Stage
T
N
M
Median
Survival, months
Description
IA
T1
N0
M0
24.1
Tumor limited to the pancreas, ≤2 cm in greatest dimension, resectable
IB
T2
N0
M0
20.6
Tumor limited to the pancreas, >2 cm in greatest dimension, resectable
IIA
T3
N0
M0
15.4
Tumor extends beyond the pancreas, but does not involve the celiac axis or superior mesenteric artery, resectable
IIB
T1, T2 or T3
N1
M0
12.7
Regional lymph node metastasis
III
T4
N0, N1
M0
10.6
Tumor involves the celiac axis and superior mesenteric artery, unresectable
IV
any T
N0, N1
M1
4.5
Distant metastasis
If the tumor is obstructing the bile duct, a stent can be placed to relieve the blockage using nonsurgical approaches. If a patient develops gastric-outlet obstruction, treatment may include duodenal wall stents or percutaneous endoscopic gastrostomy placement for decompression. Occasionally, a patient may need surgery to create a bypass (biliary bypass or gastric bypass) to manage obstructive jaundice and gastric outlet obstruction.
In the US, only about 15–20 % of pancreatic cancer cases are diagnosed early enough to be eligible for surgery. The majority of patients diagnosed with pancreatic cancer already present metastatic disease or they later develop metastatic disease. This is mainly in the liver and peritoneal cavity. The treatment of these patients remains palliative, and pain management is one of the most important aspects of care, with pancreatic cancer causing severe pain in 50–70 % of patients. This type of pain is multi-factorial and may be caused by infiltration of nerve sheaths and neural ganglia, increased ductal and interstitial pressure, and gland inflammation (Staatas et al. 2001). The current management of pancreatic pain starts with non-opioid analgesics, such as nonsteroidal anti-inflammatory drugs (NSAIDs), and progresses to increasing doses of opioid analgesics. For pain that does not respond to drugs, or when oral or topical medication leads to unacceptable side effects, a nerve block may be performed by injecting alcohol under endoscopic ultrasound or CT guidance into the nerves (celiac plexus) that carry painful stimuli from the diseased pancreas to the brain (Arcidiacono et al. 2011). This procedure, known as celiac plexus neurolysis, does provide temporary pain relief, but the benefit is limited and continued use of analgesics is often still necessary.
Thus, based on the current standard of care described above, new treatment approaches that would offer both local tumor control and palliation of symptoms would be beneficial to advanced pancreatic cancer patients. HIFU was introduced relatively recently as a modality for ablation of unresectable pancreatic tumors to reduce the tumor size and achieve pain relief. The first clinical trials were performed in China and have been reported in Chinese literature since 2001 (Jang et al. 2010), with the first publication in English literature in 2005 by Wu et al. Currently, HIFU treatment of advanced pancreatic cancer is still widely available in China, with limited availability in South Korea, Japan and Europe.
5.2 Devices and Methods
There are three US-guided HIFU devices that are commercially available outside of China for treatment of pancreatic tumors, all manufactured in China: The FEP-BY-02 HIFU tumor therapy device (Yuande Biomedical Engineering Limited Corporation, Beijing, China), HAIFU (Chongqing Haifu Technology Co.,) and HIFUNIT-9000 (Shanghai A&S Sci-Tech Co., Ltd, Shanghai, China). All devices operate at similar ultrasound frequencies (0.8–1.6 MHz) and similar acoustic powers (up to 300 W). In all three devices, an ultrasound imaging probe is inserted into the opening at the center of the HIFU transducer. B-mode ultrasound serves three purposes in these devices: visualization of the target, monitoring tissue changes during treatment and assessment of the treatment outcome. Unfortunately, to date, B-mode ultrasound imaging can neither provide a direct map of the thermally ablated region, nor does it have the capability of performing tissue thermometry. However, it does provide real-time imaging using the same energy modality as HIFU. This is a significant benefit because: 1. adequate ultrasound imaging of the target suggests that there is no obstruction (e.g., bowel gas or bone) to ultrasound energy reaching the target, and: 2. the risk of causing thermal injury to unintended tissue is minimized. The appearance of a hyperechoic region on the ultrasound image during treatment is one method often used for confirmation of general targeting accuracy and prediction of ablation efficacy. This region has been shown to correspond to the formation of boiling bubbles at the focal region when tissue temperature reaches 100 °C (Khokhlova et al. 2011).
Two recent studies attempted to evaluate the ultrasound reflectance change during HIFU therapy of pancreatic neoplasms and to correlate the change with the degree of tumor ablation (Wang et al. 2012; Ge et al. 2013). Wang et al. (2012) found a strong correlation between the appearance of a bright hyperechoic region and the increase in both average ultrasound power and total ultrasound energy delivered to the target. This was consistent with the hypothesized formation of boiling bubbles at the focus. However, the local response measured by CT in terms of tumor size 1-month post treatment in 136 patients enrolled in the study did not correlate with the change in reflectance. This lack of correlation may be related to the assessment criteria for local response: the change in tumor size would not accurately reflect the effects of HIFU ablation due to tissue swelling and imperfections in the CT evaluation. In the study by Ge et al. (2013), the extent of tumor ablation effects following HIFU in 31 patients was assessed by enhanced CT. A good positive correlation was found between the variation of ultrasound reflection and tumor ablation ratio.
The operation characteristics of the two most widely used treatment systems – FEB-BY-02 and Haifu – are very similar, but there are some notable differences. The FEB-BY-02 system uses a 1 MHz transducer (aperture of 37 cm and a focal distance of 25.5 cm) consisting of 251 elements, all of which are driven in phase. The focal area has a width of 3 mm and axial length of 10 mm at −6 dB level. The change in the focus position in tissue is achieved by mechanical scanning of the transducer array in 3D from one treatment spot to the next. The treatment spots are placed in overlapping fashion: The interval spacing in the lateral dimension is 4–5 mm, in the axial direction – 6–8 mm. The system has two identical transducers for two patient positioning options. One transducer is located below the treatment table, in a water bath, and the patient is positioned prone on the table. The other transducer is located above the treatment table, and the patient is positioned supine. Acoustic coupling in this case is achieved by pressing a water-filled balloon onto the patient’s abdomen. This position was shown to be beneficial as it allows compression of the bowel and intestinal loops, as well as reducing the amount of gas in the acoustic pathway. The sonications are usually pulsed to allow ultrasound imaging between HIFU pulses, with pulse lengths of 150–1000 ms, duty factors of 33–60 % and 50–80 pulses per spot. For treatment planning an in-situ target acoustic energy dose (in J per spot) is set at 500–1250 J, taking into account the attenuation of the intervening tissues at each focal depth. The required output electric power is then calculated accordingly, and the treatment is delivered automatically spot by spot. In most studies, therapy is divided into several 60-min long sessions and performed without sedation or anesthesia (Xiong et al. 2009; Hwang et al. 2009; Zhao et al. 2010).
The HAIFU system offers the choice of three single-element HIFU transducers with 12 cm aperture, with different focal distances (9 cm, 13 cm or 16 cm) and different frequencies (0.8–1.6 MHz) for tumors located at different depths. The target tissue is exposed to focal peak intensities from 5000 to 20,000 W cm−2, depending on the focal depth (Wu et al. 2004). The transducers are located below the treatment table in a water bath, with an expandable water balloon on top for compression; the patient is positioned prone for HIFU exposures. The treatment planning and pulsing is performed differently to that by the FEB-BY device; using US images of the tumor, it is divided into several slices with 5-mm separation. The transducer is then continuously powered as the HIFU beam is scanned at the speed of 0.5–3 mm/s in successive sweeps from the deep to shallow regions of the tumor. After each sweep the HIFU transducer is turned off and the changes in tissue reflectivity are evaluated; if the increase in hyperechogenicity is not observed, the sweep is repeated until the tissue is considered completely ablated. The major procedural difference compared to the operation of the FEB-BY-02 device is that the ablation is usually performed in a single session, under sedation or general anesthesia. Sedation is performed to avoid deep visceral-type pain that is experienced during treatment by this device, most probably due to the higher rate of energy deposition compared to FEB-BY-02 (continuous sonication vs pulsed sonication), and for immobilization purposes.
Recently, an MR-guided HIFU system ExAblate (InSightec, Israel) was used to treat a limited cohort of locally advanced stage pancreatic cancer patients in Italy (Anzidei et al. 2014). The transducer used in ExAblate system is a 1.1 MHz 206-element annular phased array (aperture 12 cm, radius of curvature 16 cm) that allows electronic beam steering in the axial direction (focal distance 60–200 cm). The transducer is positioned below the treatment table, the patient is positioned prone and a convex gel pad is used to compress the abdominal wall. A 3 T medical MR scanner (GE Medical Systems) is used for treatment planning, as well as real-time measurement of tissue temperature during treatment using the proton chemical shift method (Jolesz et al. 2005). Sonications are administered to a given spot until the thermal ablation temperature threshold (65 °C) is reached in the focal area according to the temperature maps. Follow-up evaluation is performed by contrast-enhanced MRI, allowing measurement of the non-perfused volume of the tumor – likely a more accurate method of measuring long-term local response compared to tumor volume measurement.
5.3 Preclinical Studies
The first preclinical in-vivo studies of HIFU ablation of the pancreas utilized the swine model because of its size and similarity to human anatomy (Hwang et al. 2009; Xie et al. 2010; Liu et al. 2011). The animals did not bear tumors in the pancreas, therefore it was not possible to evaluate HIFU therapy survival; however, the main goal of these studies was to systematically evaluate the safety and efficacy of HIFU ablation of the pancreas. In the earliest study the pancreas of 12 common swine was successfully treated in-vivo using the FEP-BY02 device. No significant adverse effects, such as skin burns or evidence of pancreatitis, were observed during the 7-day post-treatment observation period (Hwang et al. 2009). Three in-situ total acoustic energy doses were tested – 750 J, 1000 J and 1250 J. Only the two higher doses resulted in macroscopic ablation of the pancreas. The highest dose resulted in a greater degree of ablation, but was also associated with greater collateral damage; minor skin burns, abdominal wall injuries and bowel ulceration.
A subsequent study by another group also in swine used the HAIFU device. They used both light microscopy and electron microscopy to confirm that complete necrosis is confined to the target regions, with clear boundaries and no damage to adjacent tissues (Xie et al. 2010). Pancreatitis was an important safety concern because the mechanical effects of HIFU can cause cell lysis and release of pancreatic enzymes. Although the cavitation, or boiling bubble activity, during HIFU was confirmed by electron microscopic examination (intercellular space widening and formation of numerous vacuoles of different sizes in the cytoplasm), pancreatitis was not observed, thus confirming the safety of the treatment protocol. Another preclinical study showed that a combined treatment of HIFU ablation followed by radiation therapy may be a promising method. The injury to the targeted pancreas was increased compared to either modality alone, without additional injury outside of the targeted region (Liu et al. 2011).
One other important safety consideration for HIFU ablation of pancreatic neoplasms is maintaining the endocrine and exocrine functions of the pancreas. These both play an important role in metabolism of glucose, fat and protein. A recent study in felines (Mao et al. 2014) addressed this concern by monitoring serum glucose and amylase levels after HIFU ablation of 25 % and 50 % of pancreatic tissue for 21 days. Both serum amylase and glucose levels had a short peak after the treatment, but returned to normal 14 days and 3 days after the treatment, respectively, thus not presenting a threat to the patient’s health. Histopathological study also revealed that new pancreatic ducts formed on the 7th day, and fibrous tissue completely substituted destroyed parenchyma by the 21st day.
In order to evaluate the effect of HIFU on pancreatic tumor and the benefit in survival, a study in a small animal tumor model was performed (Jiang et al. 2013). Jiang et al. used a nude mouse model subcutaneously injected with SW1990 human pancreatic cancer cells. The tumors were ablated with US-guided HIFU, and the success of treatment was confirmed by the increase in tissue reflectivity in the ultrasound images. Following HIFU, a 100 % reduction in tumor volume was observed within 28 days after treatment. The limitation of this study, however, was that the mouse model did not exhibit metastatic disease, the primary concern in most pancreatic cancer patients.
5.4 Ultrasound-guided HIFU System Clinical Trials
HIFU was first used for the palliative treatment of pancreatic cancer in an open-label study in China in 251 patients with advanced pancreatic cancer (TNM stages II–IV) (He and Wang 2002). HIFU therapy resulted in significant pain relief in 84 % of the patients. In some cases, significant reduction of tumor volume was achieved without any significant adverse effects or pancreatitis, which appeared to prolong survival. Multiple nonrandomized studies followed; they were reported in the Chinese literature (Wang and Sun 2002; Xie et al. 2003; Xiong et al. 2005; Xu et al. 2003; Yuan et al. 2003), and later in English literature (Wu et al. 2005; Xiong et al. 2009; Orsi et al. 2010; Wang et al. 2011; Sung et al. 2011). These studies provided additional evidence showing that HIFU does provide palliation of tumor-related pain, and also achieves local disease control in some cases, but does not cause adverse effects. These trials are discussed in more detail below.
The general patient inclusion criteria were similar in all trials: Diagnosis of unresectable stage III-IV pancreatic cancer and adequate physical condition to undergo the treatment. Before the treatment, bowel preparation was performed which included preoperative fasting and catharsis. In some cases, placement of a nasogastric tube to evacuate the gas from the stomach and colon was required. These preparations are of great importance to the success of treatment as gas and food debris harboring bubbles are known to reflect and redistribute HIFU energy, preventing it from reaching the target, also causing adverse effects such as burns and bowel perforation. To further eliminate the bowel gas from the acoustic pathway, expandable water balloons were used in all studies to compress the bowel and the intestinal loops. Figure 5.1 illustrates patient positioning during HIFU procedure. In some studies (Wu et al. 2005; Wang et al. 2011), to reduce the likelihood of pancreatitis, 14-peptide somatostatin, a strong inhibitor of pancreas exocrine secretion, was administered before the HIFU treatment.
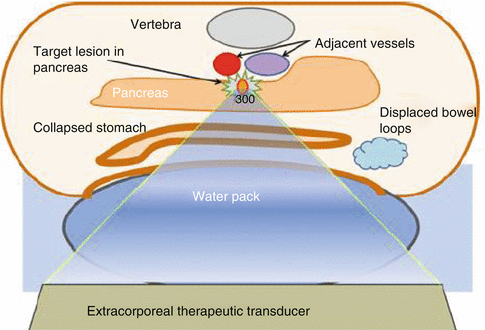
Fig. 5.1
A schematic representation of patient positioning for HIFU ablation of pancreatic neoplasms. Evacuation of gas and food debris from the acoustic pathway is achieved by bowel preparation (fasting, catharsis) and water balloon placement (Reproduced with kind permission from Springer Science + Business media from Jung et al. 2011)
The treatment outcome was measured in terms of pain palliation, using a numerical rating scale from 0 to 10 (0: no pain, 10: worst pain imaginable), as well as local tumor response, using contrast-enhanced CT or MRI to measure unperfused volume of the tumor, and finally survival. Table 5.2 summarizes the findings of these studies. Notably, pain relief was achieved in most patients immediately after or within 24–48 h after HIFU ablation, and the response was long-lasting. Some studies reported complete remission of pain for the entire follow-up period of several months (Wu et al. 2005), in others, pain relief lasted for an average of 10 weeks (Wang et al. 2011). For comparison, celiac plexus block usually provides pain relief for 3–4 months, but carries a higher risk of complications (Rykowski and Hilgier 2000). As mentioned previously, the mechanism for pain relief remains unknown, but it is hypothesized to be related to the ablation of the nerve fibers in the tumor, and possibly thermal injury to the celiac plexus. Interestingly, as noted in the study by Xiong et al. (2009), pain relief was observed both in patients who had local tumor response (unperfused areas on contrast-enhanced CT after HIFU ablation) and in those who did not, which suggests that nerve fibers may be more susceptible to damage by HIFU.
Table 5.2
Clinical trials of ultrasound-guided HIFU ablation for palliative treatment of pancreatic cancer
Author |
N
![]() Stay updated, free articles. Join our Telegram channel![]() Full access? Get Clinical Tree![]() ![]() ![]() |
---|