m, which is sufficient to visualise cardio-myocytes. The obtained volumes are compared with 2D histological examinations, which serve as a basis for interpreting the 3D X-ray phase-contrast results. Our experiments show that 3D X-ray phase-contrast micro-CT is a viable technique for investigating the 3D arrangement of myocytes ex-vivo at a microscopic level, allowing a better understanding of the 3D cardiac tissue architecture.
Keywords
Phase-contrast imagingHistologyCardio-myocyte1 Introduction
Cardiovascular diseases remain one of the most serious health problems in the world, motivating research that deepens our understanding of the myocardial function. This requires a good knowledge of the myocardial architecture, especially the myocyte architecture, to understand relations between mechanical function, hemodynamics and adaptive structural changes in cardiac diseases.
Cardiac muscle cells have diameters in the range of 10–20
m and lengths around 100
m. To image them, a resolution in the
m range is required. Current imaging techniques can deliver either very high resolution but only in a small field of view, or the entire heart can be imaged to see the overall organisation of the myocytes, but at a resolution that does not show how individual myocytes are arranged.



Optical images of histological sections can deliver high resolution for ex-vivo 2D imaging, but they can suffer from distortions due to cutting the thin samples. Another optical technique, Polarized Light Imaging (PLI), can be used to obtain maps of the orientation of myocardiac cells. In [7, 9], a fetal heart was divided into a set of parallel contiguous slices and each one was imaged with PLI, thus constructing a 3D map of the myocyte orientations in the entire heart at a resolution of
m
m
m. This resolution remains insufficient for visualizing individual myocytes, and what is actually seen is the averaged orientation of a population of myocytes in each voxel.



Compared with the previous two techniques, Magnetic Resonance Imaging is non-invasive and can be used in-vivo to image the entire heart. Diffusion Tensor MRI (DT-MRI) can recover the dominant orientation of myocytes in each voxel because of the stronger diffusion of water molecules in the direction parallel to that of the myocytes. DT-MRI can thus map myocyte orientations throughout the entire heart in-vivo and can serve to detect abnormalities [8, 15]. However, the typical spatial resolution is low (1 mm
mm
mm) therefore the 3D organization of myocytes at a microscopic level remains unknown. High-resolution MRI setups such as [1] can achieve
m
m
m or
m
m
m voxel sizes, however this remains above the diameter of cardio-myocytes.








A new method that has great potential for applications in biomedical imaging is X-Ray Phase Contrast Imaging (PCI). Just as conventional transmission-based X-ray imaging, PCI can be combined with Computed Tomography (CT) techniques to obtain 3D images, and a better contrast is obtained in soft tissue compared to classical CT. Several techniques are now available to exploit and visualize the phase-contrast, of which we mention propagation-based imaging (PBI), also known as in-line holography, which yields high spatial resolution without the need for complex instrumentation. PBI is useful for imaging cardiac tissue because it enhances contrast at myocyte boundaries [4].
Allowing high contrast 3D visualization of thick and complex samples at high spatial resolution, X-Ray PCI can image a wide range of tissues and organs not only ex-vivo but also in-vivo. In [10], angiographies in the mouse brain were performed at 6
m resolution. However, most PBI studies use higher resolutions, such as [5] who have visualised ex-vivo 3D osteon morphology with a spatial resolution of 1.4
m. Also interesting are the experiments of [10], who were able to visualize subtle details in the rat brain through a phase retrieval method that allows PBI-CT through a single distance PBI image, with a reduction in absorbed dose and acquisition time. In-vivo studies with X-Ray PCI on humans have also been conducted, the first clinical trials for mammography being described in [2]. They show that mammographies with synchrotron radiation can be used to clarify the diagnosis in patients with suspicious breast abnormalities identified by combined digital mammography and ultrasonography.


In our study, we investigate the 3D cardiac tissue structure using X-Ray micro-CT phase contrast imaging based on in-line propagation implemented at the European Synchrotron Radiation Facility (ESRF) [11]. The technique allows to detect the microscopic-scale boundaries of the myocytes in the heart tissue [4].
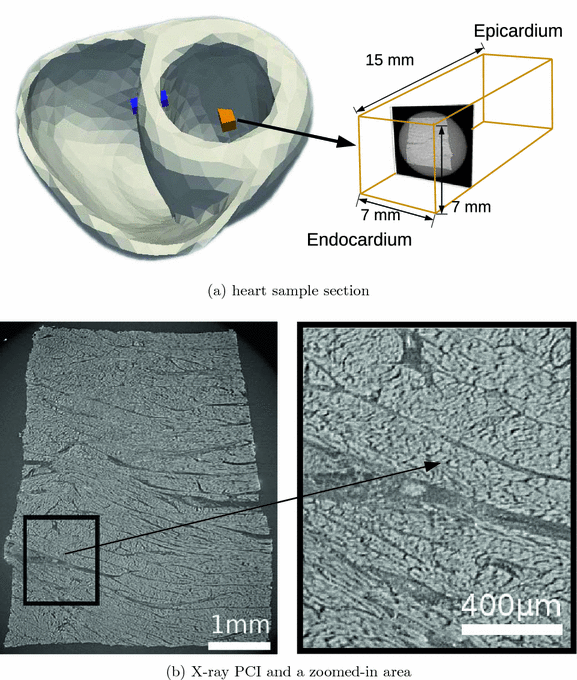
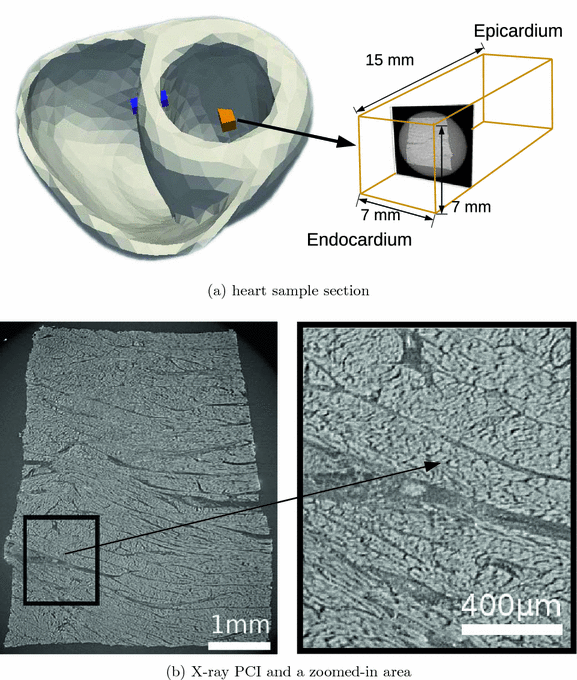
Fig. 1.
(a): schematic representation of the heart sample section at the junction between the apical third and the equatorial third. The sample has a height of 15 mm, corresponding to the thickness of the left ventricle wall, and the sides of about 5–6 mm (7 mm including the outer edges of the image). It is imaged at a resolution of 3.5
m. (b) left: X-ray PCI viewed in a plane orthogonal to the epicardium-endocardium direction. The bundle of myocardial cells grossly follows the curvature of the ventricular wall and corresponds mostly to a circular arrangement around the ventricle. (b) right: at a finer scale, the orientation of myocardial cells can be refined, they are obliquely cut. Myocardial cells appear in white, separated by short and thin dark gaps. The larger, roughly linear dark gaps separate bundles of myocardial cells. The lumen of a blood vessel is also visible as a thick dark gap in the middle of the image.

2 Materials and Methods
Human heart samples were supplied by the Medico-Legal Institute of Lyon IML HCL (
) and were collected during a medical-legal autopsy of a subject who suffered a violent death. From this heart, 9 samples were collected from the septal, anterior, lateral, and posterior left ventricle, with heights of 11–20 mm and 6 mm sides for imaging.
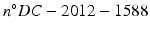
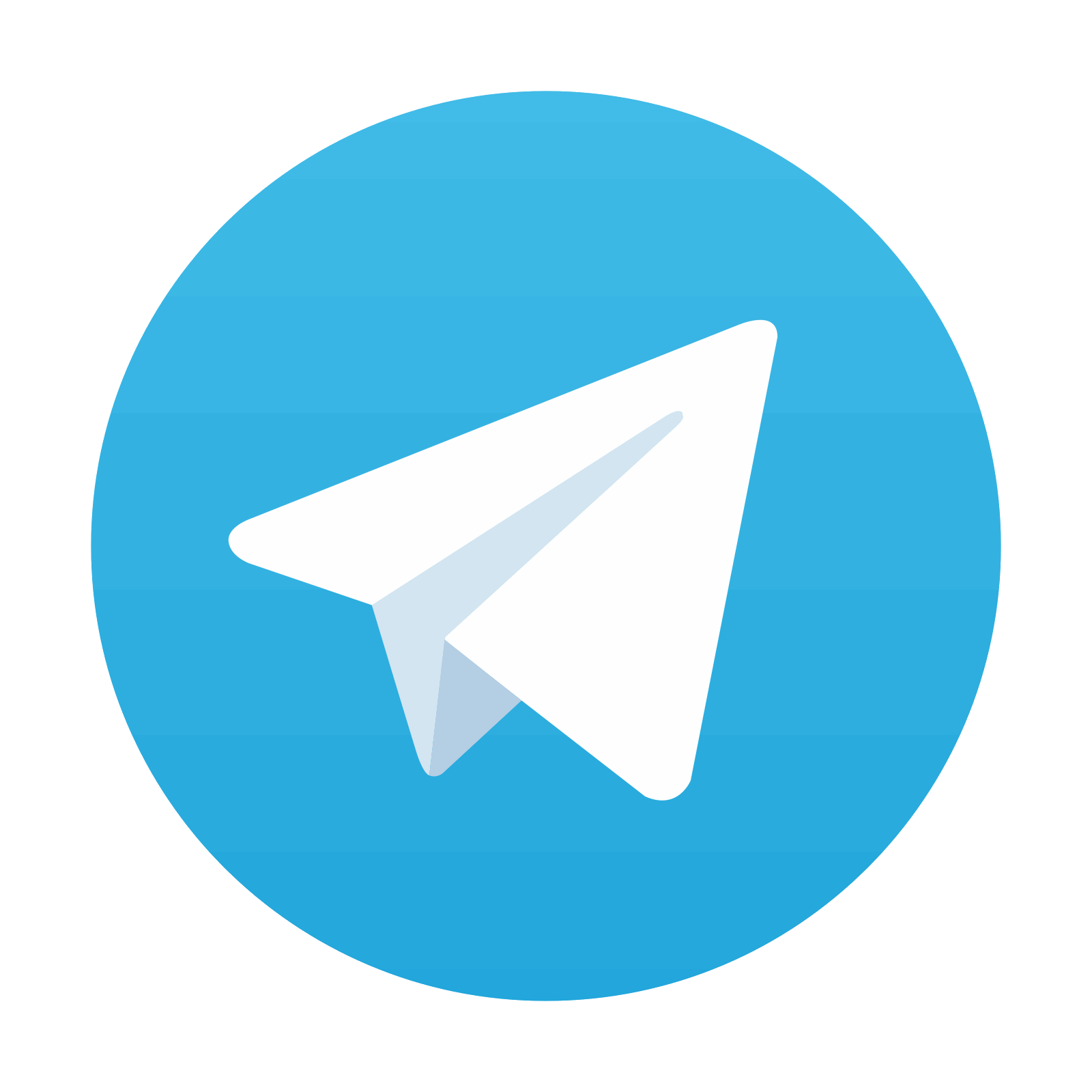
Stay updated, free articles. Join our Telegram channel

Full access? Get Clinical Tree
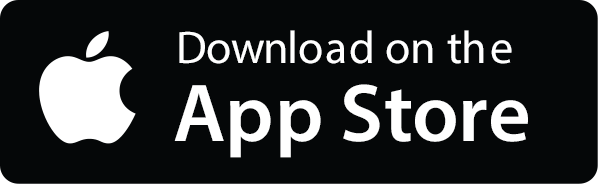
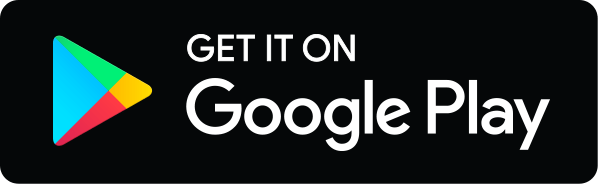