Fig. 2.1
Penetrating keratoplasty. Clinical photograph (a) of a patient at the conclusion of a corneal transplant surgery with a 10–0 nylon running suture. The anterior segment OCT image (b) of an eye with healed corneal transplant shows the graft–host junction (arrows)
Femtosecond laser technology has recently enabled femtosecond-assisted penetrating keratoplasty (FAKP). The femtosecond laser is a type of laser that can produce pulses of light of extremely short duration. The application of femtosecond laser in surgery results in no thermal effect or shock wave, so that this laser is unlikely to cause tissue injuries outside the irradiation area of the laser beam. The femtosecond laser is ablative and utilized to perform incision with varying size and shape of the cuts of the corneal tissue. The laser can be used to accurately cut a more complex shape of both the donor tissue and the host tissue, such as a top hat, mushroom, Christmas tree, or zigzag configurations using software products, such as the IntraLase (AMO, Inc, Santa Ana, CA, USA), which is otherwise known as IntraLase-enabled keratoplasty (IEK). These arrangements provide better fixation of the corneal graft. The graft interfaces are readily visible on OCT, but residual host corneal tissue can reflect light and create “noise” that degrades anatomic detail on Pentacam-Scheimpflug imaging (Fig. 2.2).
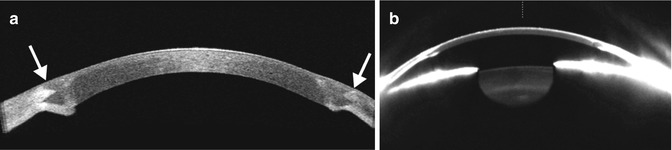
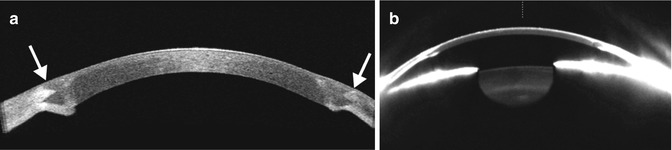
Fig. 2.2
FAKP with zigzag IEK. Anterior segment OCT image (a) shows the graft–host junctions are clearly depicted as zigzag patterns between the donor and the host matching at the peripheral edge of the graft (arrows). The Pentacam-Scheimpflug image (b) of the same eye shows that the remaining host corneal tissue reflects light and creates “noise” in the image and loss of anatomic detail
A rare but potentially vision-threatening complication of a retrobulbar or peribulbar block performed prior to corneal transplant surgery is intraorbital hemorrhage. Expanding proptosis, pain, ophthalmoplegia, and decreased vision are the immediate signs of a retrobulbar hemorrhage. An orbit CT without contrast can be obtained to confirm and localize the hemorrhage, which appears as hyperattenuating collections in the acute setting (Fig. 2.3). An emergent lateral canthotomy and inferior cantholysis may be required to decompress the orbit and prevent an ischemic optic neuropathy or a central retinal artery occlusion.
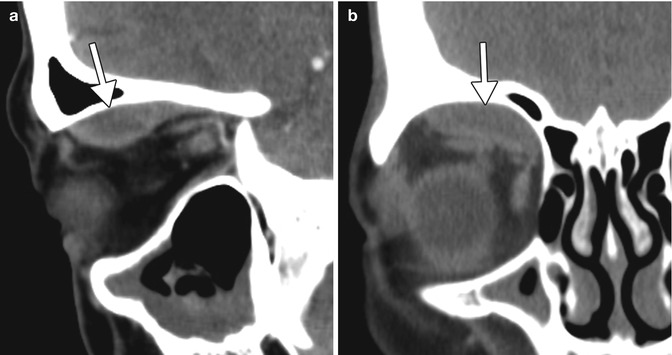
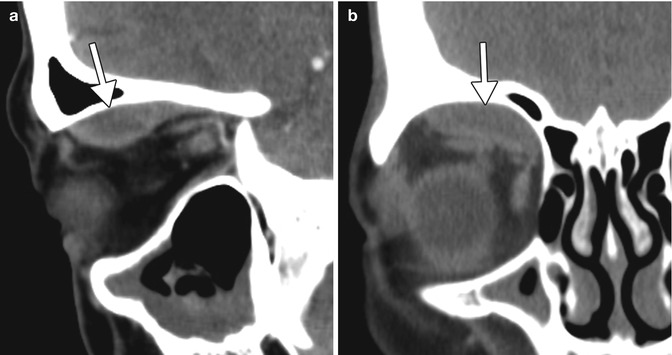
Fig. 2.3
Intraorbital hemorrhage secondary to retrobulbar block. Sagittal (a) and coronal (b) CT images show a subperiosteal hematoma in the right superior orbit (arrows)
One of the most dreaded complications of penetrating keratoplasty is expulsive suprachoroidal hemorrhage. This occurs due to spontaneous bleeding of a choroidal blood vessel after decompression of the globe and has an incidence of approximately 0.5–1.1 % of corneal transplantation cases. After phacoemulsification and foldable IOL implantation, UBM typically reveals shifting of the iris posteriorly, and deepening of the anterior chamber by 850 micrometers on average. Risk factors for suprachoroidal hemorrhage include older age, a history of glaucoma or myopia, hypertension, diabetes mellitus, generalized atherosclerosis, and Valsalva maneuver in the context of an open eye, such as sudden coughing during the surgery. Preventative measures include proper positioning of the patient, adequate anesthesia, best possible control of blood pressure, and reducing the intraocular pressure prior to surgery with medication, such as intravenous mannitol. Postoperatively, a noncontrast CT of the orbit can be obtained to demonstrate the extent of the hemorrhage, which can project beyond the margins of the globe through the corneal incision (Fig. 2.4). Otherwise, B-scan ultrasound is the imaging modality of choice for assessing the status of the retina and evaluating the potential for visual restoration.
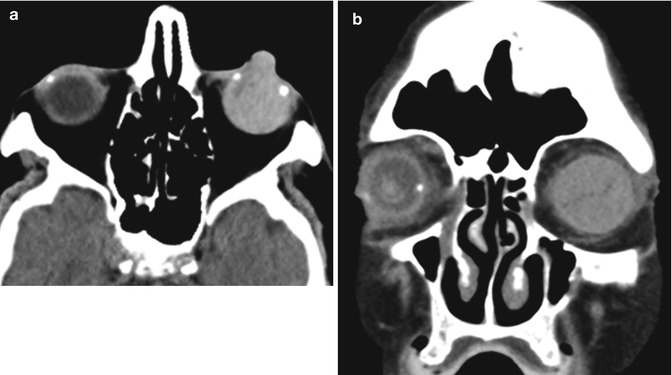
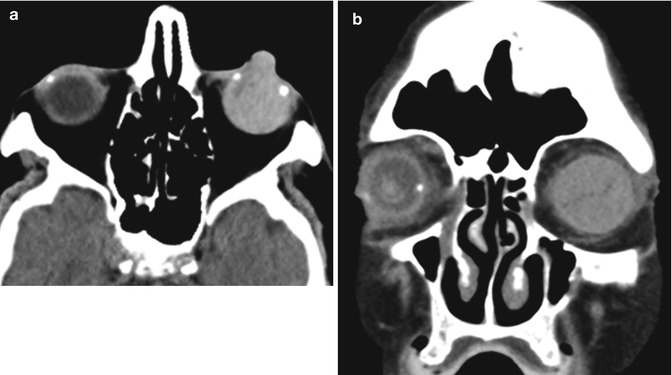
Fig. 2.4
Expulsive hemorrhage following penetrating keratoplasty. Axial (a) and coronal (b) CT images show diffuse hyperattenuation within the left globe with protrusion through the corneal dehiscence
Other early postoperative complications of penetrating keratoplasty include wound leakage and hypotony, persistent epithelial defect, infection, elevated intraocular pressure, and primary graft failure, that is, failure of the donor graft to achieve optical clarity. The most devastating complication is endophthalmitis (Fig. 2.5), and its incidence after penetrating keratoplasty ranges from 0.2 to 2.0 %. The source of infection is usually the patient’s periocular flora or a contaminated donor tissue. Risk factors for the development of endophthalmitis include concomitant vitrectomy, aphakia, history of previous surgery or inflammation, and corticosteroid use. Endophthalmitis is a clinical diagnosis and a view to the retina is usually precluded by the presence of intense vitritis. Thus, although B-scan ultrasound is not diagnostic of endophthalmitis, it can show the status of the retina and choroid prior to any surgical intervention. Orbital signs and symptoms (painful proptosis, ophthalmoplegia) suggest spread of the infection to the orbit and panophthalmitis. In such cases, orbital CT or MRI is indicated to assess the extent of orbital involvement and rule out the presence of an abscess. Treatment depends on the severity of the infection but generally includes removal of the graft, repeat penetrating keratoplasty, intravitreal injections of antibiotics, and, sometimes, pars plana vitrectomy. The main differential diagnosis for infectious endophthalmitis is toxic anterior segment syndrome. Toxic anterior segment syndrome is a sterile postoperative inflammatory reaction caused by a noninfectious substance that enters the anterior segment. The process typically begins 12–48 h after anterior segment surgery, is Gram stain and culture negative, and often responds to steroid treatment.
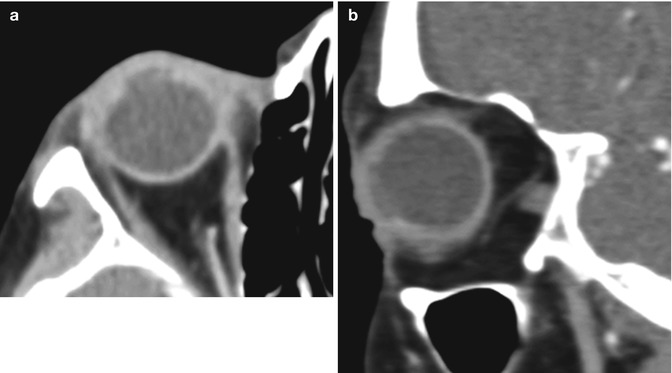
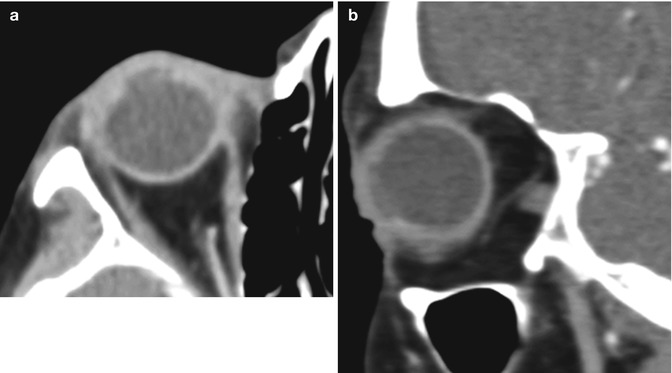
Fig. 2.5
Endophthalmitis following penetrating keratoplasty. Axial (a) and sagittal (b) contrast-enhanced CT images show irregularity of the right cornea as well as diffuse intraocular hyperdensity, uveoscleral thickening, and preseptal edema. The patient was subsequently treated via removal of the corneal graft, repeat penetrating keratoplasty, and intravitreal injections of voriconazole and vancomycin
Corneal melt (keratolysis) consists of a breakdown of the cornea with expansion of the anterior chamber, which can occur as a complication of corneal transplant surgery. Inflammation at the limbus appears to be a causative factor for postoperative corneal melting. Damage to the limbal stem cells predisposes to invasion of conjunctival epithelium onto the corneal surface, which in turn leads to an irregular and unstable epithelium, with corneal ulceration. Since the cornea forms the anterior wall of the eye, loss of structural integrity leads to enlargement of the anterior chamber. This produces a characteristic appearance on CT, in which the distended anterior chamber is filled with material of a similar attenuation to the vitreous chamber (Fig. 2.6). In addition, there may be accompanying inflammatory or infectious changes that are apparent on imaging. Corneal melt can be treated using a full-thickness corneal button denuded of endothelium in conjunction with temporary tarsorrhaphy and systemic steroids.
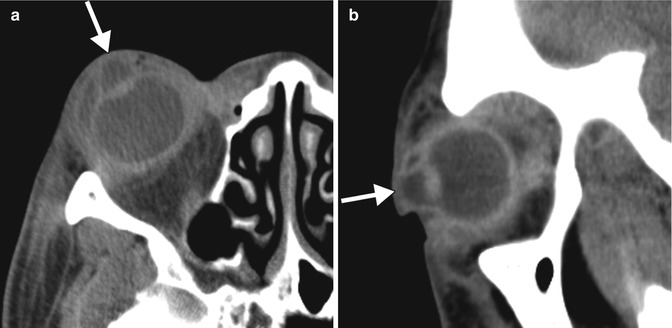
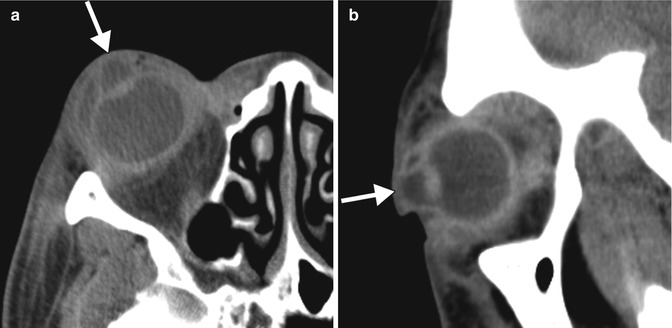
Fig. 2.6
Corneal melt. Axial (a) and sagittal (b) CT images show a considerably enlarged anterior chamber (arrows) of the right eye. There are diffuse preseptal and postseptal inflammatory changes related to concomitant panophthalmitis. The lens is in a normal position
Corneal transplant donors are routinely screened for the presence of various infectious diseases, such as HIV and hepatitis B and C. Nevertheless, there have been rare instances of prion transmission from corneal transplantation, resulting in Creutzfeldt–Jakob disease. Patients typically present with a rapidly progressive dementia. Brain MRI, particularly diffusion-weighted imaging, can be very helpful in the diagnosis of Creutzfeldt–Jakob disease, in which diffusion abnormalities involving the cortex and deep gray matter nuclei can be observed (Fig. 2.7).
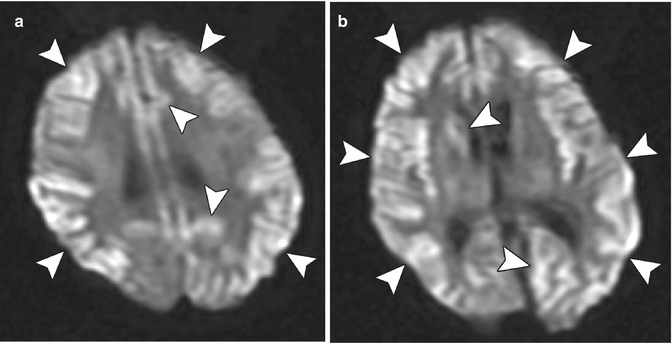
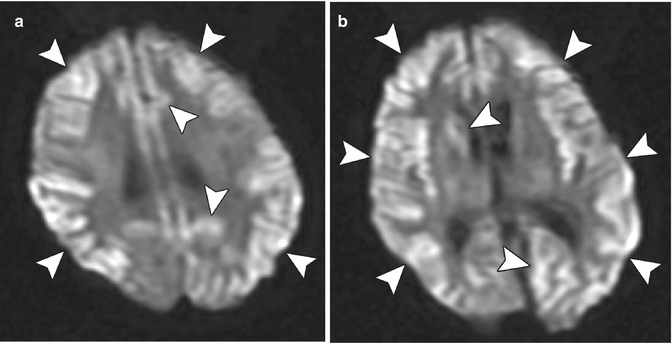
Fig. 2.7
Creutzfeldt–Jakob disease presumed to be associated with corneal transplantation. Axial diffusion-weighted images (a, b) of the brain demonstrate diffuse areas of restricted diffusion in the cortex and portions of the basal ganglia (arrowheads). The images are degraded by patient motion artifact
2.3 Lamellar Keratoplasty
Lamellar keratoplasty aims at replacing only the diseased corneal tissue while leaving the healthy tissue intact. The most common keratoplasty techniques in widespread use currently are deep anterior lamellar keratoplasty (DALK), Descemet’s stripping endothelial keratoplasty (DSEK) or Descemet’s stripping automated endothelial keratoplasty (DSAEK), and Descemet’s membrane–endothelial keratoplasty (DMEK). Each of these procedures entails replacement of different layers of the cornea (Fig. 2.8).
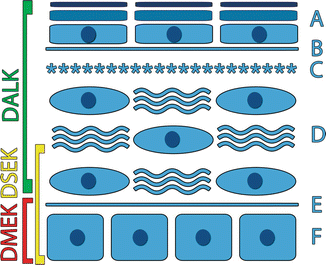
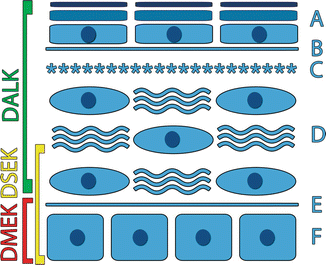
Fig. 2.8
Schematic of the corneal layers removed during the DLEK, DSEK, and DMEK procedures. A epithelium, B basement layer, C Bowman’s layer, D stroma, E Descemet’s layer, F endothelium
DALK replaces both the epithelium and most of the stroma with the donor tissue and is indicated when the corneal disease spares the posterior stroma, Descemet’s membrane, and endothelium (e.g., postinfectious stromal corneal scars, keratoconus). Since the host endothelium remains intact, the incidence of graft rejection is less with DALK than with penetrating keratoplasty. Smoothness of the stromal interface correlates with visual outcome.
DSEK/DSAEK aims at replacing a diseased endothelium (e.g., in pseudophakic bullous keratopathy or Fuchs’ endothelial dystrophy) while leaving most of the host stroma intact. Compared to full-thickness grafts, EK provides a better visual outcome by minimizing the postoperative astigmatism that is inherent to the tissue warpage, surface mismatches, and multiple corneal sutures in a penetrating keratoplasty, and it also ensures a globe that is tectonically stable to trauma and less prone to suture-related infections. Endothelial keratoplasty is a reliable surgical technique for Fuchs’ endothelial dystrophy and pseudophakic bullous keratopathy. The procedure consists of selective replacement of a patient’s diseased or dysfunctional endothelium with a graft of the posterior stroma and endothelium from a donor cornea. In DSAEK, the donor corneal dissection is changed from a manual approach to an automated, microkeratome-assisted procedure. Hence, the stromal interface is smoother and more uniform in shape in DSAEK as compared to DSEK.
DMEK is a more recent procedure that is indicated for patients with endothelial dysfunction. The procedure consists of stripping the host corneal Descemet’s membrane and endothelium and replacing it with harvested tissue from a healthy donor cornea (Descemet’s membrane–endothelial cell graft or lenticule). The thin Descemet’s membrane–endothelial cell graft measures only a few microns in thickness and is transplanted through a small (~3 mm) corneal incision, via a transfer device (such as a glass pipette) and then unrolled and adhered to proper location.
Anterior segment OCT may be considered the cross-sectional imaging modality of choice for depicting the layers of the cornea after anterior and posterior lamellar keratoplasty procedures. It is indicated in the follow-up of corneal deturgescence, in the evaluation of the donor disk and recipient stroma adhesion and apposition, and for the study of donor tissue thickness and its regularity, which may influence the final visual acuity and refractional changes after surgery. The grafts should be flush against the host tissue and the graft–host junction normally appears as a thin, smooth, hyperreflective line (Fig. 2.9). Furthermore, OCT is useful for evaluating postoperative complications, such as graft detachment, posterior lamellar dislocation, primary graft failure, and anterior chamber crowding with consequent chamber angle encroachment and pupillary block after Descemet’s stripping automated endothelial keratoplasty (DSAEK).
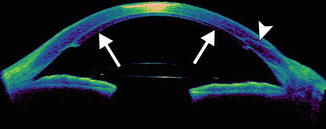
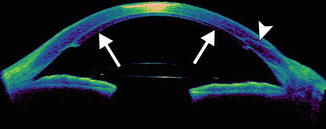
Fig. 2.9
DSEK. The anterior segment “rainbow” color OCT image shows the thin layer of donor posterior stromal tissue along with Descemet’s membrane and endothelium applied to the native host tissue (arrows). The graft–host interface appears as thin hyperreflective line. The clear corneal incision is also visible (arrowhead)
Graft detachment is the most common complication during the early postoperative period. Anterior segment OCT is very useful for diagnosing and delineating graft detachment, particularly when graft stromal edema obscures direct visualization (Fig. 2.10). Graft detachment can produce the appearance with “double anterior chamber formation” due to the hyporeflective space between the graft and the host cornea. Furthermore, anterior segment OCT can monitor Descemet’s detachments at precise topographic regions and allows appropriate clinical decisions to be made for surgical intervention, which may involve graft reshaping, repositioning, or rebubbling. Indeed, OCT is useful for evaluating the graft for potential factors that predispose to this complication, such as the presence of stromal tags (Fig. 2.11). Another notable, but uncommon, complication is interface epithelial ingrowth or downgrowth, which can undermine the results of endothelial keratoplasty by causing corneal clouding and potentially resulting in graft detachment. The sheet of proliferating epithelial cells deep in the corneal flap is clearly visible on anterior segment OCT as a highly reflective layer (Fig. 2.12). The use of shorter wavelength scanners in particular can delineate the lesion with great detail. However, penetration of the short wavelength light beyond the lesion is relatively limited.
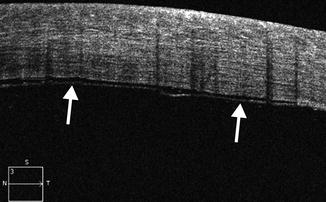

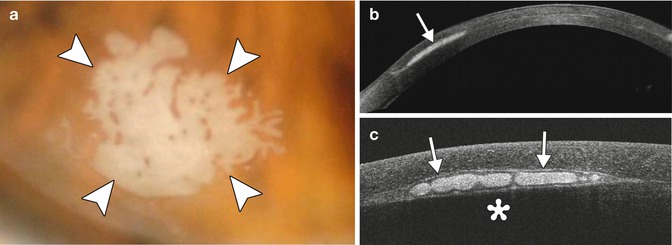
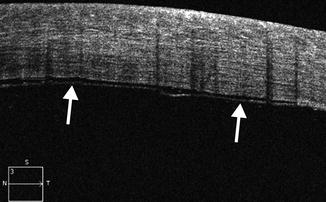
Fig. 2.10
Graft detachment following DMEK. Anterior segment OCT image shows the Descemet’s membrane–endothelial layer (arrows) is not fully adhered to the stromal tissue. There is a thin hyporeflective gap between the graft and the host cornea

Fig. 2.11
Corneal graft separation after DSEK/DSAEK with subsequent reattachment. The microkeratome stopped cutting just slightly inside the 9 mm corneal trephine used to punch the DSEK button. Anterior segment OCT (a) shows that there is a stromal tag that is left behind on the posteriorly displaced graft (arrow), resulting in a hyporeflective gap with the appearance of a “double anterior chamber” (arrowheads). The patient subsequently underwent explant of the corneal graft, which was trimmed using fine scissors and then reinserted. Anterior segment OCT obtained after the revision surgery (b) shows that there is a small air bubble trapped within the interface (arrow), which is otherwise markedly improved, and that the graft is slightly thinner where the stromal tag was previously located (arrowhead)
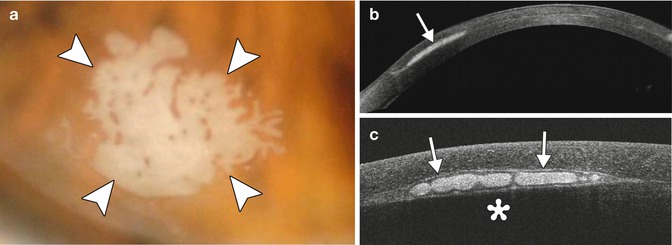
Fig. 2.12
Epithelial ingrowth after IntraLase-enabled DALK. The patient presented 19 months after surgery with a patch of epithelial ingrowth at the graft–host interface (arrowheads), as demonstrated on the clinical photograph (a). Anterior segment OCT image obtained using 1,310 nm wavelength light (b) shows the hyperreflective epithelial ingrowth (arrow) situated within the interface of the graft and the native cornea. Anterior segment OCT image obtained using 840 nm wavelength light (c) shows exquisite, higher-resolution detail of the epithelial ingrowth (arrows), but the corneal stroma posterior to the lesion is obscured due to the relatively weaker penetration of the shorter wavelength light
2.4 Keratoprosthesis
The success rate of penetrating keratoplasty is as high as 90 % after 1 year for patients with corneal scarring due to trauma, keratoconus, and corneal dystrophies and degenerations. However, corneal graft survival is poor in cases of autoimmune ocular surface disorders, such as Stevens–Johnson syndrome/toxic epidermal necrolysis and mucous membrane pemphigoid, after severe chemical burns and severe keratoconjunctivitis sicca, or in the presence of corneal vascularization from other causes. In such cases of complicated corneal blindness, the implantation of a keratoprosthesis may be the only option for visual rehabilitation.
The Boston keratoprosthesis (KPro), previously known as the Dohlman-Doane keratoprosthesis, is the most commonly used keratoprosthesis worldwide and comes in two forms. More than 10,000 of these devices have been implanted since approval for marketing by the US Food and Drug Administration in 1992. The type I device is a collar button-shaped device composed of a polymethyl methacrylate (PMMA) front plate and its stem and a PMMA or titanium back plate (Fig. 2.13). The type II device has an additional anterior nub that allows for through-the-lid implantation (Fig. 2.14). A corneal donor graft is sandwiched between the front and the back plates; the titanium back plate locks the device in place. The assembled keratoprosthesis and corneal donor graft are sutured in place in a fashion similar to standard penetrating keratoplasty.
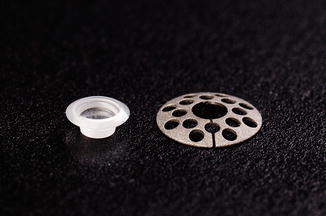
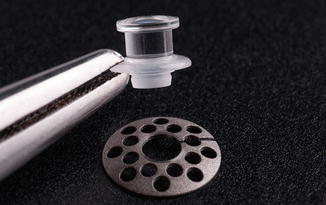
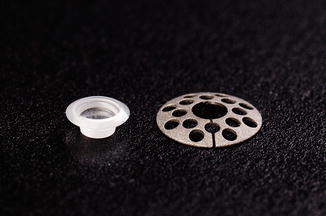
Fig. 2.13
Photomicrograph of FDA-approved version of the Boston keratoprosthesis type I device, prior to assembly
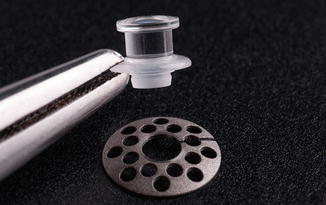
Fig. 2.14
Photomicrograph of FDA-approved version of the Boston keratoprosthesis type II device, prior to assembly
On CT, both the type 1 and 2 Boston keratoprostheses are discernible, in which the PMMA components appear as soft tissue attenuation alongside the higher attenuation titanium plates (Figs. 2.15 and 2.16). CT can also depict the surrounding orbital contents rather well, without significant artifacts from the keratoprostheses. On the other hand, the Boston keratoprostheses are MRI conditional, since the implants produce artifact. UBM can grossly depict the major components of the keratoprostheses and surrounding anatomy (Fig. 2.17). Fourier-domain anterior segment OCT is also a useful imaging technique in patients with a KPro and provides the ability to identify changes that are sometimes difficult to appreciate by clinical evaluation. The higher spatial resolution Fourier-domain systems may aid in the clinical diagnosis and management of pathology that might not be imaged with instruments of lower resolution. Anterior segment OCT has the potential for monitoring the anatomic stability of an implanted KPro and may also help to monitor for complications. Moreover, high spatial resolution imaging may enhance our understanding of periprosthetic anatomy.
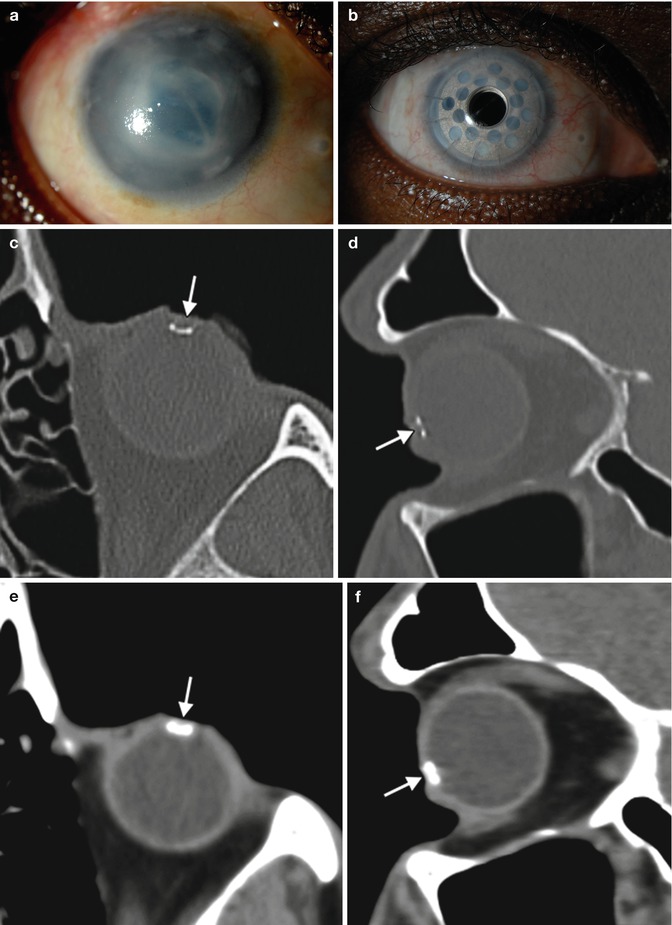
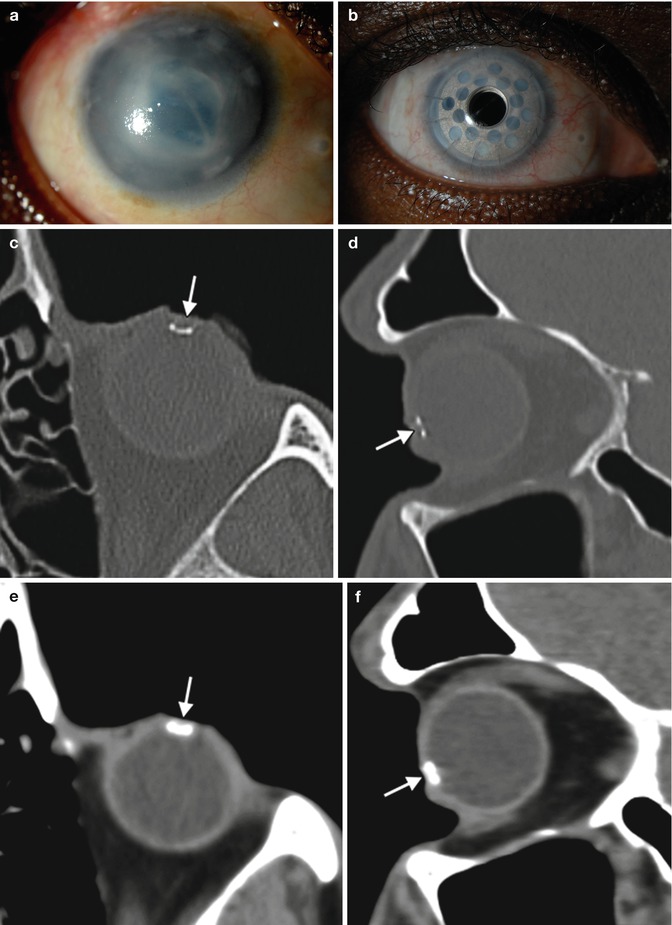
Fig. 2.15
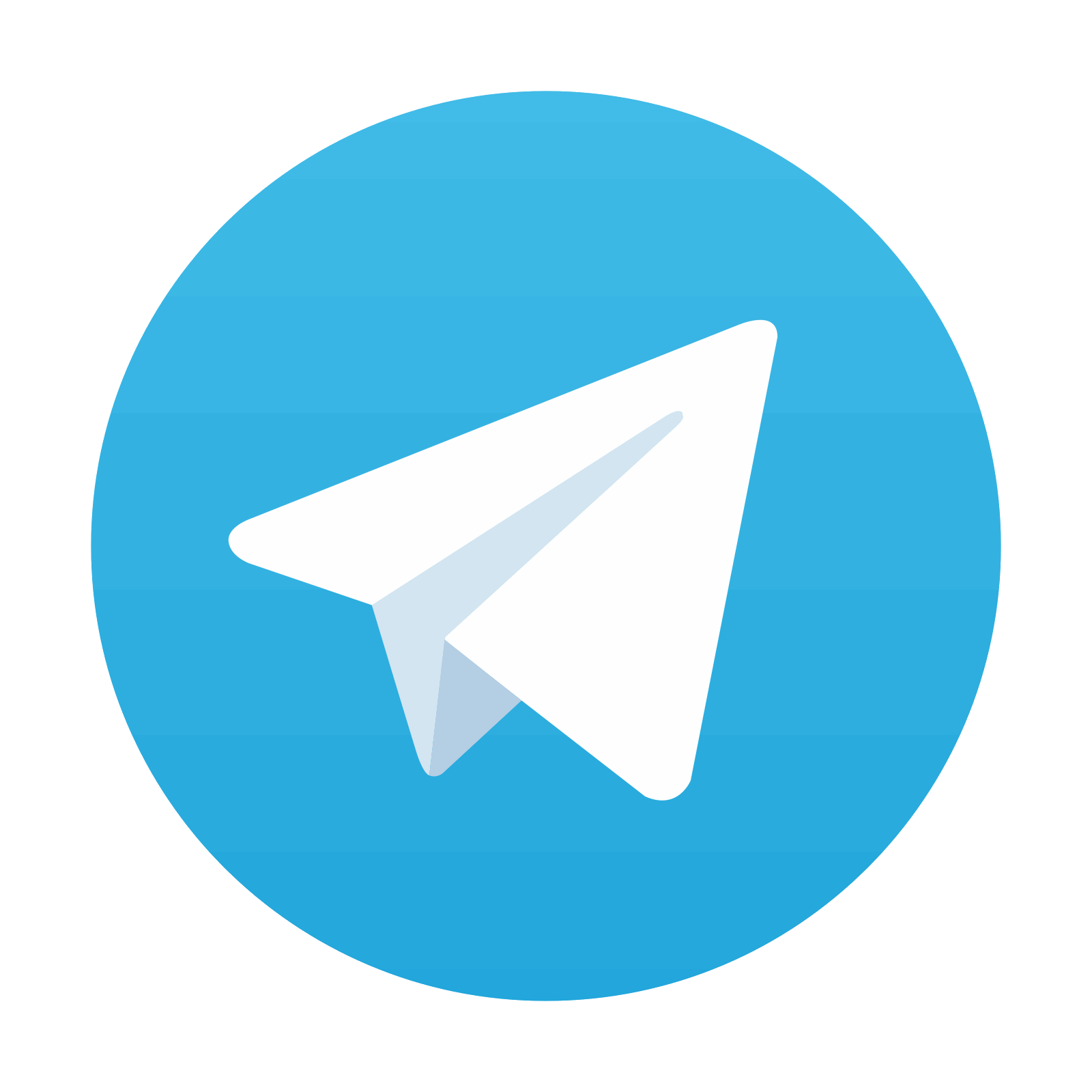
Boston keratoprosthesis type I. Preoperative (a) and 1-year postoperative (b) clinical photographs of the right eye of a patient with history of chronic uveitis, glaucoma, and corneal opacity. Axial and sagittal CT images (c–f) in bone windows and soft tissue show a titanium component (arrows) within the anterior chamber of the globe. The other portions of the prosthesis are not well delineated
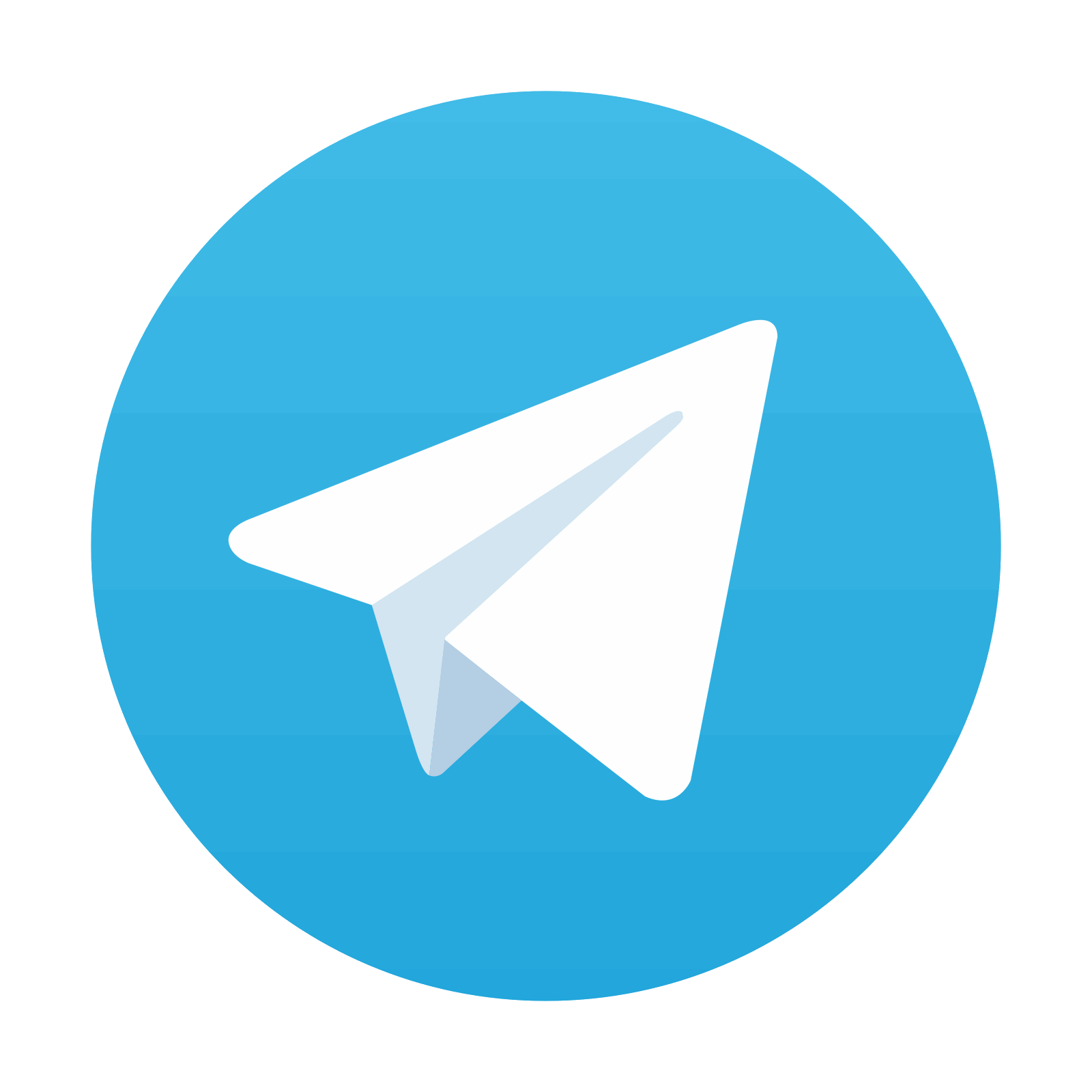
Stay updated, free articles. Join our Telegram channel

Full access? Get Clinical Tree
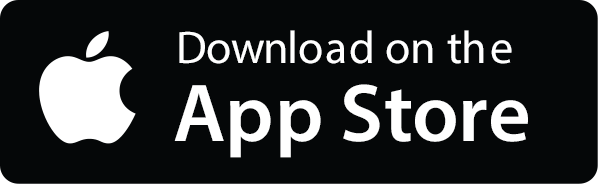
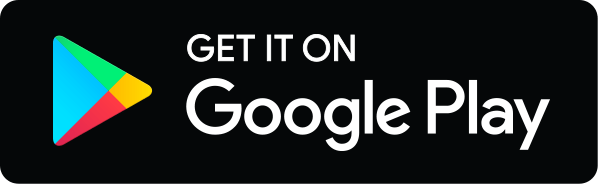
