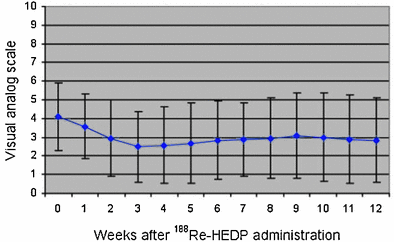
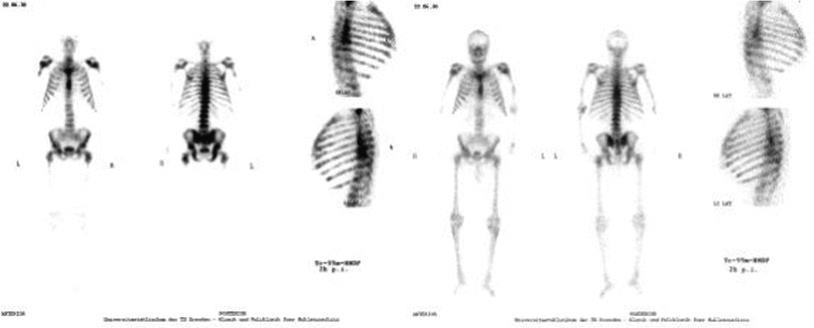
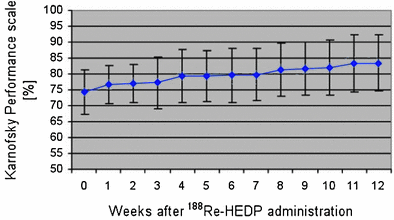
Fig. 8
Time course of Karnofsky performance scale as a sign for the general functional status of the patients after 188Re-HEDP administration (Figure from Authors–KL)
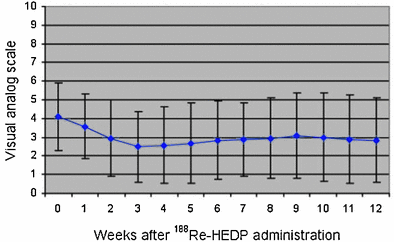
Fig. 9
Time course of the pain visualized by a VAS in 10 steps after 188Re-HEDP administration (Figure from Authors–KL)
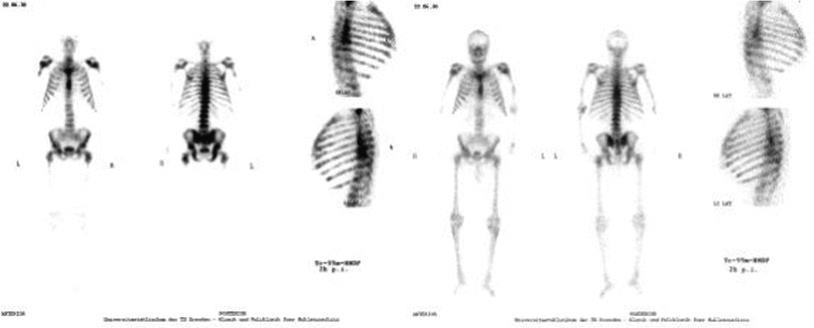
Fig. 10
Prostate cancer patient with bone metastases and a Karnofsky performance scale of 75 % before therapy. Left Panels—“Superscan” (extensive disseminated bone metastases in the central body) in the 99mTc-HMDP bone scan before the therapy; Right Panels—the same patient after a therapy with 3.1 GBq of 188Re-HEDP, the 99mTc-HMDP bone scan showed a significant reduce of the bone metabolism in all site of the tumor (Liepe, Kropp et al. 2003) (Figure from Authors–KL)
Other groups have also described a therapeutic effect from 188Re-HEDP therapy in other malignancies, such as lung, renal, rhinopharyngeal, and bladder cancer with 70–80 % pain relief (Fig. 11 ) (Li et al. 2001; Murray et al. 2001).
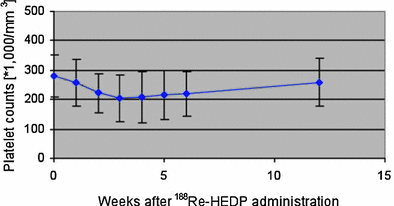
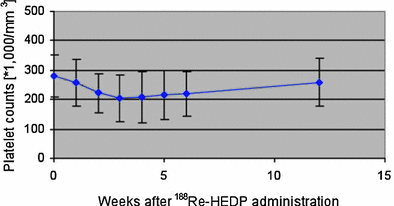
Fig. 12
Time course of platelet counts after 188Re-HEDP administration (Figure from Authors–KL)
Bone marrow toxicity, especially thrombocytopenia, traditionally represents the significant side effect in systemic radionuclide therapy. Liepe et al. (2003b) reported a moderate transient bone marrow toxicity with a decrease in platelet counts from a baseline value of 286 ± 75 × 109/l to a maximum of 218 ± 83 × 109/l) with the nadir at 3 weeks (Fig. 12). The authors found no evidence of either local or systemic intolerance to treatment with 188Re-HEDP, while a flare reaction with an increase in pain within 14 days after therapy was noted in 16 % of patients.
One clinical trial using 188Re-HEDP, 186Re-HEDP, 153Sm-EDTMP, and 89Sr in 79 patients with breast and prostate cancer (Liepe and Kotzerke 2007) found no significant differences in thrombocytopenia and leukopenia (p = 0.059–0.470). Anemia plays a minor role in the toxicity of bone pain palliation. After 188Re-HEDP administration, patients showed a 30 % decrease in the platelet counts from the baseline level to the nadir. Typically the nadir is early for thrombocytopenia and leukopenia using radionuclides with short physical half-lives (nadir between the second and 4th weeks after therapy) in contrast to the radionuclides with a longer physical half life, such as 89Sr (nadir between the 4 and the 6th weeks after therapy).
New important therapeutic advances for bone pain palliation include the option of repeated radiotracer administration rather than a single administration (Palmedo et al. 2003). New options also include a combination of radionuclide therapy with chemotherapy (Lam et al. 2009) or bisphosphonates (2009), and radionuclide therapy in primary bone tumors such as sarcoma (Anderson 2006). The group from Bonn (Palmedo et al. 2003) compared a standard single dose with two administrations of 3.3 GBq (90 mCi) of 188Re-HEDP in 64 patients with prostate cancer. An important finding was the significant extension of the median times to progression and time of survival, from 2.3 to 7.0 months for a single administration to 7.0 and 12.7 months for two administrations, with an interval of 8 weeks, respectively (p < 0.01). There was also a 60 % increase in pain relief following a single administration and a 91 % increase after two administrations (p < 0.01). Moreover, a significant reduction in the levels of the prostate-specific antigen (PSA) was documented following repeated administration, whereas this effect was not observed after just a single injection (Palmedo et al. 2003). In another study (Lam et al. 2009), 12 patients with hormone refractory prostate cancer were treated with 37 MBq/kg (1 mCi/kg) 188Re-HEDP in combination with two applications of capecitabine (Xeloda®). A dose of 2,500 mg/m2 Capecitabine per day in combination with 188Re-HEDP was reported as safe, but data on the pain relief on a Karnofsky performance scale were not documented. Clinical data using 188Re-HEDP in primary bone tumors or a combination with bisphosphonates in patients with bone metastases were not published. Zhang and co-workers have reported the successful palliative use of 188Re-HEDP in patients with metastases from lung cancer (Zhang et al. 2003)
The kinetics of 188Re-HEDP is favorable for bone pain palliation. Approximately 51 % of the injected dose is absorbed by the skeleton 3 h after administration. The decay-corrected whole body retention as the percentage of the administered activity decreases rapidly, in contrast to a slow decrease from the bone metastases. The biological half-life values (T½biol) also differ significantly between the whole body (T½biol = 51 ± 43 h) and the bone metastases (T½biol = 269 ± 166 h). These values correspond with rapid urinary excretion of 188Re-HEDP; 8 and 48 h after administration, 40 (%ID) and 60ID % of the dose, respectively, were excreted (Liepe et al. 2003). Savio et al. (2001) reported a urinary clearance of 70 within the first 6 h, bone uptake values of 10–70, and a remaining blood dose of 9 % at 2 h. The bone uptake 24 h post-administration was 43 %. The dosimetric data for 188Re-HEDP are comparable with the data from the commercially available radiopharmaceuticals used for bone pain palliation, with values of 3.83 ± 2.01 mGy/MBq for bone metastases, 0.61 ± 0.21 mGy/MBq for the bone marrow, 0.07 ± 0.02 mGy/MBq for the whole body, 0.71 ± 0.22 mGy/MBq for the kidneys, and 0.99 ± 0.18 mGy/MBq for the bladder, respectively (Table 4) (Liepe et al. 2003b).
Table 4
Mean radiation absorbed dose values using 3.3 GBq of 188Re-HEDP
Values | Target | ||||
---|---|---|---|---|---|
Bone metastases | Red bone marrow | Whole body | Kidneys | Bladder | |
Mean radiation dose in gray Ratio of metastases to organ | 12.6 N/A | 2.0 6.3 | 0.2 63 | 2.3 5.3 | 3.2 3.9 |
There is a limitation on the availability of estimated radiation absorbed dose data concerning the relative radiotracer uptake of the bone-seeking radiopharmaceutical in the trabecular and cortical regions of normal skeleton and uptake in bone metastases. The commercially available human software dosimetry code MIRDOSE (Stabin 1996) assumes an equal (50 %ID) radiopharmaceutical uptake in trabecular and cortical skeletal regions based on standard assumptions of equal surface area used in International Commission on Radiological Protection radiation protection models (ICRP 1995). However, some studies have reported higher radiotracer uptake in trabecular in comparison to cortical bone (Swailem et al. 1998; van Rensburg et al. 1998). Since red marrow cells are only found in trabecular bone, the radiation absorbed dose values to active bone marrow are thus proportional to the uptake in trabecular bone. Standard tumor dosimetry models assume a homogeneous distribution of the radiopharmaceutical, although it is acknowledged that bone metastases are formed by tumor cells, stromal cells, osteoclasts, and osteoblasts. Uptake of the bone-seeking radiopharmaceutical is limited to bone cells within the skeletal metastasis; inhomogeneous distribution of activity is thus expected (Liepe et al. 2009).
Liepe et al. developed a new model of osteoblastic bone metastases in rats and sampled animal data of 188Re-HEDP in bone metastases and normal skeleton (Liepe et al. 2005, 2009). In contrast to the MIRDOSE model with an assumption of 50–50 % distribution of bone-seeking agents in trabecular and cortical bone, the authors documented a 65 uptake in trabecular and 35 % uptake in cortical part. They concluded from these animal data that conventional radiation absorbed dose calculations using MIRDOSE underestimate the bone marrow dose. The same challenge exists for more accurate estimation of radiation absorbed dose calculation for bone metastases. The established models postulate a homogeneous bone-seeking agent distribution (Samaratunga et al. 1995) or uptake to a depth of 1 μm from the bone surface (Graham et al. 1980) in bone metastases. However, in animal studies a significant inhomogeneous distribution has been found in the whole volume of the bone metastases (Liepe et al. 2009), and these data are relevant for the discussion of the most favorable radionuclides for bone pain palliation.
Some researchers prefer the use of radionuclides with low beta energy for treatment of painful bone metastases, with the assumption that such radionuclides would reduce bone marrow toxicity. However, little evidence for such a strategy is available (Lambert and de Klerk 2006). Rhenium-188 HEDP, 186Re-HEDP, 153Sm-EDTMP, and 89Sr show similar pain relief in patients suffering from breast or prostate cancer. Also, no differences are found concerning bone marrow toxicity between the high-energy beta emitters (188Re-HEDP and 89Sr) and the low-energy beta emitter 153Sm-EDTMP (Liepe and Kotzerke 2007). The high-energy beta emitter 188Re-HEDP has a range of 3–5 in osseous tissue, with a maximum range of 11 mm, which would be the same in the target tumor as well as surrounding bone. It is likely that bone-seeking agents represent only uptake in the normal bone cells in the bone metastases, but not in tumor cells. For example, in vitro cultures of MylLyLu cells incubated with 188Re-HEDP show no specific uptake in the tumor cells (unpublished data, oral communication). This assumption correlates with the data of selective accumulation of 89Sr in skeleton (Ben-Josef et al. 1995). The higher range beta emission of 188Re in the Re-HEDP agent should lead to a higher cross fire and a more homogeneous dose distribution in the bone metastases, and in the end to a higher radiation absorbed dose to the tumor cells.
There are only limited data available concerning the use of other bone-seeking agents radiolabeled with 188Re. Blower et al. (2000) documented data from treatment of 10 patients with 600 MBq (16 mCi) 188Re dimercaptosuccinic acid complex [(V)DMSA]. The authors reported a significantly lower post-therapeutic uptake of 188Re(V)DMSA compared to a previous scan using 99mTc(V)DMSA in skeleton, which differed by a factor of approximately 0.8. Unfortunately, no concomitant data were reported concerning the clinical benefit for the treated patients. Other publications reported animal kinetic data of new bisphosphonates, such as 2-sulfonato-1,1-ethylidene bisphosphonates acid (SEDP) (Lisic et al. 2001), a conjugate between 5-fluorouracil and bisphosphonates (5-FU/BP) (El-Mabhouh and Mercer 2005), and 4-amino-1-hydroxy-1,1-butylidene-bisphosphonate (ABP) labeled with 188Re (Arteaga de Murphy et al. 2001).
As an extension of this discussion concerning the benefit of pain palliation, tumor regression, and soft tissue penetration of radiation, the current opinion is that treatment of bone metastases using alpha emitters, such as 223Ra (“Alpharadin™”), leads to minimal bone marrow toxicity, but also to a limited dose to the tumor cells (Liepe 2010). A Phase II, randomized, placebo-controlled, double-blind, multicenter clinical trial assessed Alpharadin™ (50 kBq/kg iv) as an adjunct to external-beam radiotherapy in patients (n = 64) with bone metastases from HRPC. Patients were randomized to receive four injections of Alpharadin (n = 33) or saline (n = 31), administered at 4 week intervals. Time to disease progression was significantly increased in the treated group compared with the placebo group when assessed by analysis of bone alkaline phosphatase (BAP) (39 versus 12 weeks; p = 0.045) and PSA (26 versus 8 weeks; p = 0.048) levels (Nilsson et al. 2005; Nilsson et al. 2007). BAP (−65.6 versus +9.3 %; p < 0.0001). The PSA (−23.8 versus +44.9 %; p = 0.003) levels were also significantly reduced from baseline in the treated group compared with the placebo group (Nilsson et al. 2007). In addition, the median survival was significantly increased in the treated group compared with placebo (65.3 versus 46.4 weeks; p = 0.066).
Other interesting therapeutic approaches using radionuclides which have been evaluated include the combination of systemic radionuclide therapy with bisphosphonates and local field external-beam radiotherapy. Potential synergistic effects of a radionuclide–bisphosphonate combination have been noted in patients (Soerdjbalie-Maikoe et al. 2002), and systemic radionuclide therapy as an adjuvant to local field external beam radiation demonstrated good therapeutic results (Smeland et al. 2003). New therapeutic options in the treatment of metastatic bone pain, such as endothelin-1 antagonists (Fizazi et al. 2003), osteoprotegerin (Mundy 2002), TGFβ mAbs (Kakonen et al. 2002), or FGF inhibitors (Nakamura et al. 1995) offer competition to developmental therapies, although synergistic effects are conceivable. This may be particularly true with FGF, since there is evidence of radiosensitization following FGF inhibition (Harari and Huang 2002; Chinnaiyan et al. 2003), and evaluation would certainly be of interest.
To summarize the experiences with 188Re-HEDP, use of this agent shows response rates and side effects comparable to the commercially available radiopharmaceuticals, including 89Sr, 186Re-HEDP, and 153Sm-EDTMP. Some researchers prefer radionuclides with low beta particles in treating bone pain palliation, because of the assumption bone marrow toxicity is lower for these agents. However, neither dosimetric data for radiation absorbed dose to the bone marrow (Maxon et al. 1990; Eary et al. 1993; Liepe et al. 2003a) nor clinical blood count depression (Liepe et al. 2005) has shown any significant differences between these agents. Other researchers suggest enhanced antitumoral effects using high-energy beta emitters and propose aggressive first-line treatment in the early disease stage instead of using these radiopharmaceuticals only in end-stage patients suffering intractable bone pain (Enrique et al. 2002; O’Sullivan et al. 2006). The wide range of the electrons of 188Re in osseous tissue lead to cytotoxic effects in the outer layers of the tumor, and repeated treatment of short intervals would prevent new tumor growth and eradication of deeper tumor layers (Palmedo et al. 2003). Another approach consists of including other treatment modalities such as autologous stem cell rescue or in combination with chemo or bisphosphonate therapy to a radionuclide treatment scheme (O’Sullivan et al. 2006; Lam et al. 2009). Future research should focus more curative effects of combination with radiosensitizer, for example, chemotherapy (Tu et al. 2001), or repeated treatments with 188Re-HEDP (Palmedo et al. 2003).
4.2 Marrow Ablation
Another application of 188Re that has been explored on a clinical basis in some detail is the ablation of marrow with a directly labeled NCA 95 antibody (Reske et al. 2001; Zenz et al. 2006). The use of 188Re-labeled antibody anti-CD20 (anti NCA95) was evaluated as an adjuvant for conditioning of leukemia therapy (Bunjes et al. 2001; Buchman et al. 2002). The results of leukemia therapy were quite impressive, but this approach was not recommended for routine use because of the persistently high renal radiation doses encountered. Because of the cost-effective routine availability of the 188W/188Re generator and facile preparation of directly 188Re-labeled anti CD20, this approach may still have promise if methods become available that could significantly increase renal clearance.
4.3 Peptides and Proteins
Well-established targeting strategies using peptides and proteins are also being explored with 188Re. In one example (Cyr et al. 2007), the 188Re-labeled P2045 peptide targeted to the somatostatin receptor (Magram et al. 2003) has been evaluated in Phase I studies for the targeting of small cells and on small-cell lung cancer. In another recent example, Phase I studies have recently been completed for the targeting of 188Re-labeled antibody PTI-6D2 to melanin excreted from metastatic melanoma cells (Klein et al. 2008). These studies are based on the pioneering preclinical studies focused on the targeting of 188Re antibodies to cancer-cell-expressing antigens (Dadachova et al. 2004a, b). In this non-randomized, open-label, single-assignment Phase I safety study, dose escalation studies are in progress in an estimated cohort of 21 patients to determine the safety, maximum tolerated dose, and any dose-limiting toxicities in patients with metastatic melanoma. Adjuvant use of 188Re agents in conjunction with chemotherapeutic regimens are also being explored and may offer important benefits.
4.4 Therapy of Liver Malignancies
Because of the widespread incidence of non-resectable primary hepatocellular carcinoma (HCC) in many countries and availability of only limited therapeutic options, the benefits for use of invasive radiotherapeutic methods has stimulated interest beyond the use of 131I-lipiodol (Biersack 2010). The important therapeutic benefits and ready cost-effective availability of an in-house generator system make the use of 188Re of great interest in the preparation of a number of radiolabeled agents in which strategies for the palliative treatment of liver malignancies have been explored (Bult et al. 2009). Tumor access has involved the traditional catheter-based intraarterial selective administration to the tumor-feeding arteries using various agents. The clinical strategies (Table 5) include the use of 188Re-labeled lipophilic agents for hepatocyte extraction and 188Re-labeled microspheres for arterial radioembolization. In addition, animal studies have also explored the therapeutic effectiveness of direct intratumoral injection of 188Re lipophilic agents. A number of 188Re-labeled agents have thus been developed and evaluated in either preclinical animal models or in clinical trials for assessment of their utility for non-resectable liver cancer therapy (Table 5).
Table 5
Examples of Rhenium-188-labeled agents for therapy of liver malignancies
Rhenium-188- Labeled Agent | Trial Status | Results/Reference |
---|---|---|
Lipiodol complexes | ||
Re-188 HDD/Lipiodol | Clinical | |
Re-188 DEDC/Lipiodol | Clinical | |
Re-1888-SSS/Lipiodol | Preclinical | |
Microspheres | ||
Re-188 B20 Particles | Clinical | |
Preclinical | ||
Agents for direct tumor injection | ||
Re-188-ECD-Lipiodol | Preclinical | Luo et al. 2009 |
Re-188 Microspheres | Preclinical | Wang et al. 1998a |
4.4.1 Lipiodol Complexes
Administration of the iodinated lipiodol agent results in hepatocyte extraction as a fluoroscopic target to evaluate hepatic tumors. Therapy of liver tumors with radioactive lipiodol analogs containing 131I is well know and, based on this strategy and other radioactive lipiodol analogs, can also be used. Development of 188Re-labeled lipophilic complexes (Jeong and Knapp 2008; Jeong et al. 2001a, b) for admixture with lipiodol and administration via liver tumor feeding arteries has been evaluated and demonstrated as an effective palliative therapy for treatment of both primary and metastatic liver cancers (Padhy et al. 2007, 2008 ; Bernal et al. 2007, 2008). The 188Re HDD/lipiodol formulation was developed and evaluated under a multi-center Phase I/II program orchestrated by the International Atomic Energy Agency (IAEA) (Padhy et al. 2007, 2008; Sundram et al. 2001, 2002, 2004; Kumar et al. 2005, 2006a, b, 2007). The 188Re-DEDC (Fig. 13) is another lipophilic 188Re-labeled agent which has been developed and evaluated for similar applications and has been evaluated in animal models and limited human trials (Boschi et al. 2004; Duatti et al. 2002). Another more recent approach has described the synthesis, animal evaluation, and human studies with an 188Re-SSS lipiodol agent (Garin et al. 2004a, b, 2005, 2006).
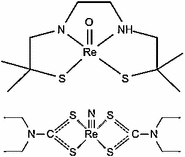
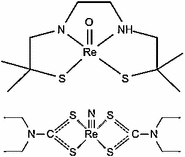
Fig. 13
Chemical structures of Re(VII)-HDD and Re(VII)-DEDC
4.4.2 Microsphere/Particle Radioembolization
The arteriole vascular trapping of radioactive microspheres and particles selectively administered through a hepatic artery is also an effective strategy for the delivery of beta irradiation for therapy of non-resectable liver tumors (Bult et al. 2009; Liepe et al. 2007; Wunderlich et al. 2000a).
Primary and secondary liver cancer is one of the leading causes of cancer-related deaths worldwide. For many patients with disseminated large bowel cancer, the liver represents either the dominant or only site of metastases, and is a major cause of patient mortality. For these patients with colorectal liver metastases, resection represents the therapy of choice but is achievable in only about 10 % of patients (Gilbert et al. 1984). Approximately 30 % of patients with resectable hepatic metastases survive 5 years after surgery. In the remaining 90 % of patients with non-resectable liver metastases, survival is poor, even after partial response to chemotherapy (Bismuth et al. 1996). The median survival of these patients ranges from 6 to 9 months depending on the volume of the metastases and histology of the original tumor (Cunningham et al. 2004). The current first-line mainstay for treatment of advanced liver metastases is systemic chemotherapy (Ong 2003). HCC is a malignant epithelial tumor arising from parenchymatous liver cells. Only a minority of patients with HCC fulfill the criteria for curative surgery, either partial liver resection or liver transplantation. Other treatment strategies have thus been explored which include induction of hepatic arterial embolization with or without chemotherapy (Bastian et al. 1998), local ablative therapies, such as radiofrequency ablation (RFA) (Ellis et al. 2004), laser-induced interstitial thermotherapy (LITT) (Vogl et al. 2004), and interstitial-seed brachytherapy (Ricke et al. 2004; Schwartz et al. 2002). External beam radiotherapy is effective in localized disease in doses above 50 Gy, but a key limitation is the tolerance of normal liver parenchyma to radiation with an acceptable dose for the whole liver of 35 Gy (Emami et al. 1991).
A particularly useful expected alternative treatment in many cases is the intraarterial administration of radioactive particles (selective internal radiotherapy = SIRT) of a size sufficient to lodge in hepatic end-arterioles. This treatment is based on the architecture of the vascular supply, since these tumors are usually richly vascularized and have an almost exclusively arterial blood supply. This contrasts to the normal liver, which receives most of its flow from the portal vein. A number of studies have presented encouraging results using radioactive particles in patients with liver metastases from colorectal cancer and primary HCC (Hosono et al. 1998). There have been many reports on the use of SIRT in patients with colorectal liver metastases or HCC (Hosono et al. 1998; Lau et al. 1999; Sundram et al. 2003; Liepe and Kotzerke 2005; Kennedy et al. 2006). Different radiopharmaceuticals have been used for this treatment, including 90Y microspheres, (Andrews et al. 1994), and 131I lipiodol (Hind et al. 1992), and different therapeutic efficacy has been observed. Using CT data as the response criteria, a partial response in 5 of 24 patients treated with 90Y microspheres was found (8 patients with progressive disease, 7 with stable disease, and 4 with minimal response) (Andrews et al. 1994). Other papers showed similar results with a 50 % decrease in tumor volume in 27 % (19/71) of patients (Lau et al. 1998). Goin et al. (2003) showed in 5 of 25 patients a complete response and in 19 % a partial response. Using 131I-lipiodol in patients with HCC, a significantly higher 3 year overall survival and disease-free survival was observed (Lau et al. 1999). Other authors reported a significantly better tumor response and survival in patients treated with lipiodol chemoembolization compared to chemotherapy (Llovet et al. 2002).
The published data from the largest study using SIRT included 208 patients treated with 90Y microspheres (Kennedy et al. 2006). The authors reported a median survival from 10.5 months in the responder group and only of 4.5 months in non-responders. Using computed tomography, a partial response was seen in 35 of patients, and in 91 using PET, and a reduction of carcinoembryonic antigen, a tumor marker, was achieved in 70 %. Commercial radiopharmaceuticals for this application have been available for several years, including the 90Y-labeled resin microsphere (SIR-Spheres®), with a particle size of 20–60 μm, and the 90Y-labeled glass microsphere (Theraspheres®) with a particle size of 20–30 μm. The very high cost is a major challenge for the broader use of these radiopharmaceuticals agents in routine practice, which lead to especially high therapy costs in Europe, for instance, in excess of 12,000 €/patient treatment, which has limited the broader, worldwide implementation of this radionuclide therapy.
An expected much more cost-effective therapeutic strategy for therapy of colorectal liver metastases or HCC is the use of the 188Re radionuclide, which represents an interesting potential alternative to 90Y or 131I (Raoul et al. 2010). Initial animal studies using a 188Re-labeled sulfide suspension developed for direct tumor administration showed in vitro stability more than 99 % over a 3 day period (Junfeng et al. 1999). In another study, Lee et al. (2002) documented the synthesis of an HDD-chelator (4-hexadecyl-1,2,9,9-tetramethyl-4,7-diaza-1,10-decanedithiol) and established the optimal conditions for radiolabeling with 188Re and preparation of homogeneous suspensions with lipiodol. This radiopharmaceutical was first introduced in clinical studies for intraarterial administration by an IAEA-sponsored group multi-center trial (See “Radionuclide Therapy of Bone Metastases Using Re-188 HEDP”), which encompassed clinical sites in eight different countries in Asia (Mongolia, China, India, Philippines, Singapore, Thailand, and Vietnam) and South America (Colombia) (Padhy et al. 2007, 2008). The initial data were encouraging, and the final results from this multi-center trial in 185 patients with inoperable HCC were published in 2008 (Bernal et al. 2007, 2008). The dosimetric approach developed for this study was based on the initial administration of a low-activity “scout” dose of 0.2 GBq (5.3 mCi) of 188Re-HDD/Lipiodol on the day of therapy (Zanzonica et al. 2008). Tolerable adsorbed dose estimates to the key organs for the therapy were 12 to the lung and 30 Gy for the liver, which led to a mean therapy activity of 3.7 GBq (100 mCi) (range from 0.78 to 13.4 GBq). While the bone marrow was never identified as the dose-limiting organ, the liver parenchyma was dose limiting in 68 of patients and the lung in 32 %.
A single treatment was administered to 134 patients (72), and 42 patients (23) received 2 treatments, 8 patients (4 %) 3 treatments, and 1 patient 4 treatments, respectively. The side effects included liver pain in 25 patients (14), vomiting in 10 (5), and a mild or moderate fever in 34 (18), within the first day. Liver toxicity was the most frequent observation, with an increase in bilirubin levels. Deaths at a rate of 15 % were recorded in the first 2 months after therapy, which were directly caused by the tumor in 10 cases and by other factors in 18 patients. Liver toxicity was not more prevalent in the subgroup of patients in whom the liver was the dose-limiting organ. Pneumopathy occurred in 3 cases, one patient with only 1 treatment and higher liver-lung shunt (the dose to the lung was exceeded 12 Gy), and in 2 patients following repeated treatment, despite low lung uptake (Bernal et al. 2008). Tumor response in 88 patients was evaluated by CT and showed an objective response rate in 25, a stable disease in 53, and tumor progression in 22 %. Complete response was recorded in 3 patients. With a median follow-up of 455 days, estimated median survival was 256 days. The estimated 3, 6, 12, and 24 month overall survival values were 93, 60, 46, and 23 %, respectively. In addition, patients with portal vein thrombosis tolerated the therapy well. In a dosimetric sub-study the researchers found statistically significant differences between the median doses received by progression tumors (18 Gy) compared to the dose received by non-progressive tumors (57 Gy). There existed a significant difference (p = 0.006) in overall survival between patients who received a tumor dose <30 Gy versus those who received more than 30 Gy (Bernal et al. 2008).
In a comparative study using 1.9 GBq of 131I-lipiodol, it was documented that use of 3.56 GBq 188Re-HDD/lipiodol yielded lower cytotoxicity and a lower radiation exposure for an expected higher tumor-killing effect in comparison to 131I-lipidol (De Ruyck et al. 2004). A dose escalation study in patients with cirrhosis characterized as Child A described a tolerated activity of 7.4 GBq (200 mCi) of 188Re-HDD/lipiodol and projected that an even higher activity was possible (Lambert et al. 2006). The Ghent group (Lambert et al. 2009) reported the results of 70 patients who had been given one to four treatments with 3.7–7.4 GBq (100–200 mCi) of 188Re-HDD/lipiodol. Toxicity was scored within 10 weeks after the therapy. In 20 of 68 patients, Grade 3 or 4 toxicity, using Common Terminology Criteria for Adverse Events, CTCAE, vol. 3 (Trotti et al. 2000), were found. Radiological response was assessed on contrast-enhanced CT or MRI images using RECIST criteria. Out of 76 accessible treatment sessions, 69 patients had stable disease, 4 had complete or partial response, and 3 suffered progressive disease. Lambert et al. (2003); Sundram et al. 2003) also used this 188Re radiopharmaceutical in HCC patients. A biodistribution study documented the predominate elimination of the activity through urinary excretion. The mean renal clearance of 44.1 %ID within the first 76 h suggested excretion of oxidized free 188Re-perrhenate and may be evidence of suboptimal stability of 188Re-HDD/Lipiodol in vivo.
The use of 188Re-labeled microspheres has been suggested as a more optimal alternative to the use of 188Re-lipiodol analogs. In this case, the administered agent is physically trapped in the microvascular rather than targeting uptake to the hepatocytes. Results obtained with 188Re-human serum albumin (HSA) microspheres in animal studies demonstrated high >90 % in vivo stability. In vitro stability investigated in human plasma, blood, and saline for 30 h was >88 % (Wunderlich et al. 2000b). The first human applications of 188Re microspheres in 8 patients (14 treatment sessions) with hepatocellular cancer or liver metastases from colon cancer revealed a low urinary radioactivity excretion rate following administration of 188Re-HSA microspheres (8.5 ± 3.6 % of administered activity within 96 h) (Liepe et al. 2005). In contrast, the urinary excretion rate of 188Re-HDD/Lipiodol was much higher with 44.1 ± 11.7 % of the administered activity excreted in the urine within 72 h. These data reflect a higher effective half-life of 15.7 h in the total body for 188Re-microspheres in comparison to 14.3 ± 0.9 h for 188Re-HDD/lipiodol. An explanation for this difference in urinary excretion is the physical characteristic of these two pharmaceutically diverse agents. Lipiodol is an emulsion of iodized ethyl esters of fatty acid of poppy seed oil, which essentially represents a more “chemical” embolization agent with a high viscosity (Nakajo et al. 1988). Intraarterial injected lipiodol flows retrograde into the portal venules through hepatic sinusoids and with flow antegrade through the peribiliary vascular plexus (Nakamura et al. 1988). This fact indicates only a weak fixation of this agent in the tumor microvasculature capillary system. In contrast, HSA microspheres represent “physical” embolization agents, which are embolized and physically trapped in the capillary vascular plexus (Fig. 14).
