Fig. 10.1
Normal hamstrings tendon origin on MRI and ultrasound. (a) Axial T1-weighted MRI demonstrates the normal hamstrings tendon origin. The semimembranosus origin (arrowhead) originates laterally from the ischial tuberosity whereas the conjoined tendon of the semitendinosus and biceps femoris originates medially (arrow). (b) Short-axis gray-scale ultrasound of the hamstrings tendon origin (arrows); image (right) is medial. (c) Photograph depicting transducer orientation for short-axis image of the hamstrings tendon origin; image (right) is medial. (d) Long-axis gray-scale ultrasound of the hamstrings tendon origin (arrows) from the ischial tuberosity (IT); image (left) is proximal. (e) Photograph depicting transducer orientation for long-axis image of the hamstrings tendon origin
Grade II muscle strains, also known as partial tears, are characterized by partial fiber disruption. Some fibers remain intact from the origin or insertion, but, particularly on the fluid-sensitive sequences, fiber waviness or partial-thickness retraction is apparent (Fig. 10.11). Fluid may track along the fascial interface with the injured muscle or myotendon, and this fluid may demonstrate variable degrees of heterogeneity depending on the presence of associated blood products and debris. An intramuscular hematoma is often seen at the myotendinous junction in grade II injuries (Peetrons 2002). The appearance on longitudinal plane ultrasound is particularly complementary to the blunted fibers seen on MRI, as one sees blunting of the typically linear fibrillar architecture of the muscular unit along its long axis (Fig. 10.12).
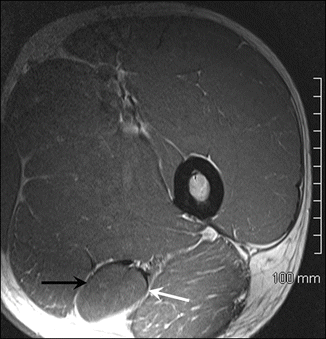
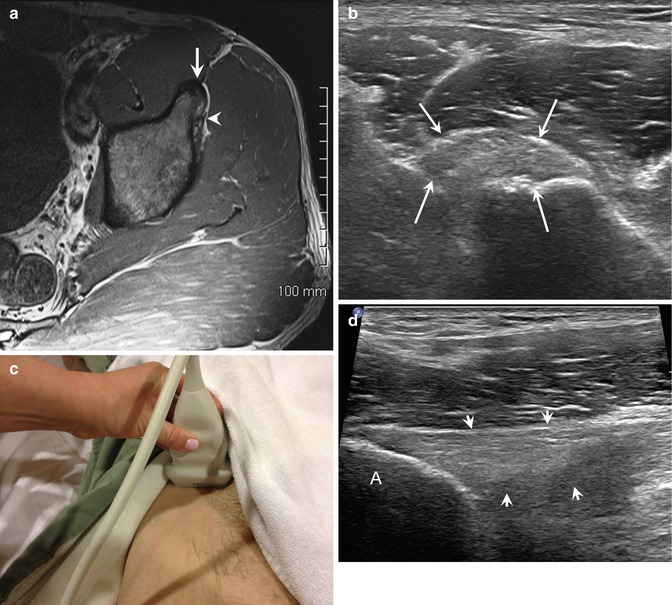
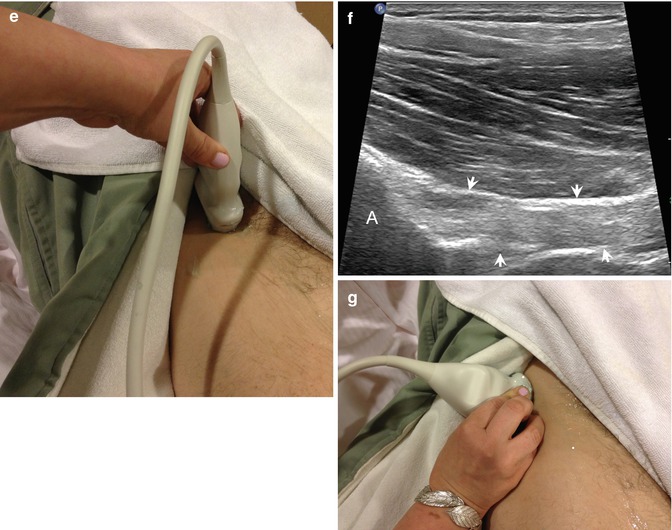
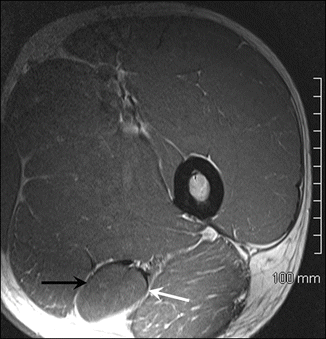
Fig. 10.2
Normal proximal hamstrings musculature on MRI. Axial T1-weighted MRI of normal proximal hamstrings musculotendinous junction region. The biceps femoris (white arrow) is now lateral; the semimembranosus is now medial (black arrow)
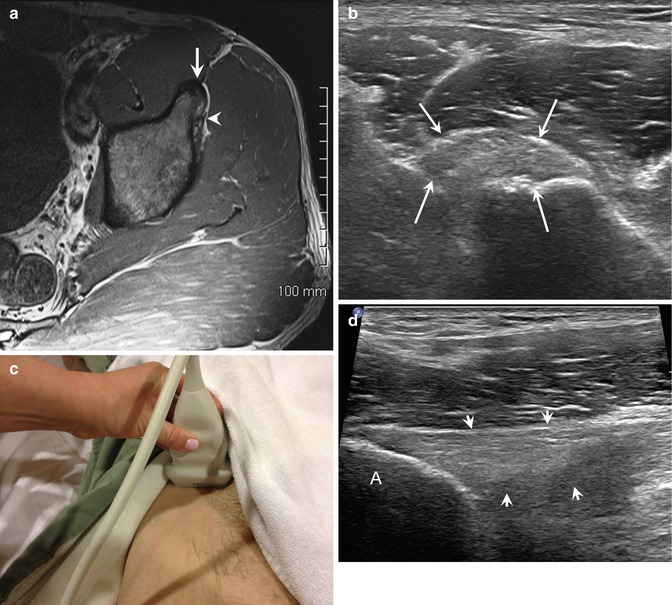
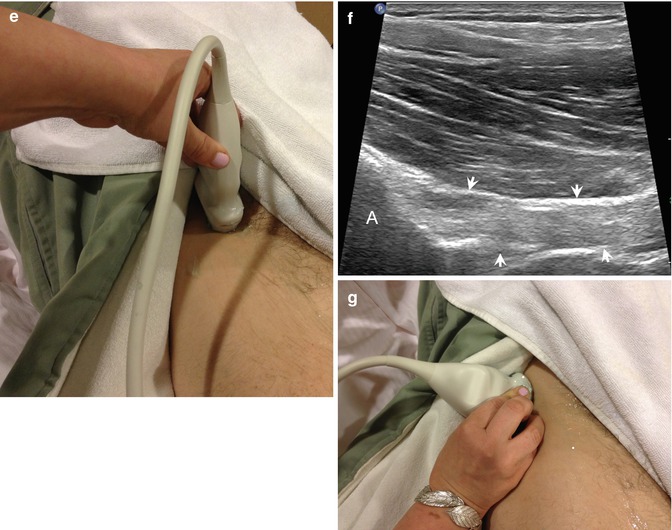
Fig. 10.3
Normal rectus femoris origin on MRI. (a) Axial T1-weighted MRI of normal rectus femoris origin, direct head (arrow) and indirect head (arrowhead). Normal rectus femoris direct head origin on ultrasound. (b) Short-axis image of the direct head origin (oval) and (c) corresponding photograph showing transducer orientation. (d) Long-axis image of the direct head origin (arrowheads) from the anterior inferior iliac spine (A) and (e) corresponding photograph. Normal rectus femoris indirect head origin on ultrasound. (f) Ultrasound of normal rectus femoris indirect head origin (arrowheads) from supra-acetabular ridge of ilium (A) and (g) corresponding transducer position nearly in the coronal imaging plane
The most striking feature of grade III injuries is the “fluid gap.” However, depending on the timing of the injury in relation to imaging, it may or may not be obvious. Grade III injuries are complete disruptions and are often surgical cases. As with grade II injuries, MRI and ultrasound findings are intuitively interchangeable for grade III injuries. Whereas on MRI the fluid gap may be homogeneously T2/PD hyperintense or demonstrate heterogeneity, on ultrasound, the fluid gap may be anechoic or, more commonly, of heterogeneous echogenicity due to a mixture of hemorrhage products (Fig. 10.13). For all grades of injury, the radiologist should remember that architectural distortion depicted as blunting of fibers, feathery fat, relative volume loss, and gross muscle or myotendinous retraction may be present in remote injury.
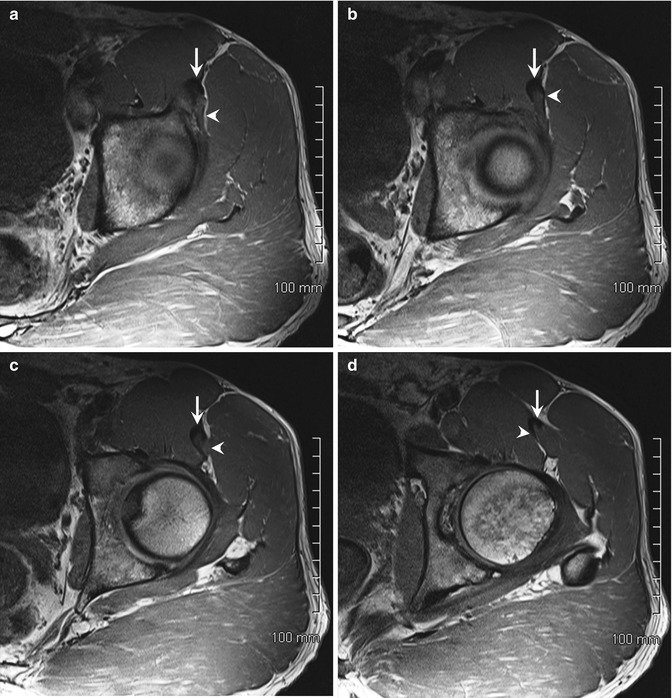
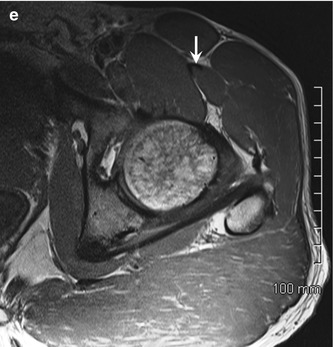
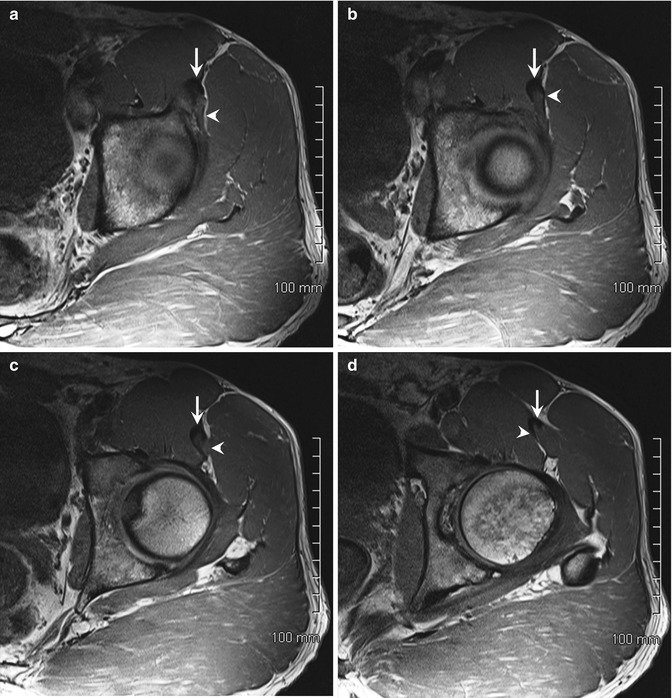
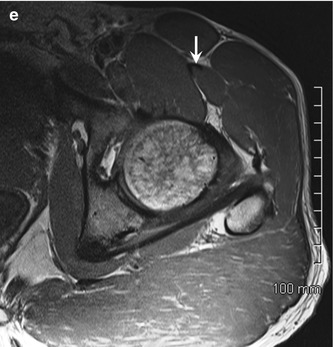
Fig. 10.4
Normal proximal rectus femoris on MRI. (a–e) Axial T1-weighted MRI of normal confluence of direct (arrow) and indirect (arrowhead) heads of rectus femoris, proximal to distal
10.2.5.2 Hamstring Injuries
Location within a body part is as critical to the radiologist as location within a town is to a real estate agent. Likewise, the location of injury within the hamstring muscle group is a key discriminating feature in soccer, as it can provide some degree of prognostic information as well as correlate with the type of injury. Recall from the earlier discussion the differentiation between “sprinting-type” and “stretching-type” injuries. These different mechanisms of injury usually result in different locations and gradations of strain in the hamstrings.
Of all hamstring injuries in soccer players, the biceps femoris is the most frequently affected muscle. Most of these injuries occur in a “sprinting-type” setting, which is similar to the hamstring injury seen in track and field athletes (Fig. 10.14). Biomechanical studies have shown the biceps femoris to be the hamstring muscle most prone to injury in sprinting, with injuries occurring in the terminal swing phase of the running gait cycle. This is due to a lengthening contraction occurring at this phase of the running cycle. Compared to the medial hamstring muscles, the biceps femoris experiences more stretch at this phase of the running cycle due to the smaller size of the knee flexion moment arm in the biceps femoris (Chumanov et al. 2012). In a study of top Danish soccer players with acute injuries, the biceps femoris was the affected muscle in 2 out of 3 hamstring injuries (Petersen et al. 2014). This is consistent with other previously published series (Connell et al. 2004). Of biceps femoris injuries, the majority are localized within the muscle belly or at the proximal myotendinous junction (Fig. 10.15a–c). Distal myotendinous junction injuries are very rare.
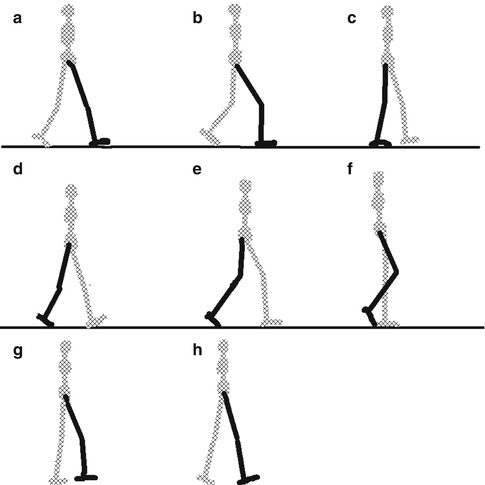
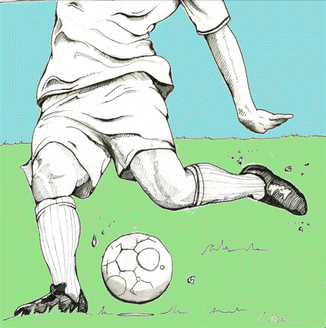
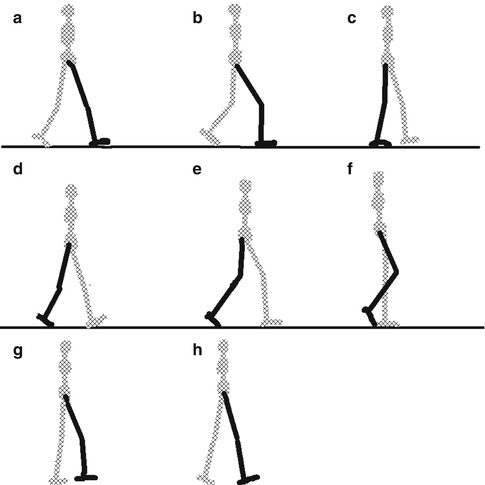
Fig. 10.5
Phases of the gait cycle (a initial contact, b loading response, c mid-stance, d terminal stance, e pre-swing, f initial swing, g mid-swing, h terminal swing)
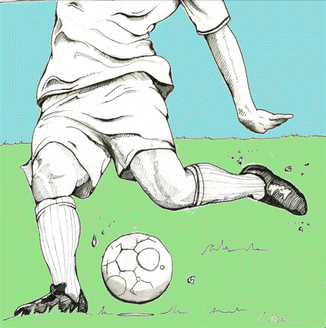
Fig. 10.6
Mechanism of rectus femoris injury while kicking in soccer. Soccer player in the act of an instep kick. Player’s left rectus femoris is susceptible to injury in the early part of the swing phase due to eccentric contraction as the leg begins to move forward. The right, planting limb rectus femoris is susceptible to injury at this time in the foot plant/ground contact phase of the kick due to a lengthening contraction as the body decelerates upon foot plant; the body continues to move forward relative to the plant foot, stretching the planting limb rectus femoris (Drawing courtesy of Craig Muderlak)
Although sprinting-type injuries and pathology localized to the biceps femoris are the most common type of hamstring injury in soccer players, they are not the only injury pattern seen in this population. As discussed previously, soccer requires players to perform a wide range of motions in addition to sprinting. In these scenarios, stretching-type injuries can occur. The hamstring injury pattern in this setting is more akin to that seen in dancers, who perform sagittal-split maneuvers and overhead leg extensions similar to the high kicking motion of soccer players. Mechanically, the position of the lower extremities is different in stretch-type injuries than in sprinting-type injuries. Instead, in this setting, the hip is flexed and the knee is fully extended. In this population and activity, studies have shown the injury to more commonly involve the semimembranosus. These injuries of the semimembranosus almost always involve the proximal free tendon (Askling et al. 2008) (Fig. 10.15d–e). Semimembranosus stretch injuries are associated with a longer recovery period than biceps femoris injuries. When injuries affect the semitendinosus, the pathology is frequently intramuscular.
In the soccer player, hamstring muscle and myotendinous injuries are overwhelmingly grade 0 or grade I in severity, with up to 70 % of injuries falling into this category in a large-scale trial of European professional players (Ekstrand et al. 2012). Full-thickness avulsions have been reported, but they are uncommon by comparison (Fig. 10.16). From the imaging perspective, accurate description of any injury is most important, rather than simply providing a numeric grade. Communication of findings can be improved by familiarity with other classification systems, such as those used by orthopedic surgical colleagues. For example, in the setting of proximal hamstring injuries, the important characteristics to evaluate for and communicate from the orthopedic surgeons’ perspective include osseous apophyseal avulsion in skeletally immature patients (type 1), musculotendinous avulsions (type 2), incomplete tendon avulsions from bone (type 3), complete avulsions from bone without retraction (type 4), and complete avulsions with retraction (type 5) (Wood et al. 2008) (Fig. 10.17).
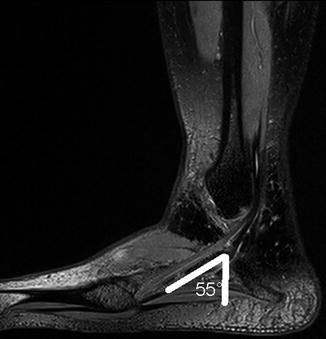
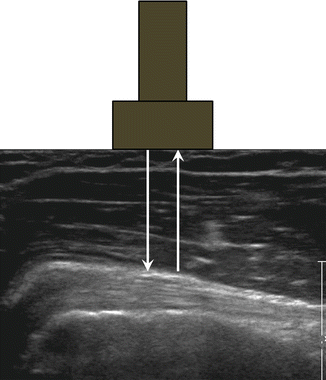
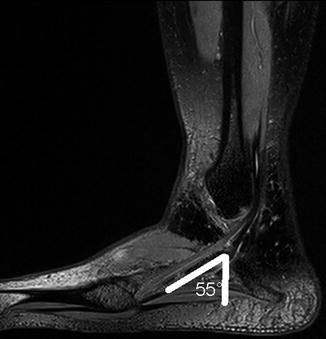
Fig. 10.7
Magic angle artifact. Sagittal 3D Cube fat-suppressed proton density-weighted MRI of the peroneal tendons showing increased signal of the inframalleolar peroneus brevis tendon due to magic angle artifact from the tendon being oriented 55° relative to the main magnetic field (B 0)
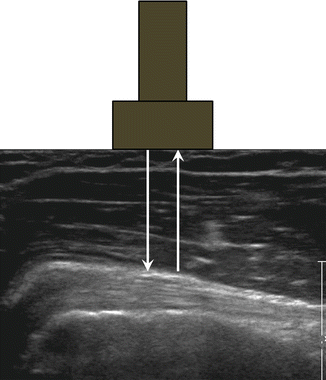
Fig. 10.8
Normal appearance of a tendon on ultrasound. In this longitudinal gray-scale image of the long head of biceps tendon, the tendon appears echogenic and fibrillar when the angle of incidence of the ultrasound beam and the axis of the tendon are oriented 90° relative to each other
MRI has been shown to have prognostic value regarding expected return to play, and the radiologist should take this into consideration when formulating a report. The most comprehensive scoring system for prognostic purposes at the present time exists for MRI and is based on hamstring injuries in professional American football players. In this scoring system, multiple factors are analyzed and include player age, number of muscles involved, location (proximal, middle, or distal; distal considered more severe), insertional location of injury, cross-sectional percentage of involvement, retraction, and long-axis length of involvement on T2-weighted fluid-sensitive sequences. Although it is unclear if this system can be directly extrapolated to soccer players, it is interesting to note that factors associated with longer time on the injured reserve included retraction, multi-site involvement, greater cross-sectional percentage of muscle involvement, and long-axis T2 signal abnormality greater than 10 cm (Cohen et al. 2011). The long-axis signal abnormality measurement has also been suggested as a predictive indicator of return to play in Australian Rules football players (Gibbs et al. 2004; Connell et al. 2004). While this information is interesting, studies of equal rigor have not been performed in soccer players; this information is worth noting but should not be applied directly without caveat.
10.2.5.3 Quadriceps Injuries
The rectus femoris is the most frequently injured of the 4 individual muscles comprising the quadriceps muscle group. Injuries can be subcategorized based on location and can be thought of in a proximal-to-distal approach: direct head origin, indirect head origin, peripheral proximal myotendinous junction, deep myotendinous junction of the indirect head, and the distal myotendinous junction closer to the knee joint. Injuries to the vastus musculature do occur but are much less common than those affecting the rectus femoris.
Of the rectus femoris injuries, the proximal deep myotendinous junction of the indirect head is the most common injury location among soccer players (Mendiguchia et al. 2013). For clarification purposes, the reader should be aware of synonymous terms used in the literature for injuries in this location, which include “central aponeurosis tears,” “central tendon tears,” and “strains about the central tendon.” Recall from the earlier discussion that the indirect head contributes to the long, deep intramuscular tendinous scaffold within the rectus femoris muscle. The direct and indirect head contributions are best depicted on axial and coronal MRI. Though fluid-sensitive sequences typically best show pathologic changes, T1-weighted images better depict anatomic detail. Following the individual tendons from their origin until the point at which they blend with their respective myotendinous junction on T1-weighted images allows the imager to appreciate these important anatomic relationships. In a study that reviewed MRI in 20 patients with deep myotendinous junction injuries, a so-called “bull’s-eye” pattern was the most frequently observed finding (Gyftopolous et al. 2008). The bull’s-eye pattern is characterized by a halo of increased signal around the darker deep tendon on sequential axial images and can be appreciated on T1, fluid-sensitive, or post-contrast sequences (Fig. 10.18). Most injuries in this series were grade II in severity. Other findings that may be seen in this type of injury include tendon thickening, partial distal retraction of muscle fibers, pseudocyst formation, hematoma, fatty-infiltration of muscle, and scar formation (Fig. 10.19). Grade I injuries are less frequently seen, presumably because the mild nature of the injury does not require imaging. Grade III injuries are even rarer, possibly due to the protective nature of the long deep myotendinous junction.
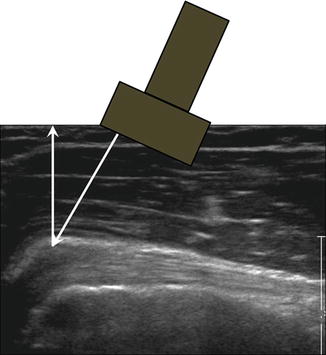
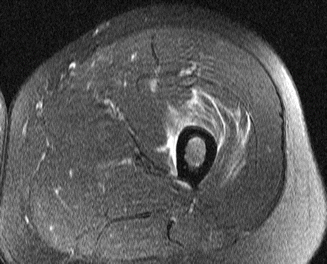
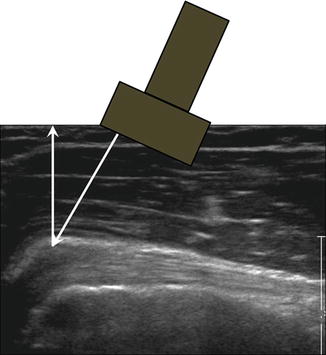
Fig. 10.9
Anisotropy of a normal tendon on ultrasound. In the same longitudinal gray-scale image of the long head of biceps tendon as in Fig. 10.8, the principle of anisotropy can be observed in the portion of the tendon coursing into the glenohumeral joint, turning away from the ultrasound beam and creating an angle between the incidence beam and the reflection beam different than 90°. The tendon appears artifactually hypoechoic in this region as a result
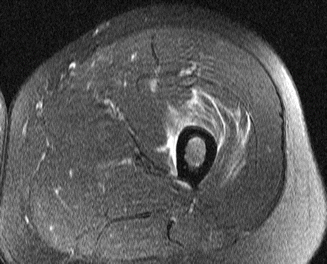
Fig. 10.10
Grade 1 muscle strain. Axial fat-suppressed T2-weighted MRI of the left thigh showing increased “feathery” signal centered in the vastus intermedius and vastus lateralis secondary to low-grade muscle strain
Deep proximal myotendinous junction injuries are noteworthy because they are associated with longer healing time than their peripheral counterparts. This phenomenon is reportedly related to a shearing effect that may occur with central tendon injuries as a consequence of the direct and indirect heads of the rectus femoris subsequently functioning independently from one another. This does not seem to happen with peripheral injuries. Importantly, 2 relevant imaging studies from within the past 10 years that involved kicking athletes with rectus femoris injuries evaluated the prognostic ability of ultrasound and MRI with respect to return to play and discriminated among different locations of rectus femoris injuries. These studies confirmed that central/indirect tendon injuries are associated with longer healing times. Specifically, the major ultrasound study prospectively evaluated rectus femoris injuries in 35 players from highly competitive Spanish soccer teams, characterizing the proximal/distal level of the injury to the central deep myotendon as well as the craniocaudal length of the ultrasound abnormality (Balius et al. 2009). The amount of time the players spent on the injured reserve was tracked. The proximal/distal designation in this study was binary; a “proximal” label was given if the ultrasound abnormality was located cranial to the intersection point of the lateral edge of the sartorius muscle and the medial edge of the rectus femoris, whereas “distal” lesions were caudal to this intersection. Unlike MRI, ultrasound cannot assess the “cross-sectional area percentage involvement” of the abnormality. Therefore, this was not recorded. In this study, proximal injuries were associated with a statistically significant longer amount of time on the injured reserve compared to distal injuries, averaging 45.1 days for the former and 32.9 days for the latter. Perhaps somewhat intuitively, the study also confirmed that the longer the amount of craniocaudal involvement and the higher the grade of injury, the longer the time to return to play. The main MRI prognostic study followed Australian rules football players with rectus femoris injuries over 3 years. A caveat worth noting is that the demands on Australian rules players and soccer players are somewhat different, with different sized fields, quantities of kicking per competition, and styles of kicking. Nevertheless, this study showed that, like soccer players with ultrasound-confirmed central tendon injuries, Australian rules players with MRI-confirmed central tendon injuries likewise experienced longer rehabilitation periods than those with peripheral rectus femoris injuries. In addition, players who sustained injuries to quadriceps muscles other than the rectus femoris had the shortest rehabilitation times, further supporting the claim that the rectus femoris is the most important of the quadriceps muscles from an injury perspective.
Rectus femoris myotendinous junction injury distally near the knee joint is the classically described location, with its associated proximal retraction of muscle producing the so-called pseudomass. However, it is much less common among soccer players. Nevertheless, its imaging appearance is characteristic, and an example is provided in Fig. 10.20.
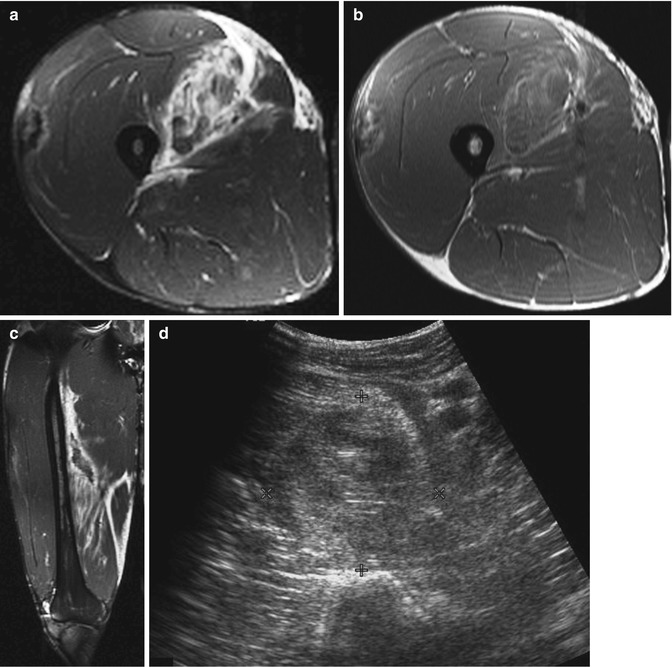
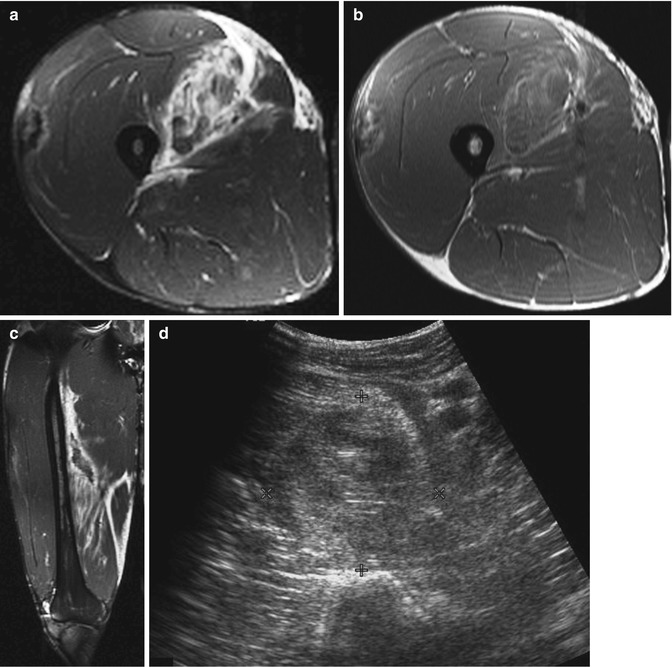
Fig. 10.11
Grade 2 muscle strain on MRI with ultrasound correlation. (a) Axial fat-suppressed T2-weighted MRI of the right thigh demonstrates fibrillar waviness, edema, and heterogeneous signal centered in the vastus medialis compatible with macroscopic partial muscle fiber disruption, retraction, and hematoma. (b) Axial T1-weighted MRI of the right thigh demonstrates T1 hyperintense intramuscular and intermuscular signal within and about the vastus medialis, compatible with hemorrhage products. (c) Coronal fat-suppressed T2-weighted MRI of the right thigh demonstrates fibrillar waviness and retraction, as well as intermuscular fascial edema. (d) Short-axis ultrasound image over the area of pain corresponding to the abnormalities described in (a–c) reveals a heterogeneous collection of mixed echo-rich and echo-poor material within the vastus medialis, compatible with a hematoma
Though less common, rectus femoris origin injuries are an interesting subset because of the dual-origin anatomy relationship in conjunction with the close association to the hip capsule. One of the more extensive retrospective review analyses showed that when the rectus femoris origin is injured, the injury sequence tends to follow a pattern. The first structure to tear is the indirect head origin, followed by the direct head origin (Fig. 10.21). In the most severe case, the conjoined tendon is involved (Ouellette et al. 2006). Retrospective analysis has shown a possible correlation between avulsions of the tendon origins and labral tears or capsular avulsions in the adolescent and pediatric soccer population (Foote et al. 2013). These injuries may occur together in this population because of concurrent traction forces placed upon the hip capsule, labrum, and rectus femoris origins in kicking and sprinting activities. As such, patients with persistent pain after an initial diagnosis of tendon avulsion (usually a nonoperative injury) may benefit from further evaluation of an intra-articular pathology to explain the ongoing pain.
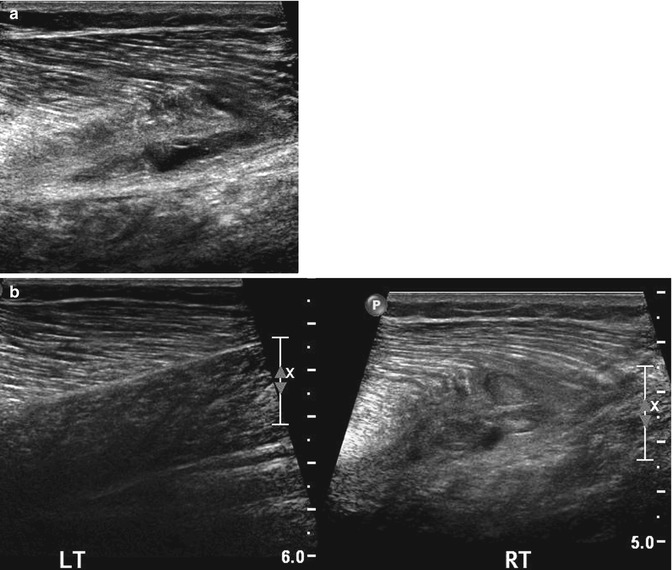
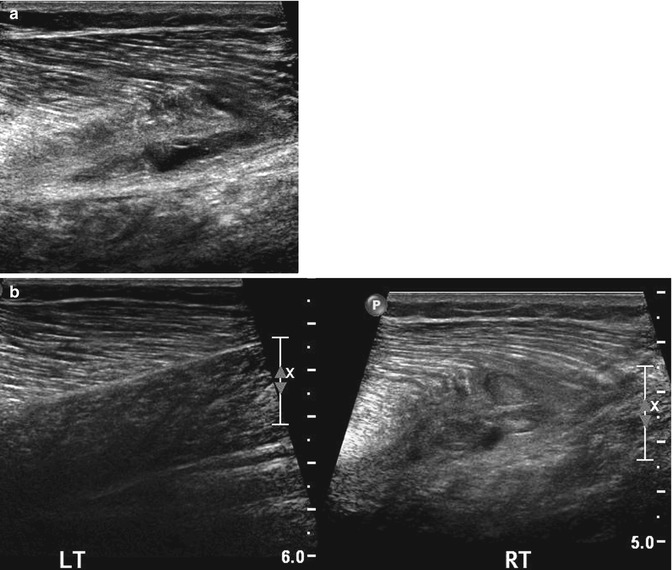
Fig. 10.12
Ultrasound of grade 2 muscle strain. (a, b) Long-axis ultrasound image of the right medial gastrocnemius in a soccer player who felt a pop while sprinting shows blunting of fibers and retraction from the long aponeurotic attachment. The associated nodular, echogenic structures are compatible with hematoma, a characteristic of grade II muscle injuries. The normal contralateral extremity was imaged for comparison
10.3 Ankle
10.3.1 Introduction
The ankle, with its wide range of motion, enables the soccer player to perform a multitude of tasks integral to the game. The capability of the ankle to undergo supination, pronation, dorsiflexion, plantarflexion, and circumduction allows the player to dribble, possess, and kick the ball, while it also acts as the conduit of energy transfer between the foot and leg/thigh for sprinting and jumping. However, the ankle’s important role as a lynchpin of the lower extremity is often jeopardized by the ankle’s susceptibility to injury due to the high-energy nature of the game.
Injuries to the ankle are fairly common in soccer, accounting for between 7 and 41 % of all injuries depending on the population sampled (Wong and Hong 2005; Ekstrand et al. 2011b). The vast majority of ankle injuries in soccer are ligamentous sprains, totaling up to 72 % of cases. Of these, 80–90 % involve the lateral ligamentous complex (anterior talofibular ligament, calcaneofibular ligament, and posterior talofibular ligament) (Kofotolis et al. 2007). Ankle sprains are more common in men than women and more frequently occur in the dominant leg than the non-dominant leg (Wong and Hong 2005). At the professional level, ankle injuries are much more common during match play than in practice, though amateurs have less variation of incidence between match versus practice setting. Ankle injuries are somewhat less common in professional play nowadays than they were 20–30 years ago, likely attributable to multiple factors, among them modifications in the intensity of training and improvements in the quality of rehabilitation and injury-prevention practices. Foul play still accounts for up to 40 % of all ankle sprains (Walden et al. 2013). Players with a history of prior ankle sprain are subject to a threefold increased risk for recurrent injury (Kofotolis et al. 2007; Walden et al. 2013). Inversion ankle sprains can be notoriously nagging from a symptoms perspective, with up to 74 % experiencing persistent pain, swelling, weakness, or instability in a study of soccer players and other athletes in Australia (Anandacoomarasamy and Barnsley 2005). Ankle osteoarthritis is an established potential long-term sequela of playing soccer at an elite level and is even more prevalent among retired soccer players who experienced an ankle sprain. A study of the Danish national team found osteoarthritis in 33 % of the players with prior ankle injuries, but of those without prior ankle injuries, only 18 % had osteoarthritis (Larsen et al. 1999). In part, the clinical diagnosis of “lateral ankle sprain” or “chronic lateral ankle instability” may be associated with prolonged recovery, persistent symptoms, or subsequent osteoarthritis in the soccer player because, in fact, many cases are accompanied by additional injuries which do not infrequently escape clinical detection. For example, a review of 61 patients with chronic lateral ankle instability in the orthopedic surgical literature found 15 different associated injuries at surgery, chief among them peroneal tenosynovitis, anterolateral impingement lesions, peroneal retinaculum attenuation, and ankle synovitis but also talar osteochondral injuries (DiGiovanni et al. 2000). Aside from ligamentous sprains, soccer players may experience anterior or posterior ankle impingement, the so-called “footballer’s ankle.” Though these entities are much less frequently cited in the literature of soccer epidemiology as maladies resulting in time on the injured reserve, they are repetitive overuse injuries that are largely confined to soccer players and have garnered attention in the orthopedic journals.
10.3.2 Relevant Ligamentous Anatomy
The lateral ligamentous complex is of primary importance in soccer ankle injuries. It is comprised of 3 individual ligaments: the anterior talofibular ligament, the calcaneofibular ligament, and the posterior talofibular ligament. Each normal ligament is thin, uninterrupted between named osseous structures, low in signal on all sequences, and contrasted by higher signal intensity fat on non-fat-suppressed images. The normal ultrasound appearance is characterized by an echogenic fibrillar, compact band of tissue. The anterior talofibular ligament (ATFL) extends between the anterolateral tip of the lateral malleolus and the talar neck and is best appreciated on a single axial image just distal to the tibiofibular ligament level (Rosenberg et al. 2000; Perrich et al. 2009). A helpful osseous landmark is the C-shaped configuration of the fibula at this level (Levine et al. 2010). The ATFL is found on ultrasound by angling the probe anteriorly from the distal tip of the lateral malleolus toward the talar neck (Fig. 10.22). The calcaneofibular ligament courses from the inferior tip of the lateral malleolus proximally to the trochlear eminence of the calcaneus distally, situated deep to the peroneal tendons. Typically, complete visualization requires several contiguous images in both axial and coronal imaging planes. On ultrasound, placing one end of the probe on the posterolateral calcaneus and the other end on the inferior tip of the lateral malleolus reveals the calcaneofibular ligament in long axis, deep to the peroneal tendons. The posterior talofibular ligament fans out from the lateral tubercle of the posterior process of the talus to the malleolar fossa of the fibula. It is easiest to appreciate on axial images and is the only one of the 3 lateral ligaments to show a striated appearance, secondary to a normal mixture of intervening fat and fibrous tissue (Perrich et al. 2009).
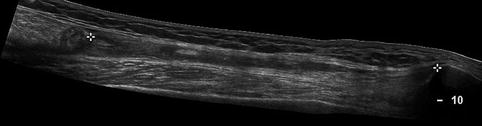
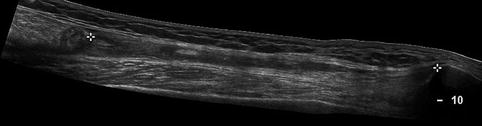
Fig. 10.13
Grade 3 muscle injury. Longitudinal ultrasound image of the quadriceps musculature obtained with panoramic function enabled, depicting a long tendon-bone gap (asterisks) between the retracted rectus femoris and the patella due to a full-thickness tear
10.3.3 Relevant Osseous Anatomy
The talar body is the proximal portion of the talus forming the ball component of the tibiotalar ball-and-socket joint. Arising from the posterior surface of the talar body, the posterior process of the talus is made up of medial and lateral talar tubercles that form a groove for the flexor hallucis longus. The lateral talar process originates as an accessory ossicle called the os trigonum. Usually, it appears between 7 and 13 years of age and fuses with the talus (Karasick and Schweitzer 1996). Sometimes, the lateral talar tubercle is asymmetrically elongated or prominent, in which case it is called a Stieda process (Hess 2011). The lateral and medial surface of the talar body articulate with the lateral and medial malleolus, respectively. Moving distally, the talar neck is found, with its superior surface containing an osseous prominence, the talar ridge, which serves as the attachment site of the talonavicular joint capsule. The lateral surface of the talar neck provides the attachment site of the anterior talofibular ligament. The most distal part of the talus, the talar head, articulates with the navicular bone and the calcaneus. The anterior subtalar joint is a composite of the anterior and middle talar facet articulations with the anterior and middle facets of the calcaneus, as well as the talonavicular articulation. The posterior subtalar joint is formed by the posterior facets of the talus and calcaneus.
10.3.4 Relevant Tendon Anatomy
The peroneus longus and brevis constitute the lateral ankle tendons and are located in the retromalleolar groove of the fibula. Their relationship can be recalled from the acronym “PL” for the peroneus longus as being relatively posterior and lateral to the peroneus brevis. They are enclosed by a common peroneal tendon sheath beginning 4 cm proximal to the inferior tip of the lateral malleolus and dividing into proper sheaths at the level of the calcaneocuboid joint (Tjin et al. 1997). A reasonable rule of thumb for differentiating physiologic fluid from pathologic fluid is that, in general, there should be less fluid than tendon in the sheath (Levine et al. 2010). The peroneal tendons are maintained within the fibular groove by the superior peroneal retinaculum, which arises from the lateral fibular periosteum, swings inferomedially around the tendons, and has 1 of 2 insertions: along the lateral border of the calcaneus or blending with the Achilles tendon aponeurosis (Rosenberg et al. 2003). On their caudal course, the tendons are separated by an important osseous landmark along the lateral aspect of the calcaneal body called the peroneal tubercle. The retrotrochlear eminence is situated posterior to the peroneal tubercle. This relationship can be remembered by understanding that the peroneal tubercle is also called the peroneal trochlea; therefore the retrotrochlear eminence must be posterior. The peroneus brevis extends to the base of the fifth metatarsal, while the peroneus longus courses over or encompasses the os peroneum, its sesamoid bone, before crossing the plantar aspect of the foot to insert on the medial cuneiform and base of the first metatarsal (Tjin et al. 1997).
10.3.5 Mechanism of Injury
A variety of scenarios expose the ankle to injury in soccer, whether from a slide tackle, upright player-to-player challenge, contested shot or clearance, awkward fall from jumping, or lost footing. However, the vast majority of injuries can be broken down into 1 of 2 main mechanistic categories. In the first, the inversion landing of the foot occurs as a consequence of a laterally directed force, most often due to opponent contact on the medial side of the leg. The second type occurs in forced plantar flexion when the player’s foot contacts the opponent while attempting to strike the ball with the instep, a kicking technique whereby the dorsal aspect of the foot strikes the ball while the foot is in plantarflexion. The latter may be acute but is more frequently a repetitive overuse injury. The 2 resulting injuries, lateral ankle sprain and “footballer’s ankle,” have long been known, but a relatively recent study specifically delineated these basic mechanisms of injury in soccer through analysis of 46 acute injuries in 313 videotaped matches compiled from 2 seasons in the elite Norwegian and Icelandic leagues (Andersen et al. 2004).
The lateral ankle inversion sprain has several common features, regardless of the specific subject-opponent action at the time of injury. In all cases from the abovementioned study, at least a part of the injured foot was in contact with the ground at the time of injury. In most cases, the exact moment of injury could be pinpointed to when the injured player transferred body weight to the injured ankle. Usually, the laterally directed force imparted to the subject by the opponent did not directly result in the injury; instead, the force caused the subject to land awkwardly, with the typical inversion event ensuing and the subject’s own bodyweight tensing the lateral ligamentous complex (Fig. 10.23). The UEFA Champions League injury study, the largest prospective soccer injury analysis, showed relatively less disparity between noncontact lateral ankle sprains and those resulting from player-to-player contact, 42 % in the noncontact group versus 58 % in the contact group (Walden et al. 2013). In both settings, as in most inversion ankle injuries, the first ligament under stress is the anterior talofibular ligament (ATFL). Once the ATFL experiences mechanical failure, the calcaneofibular ligament, followed by the posterior talofibular ligament, is stressed.
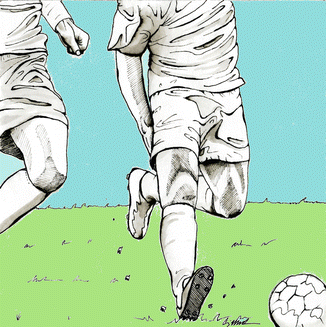
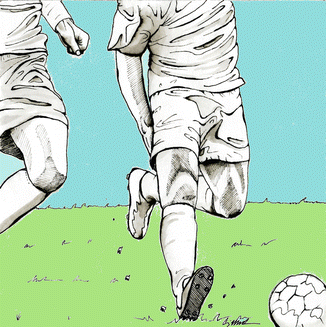
Fig. 10.14
Mechanism of hamstrings tendon injury while sprinting in soccer. Soccer player sprinting after the ball feels a sudden jolt of pain while in the terminal swing phase of gait just before the affected extremity makes ground contact. The biceps femoris is most prone to injury in this phase of sprinting because it experiences a lengthening contraction in terminal swing. Also, the muscle is biarticular, subjecting it to different stresses than a monoarticular muscle (Drawing courtesy of Craig Muderlak)
The exact mechanism of “footballer’s ankle” has been the subject of debate in the sports injury and biomechanics literature since it was first described over 70 years ago by Morris (Morris 1943). For clarification, “footballer’s ankle” has, in most instances, historically been considered anterior ankle impingement secondary to the repetitive actions that the ankle undergoes in the act of kicking. That impingement is a kicking-related phenomenon is supported by the fact that it is seen in the dominant leg two-thirds of the time when it is anterior (Walden et al. 2013). Three main theories of anterior impingement in soccer players have been at the forefront. In one theory, repeated kicking results in traction to the anterior joint capsule. Resulting enthesophytes predispose to the anterior impingement. In another theory, the bony proliferation along the anterior tibiotalar joint is secondary to multiple contact events between the ball and the anterior cartilage when the player kicks. Resulting inflammation turns into scar tissue, which eventually calcifies. In the third theory, the tibia and talus repeatedly impact against one another in the act of ankle dorsiflexion, resulting in microfractures and anterior exostoses (Andersen et al. 2004). An anatomic-imaging correlation study by Hayeri et al. sheds some light on the debate. According to their study, rather than being a “this or that” scenario, anterior ankle impingement, and perhaps then footballer’s ankle, could be due to both osteophytes and enthesophytes (Hayeri et al. 2009). The argument for enthesophytes stems from the coincidence of bony proliferations along the lateral margin of the talus at the same location as the insertion of the lateral aspect of the joint capsule and associated dorsal ligamentous structures. However, the medial talar neck bony proliferations were felt to represent osteophytes, as they occurred well within the joint and not at the site of capsular reflection. As Hayeri et al. pointed out, the dorsomedial foot has been shown to be the epicenter of foot contact with the ball in soccer players. As such, they concluded that the microtrauma-bony outgrowth cascade may be at play in this particular microenvironment. A caveat to these revelations, however, is that the study assessed cadavers and isolated talar specimens from a museum, in which past history of playing soccer, or even more generally, athletic involvement, was unknown. Additionally, the corresponding tibia was not available with the talar paleopathologic specimens.
Beyond the etiology debate regarding an anteriorly impinging footballer’s ankle, there is also some conflicting evidence as to which kind of impingement actually constitutes footballer’s ankle. In subgroup analysis of the UEFA Champions League study, there was an over threefold increased incidence of posterior impingement compared to anterior impingement. Like anterior impingement in soccer players, posterior impingement is likewise thought to be kicking-related, as more than half of posterior impingement cases involved the dominant leg (Walden et al. 2013). Posterior impingement is diagnosed clinically when there is chronic posterior ankle pain, generally related to activities that involve repeated plantar flexion of the ankle. There may be associated posterior ankle swelling. Any of 4 normal variant osseous structures may predispose a player to posterior impingement symptoms: a Stieda process, an enlarged os trigonum, a prominent posterior calcaneal tuberosity, and a downward-sloping posterior tibia. Of these, the Stieda process is most common and the os trigonum is the second most common (Hess 2011). Usually, patients present once a new associated soft tissue abnormality develops. For example, thickening of the posterior talofibular ligament, posterior intermalleolar ligament and/or posterior joint capsule, or flexor hallucis longus tenosynovitis are commonly identified soft tissue changes (Donovan and Rosenberg 2010). Furthermore, posterior ankle impingement and lateral ankle sprains may be related, with some players with posterior impingement symptoms reporting a relatively minor precipitating lateral sprain (Robinson and White 2002).
10.3.6 Imaging
Usually cross-sectional diagnostic imaging is not required in the workup of lateral ankle pain in the soccer player with an acute ankle sprain, as the injury mechanism and clinical signs and symptoms dictate management. Nevertheless, a review of the typical imaging workup is useful for understanding the role that imaging sometimes plays in the management of the injured ankle. The Ottawa ankle rules were initially described in 1992 and provide a guideline for clinicians to determine when it would be appropriate to screen adult patients with an acutely injured ankle using radiography (Stiell et al. 1994). The rules divide the foot and ankle into “malleolar” and “midfoot” zones. If the patient has pain in either zone and meets any one of specified zonal criteria, then radiographic assessment of the ankle or foot is indicated. These criteria include point tenderness to palpation over the medial malleolus, lateral malleolus, navicular or fifth metatarsal base, and inability to bear weight both immediately and in the emergency department. Inability to bear weight is defined as being unable to take 2 steps with each extremity without assistance. The implementing study found that the rules resulted in a 28 % relative reduction in ankle radiography and 14 % reduction in foot radiography at the study institution compared to the control institution. Furthermore, the rules were shown at that time to be 100 % sensitive for detection of malleolar and midfoot fractures. A subsequent systematic review analysis of 27 studies sought to assess the accuracy of the rules roughly 10 years after they were introduced and confirmed the high specificity of the rules and their projected reduction of inappropriate use of radiography by 30–40 % (Bachmann et al. 2003).
Beyond radiography, the soccer player with ankle pain following a lateral sprain may undergo further evaluation with cross-sectional imaging, usually MRI or ultrasound. Acutely, imaging of lateral ankle ligament sprains applies as it does elsewhere in the body. Edema about the ligament on fluid-sensitive MRI sequences with overlying soft tissue swelling and edema typifies the lower-grade injuries. Edema on ultrasound is manifest as hypoechoic reticulation of the soft tissues immediately surrounding the injured ligament. In moderate-grade sprains, the ligament is thickened and may demonstrate increased signal on fluid-sensitive MRI sequences (Perrich et al. 2009). On ultrasound, the ligament is thickened and hypoechoic. Edema around the ligament is the crucial diagnostic feature for acute injuries, as ligaments in remote injuries may also be thickened as in the acute setting, but they are not associated with edema (Levine et al. 2010). In cases of complete rupture, avulsions with or without associated osseous fragments may be seen. Alternatively, absence of definable fibers in the presence of edema may also indicate complete ligament failure. Anterior talofibular ligament injuries are readily seen on axial MRI, with a spectrum of findings ranging from normal to completely absent. The anterior talofibular ligament is depicted on ultrasound along its long axis by anchoring one end of the probe to the anterior aspect of the inferior tip of the lateral malleolus and then caudally deviating the other end of the probe toward the talar neck attachment (Fig. 10.24). In addition to routine signs of injury for the calcaneofibular ligament, a secondary sign of calcaneofibular ligament injury is the presence of fluid in the peroneal tendon sheath (Rosenberg et al. 2000).
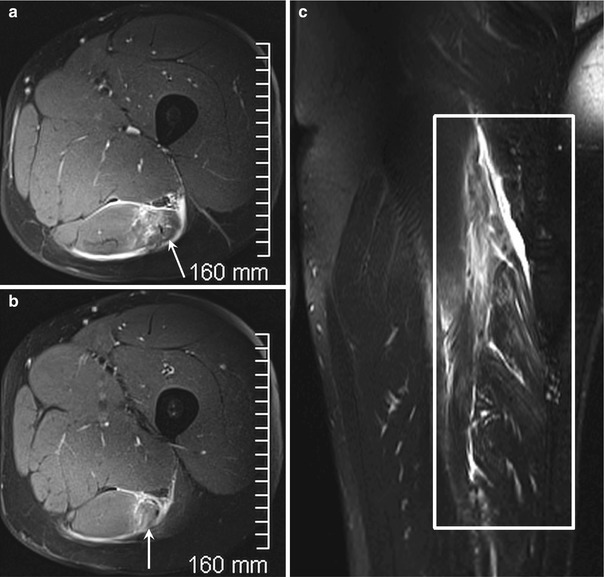
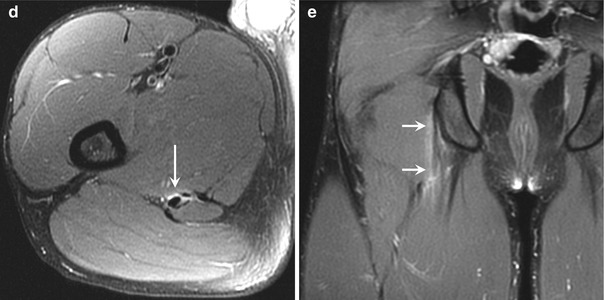
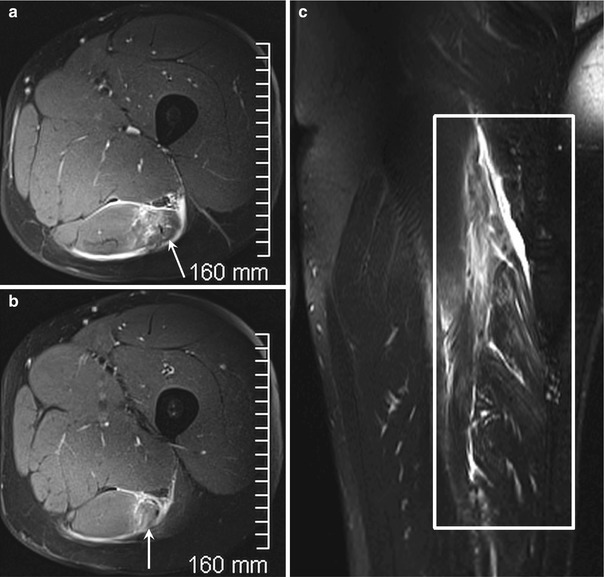
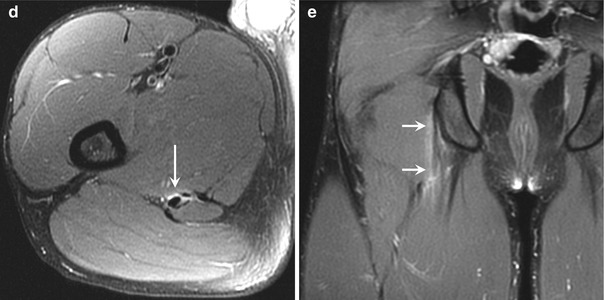
Fig. 10.15
Proximal biceps femoris myotendinous junction tear. (a, b) Axial fat-suppressed T2-weighted and (c) coronal fat-suppressed T2-weighted MRI of the left thigh in a 30-year-old man who felt a tearing sensation in the posterior thigh while at full sprint demonstrate edema within and surrounding the proximal biceps femoris myotendinous junction (arrows in a, b). Waviness of fibers is best appreciated on the coronal fat-suppressed T2-weighted MRI (white rectangle). Proximal semimembranosus free tendon injury. (d) Axial fat-suppressed T2-weighted and (e) coronal fat-suppressed T2-weighted MRI of the right thigh in an 18-year-old man who experienced pain in the buttock region after lunging for the ball on the receiving end of a teammate’s pass, showing a collar of edema around (arrow in d) and along (arrows in e) the semimembranosus free tendon
While ankle impingement syndromes are usually diagnosed clinically, imaging plays a role in confirming suspected pathology and delineating the exact underlying anatomy. As mentioned earlier in this section, impingement syndromes, particularly when posterior, may be the result of a variety of anatomic relationships. Given the anatomic variations in each individual, imaging can provide a preoperative overview as well as a means to a nonsurgical alternative in the form of image-guided intervention, most often with ultrasound or fluoroscopy.
In anterior ankle impingement, radiographic assessment suffices in most cases, as the underlying pathology is almost always osseous. Though the anterior bony proliferations may be due to both enthesophytes and osteophytes, the exact distinction is usually difficult as detection typically relies on a single projection, the lateral radiograph. MRI and ultrasound may show associated synovial hypertrophy with T1 hypointense/T2 hypointense to intermediate signal intensity tissue which is hypoechoic on ultrasound (Fig. 10.25). However, MRI and ultrasound are rarely employed in anterior impingement (Robinson and White 2002).
Radiographic assessment of the posterior ankle impingement syndrome is useful for detecting anatomic predisposing conditions, which include a Stieda process, an os trigonum, a downwardly sloping posterior tibia, or a prominent posterior calcaneal tuberosity (Fig. 10.26). However, MRI provides additional information often not visible on radiographs. This can include marrow edema within or around an os trigonum or a fractured synchondrosis (fluid within the synchondrosis between the os trigonum and the posterolateral talus) (Fig. 10.27). Recall that symptoms typically appear once associated soft tissue abnormalities develop. MRI is excellent for depicting the exact precipitating pathology, which can range from thickened posterior ligaments (posterior talofibular, tibiofibular, or intermalleolar) to flexor hallucis longus stenosing tenosynovitis (Fig. 10.28).
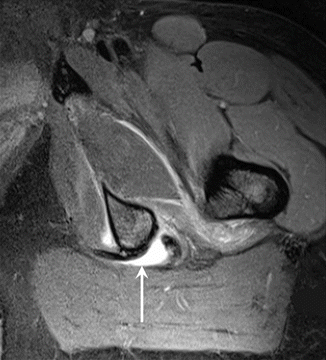
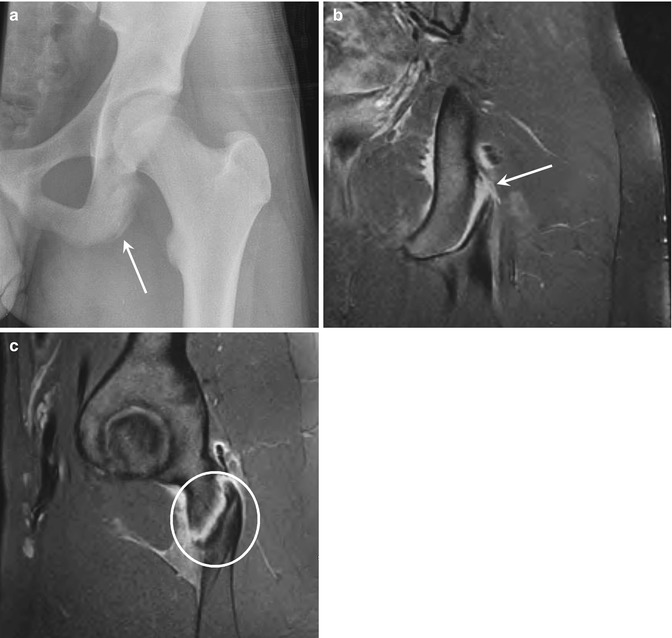
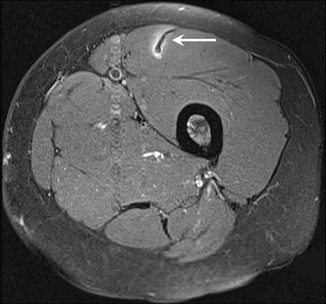
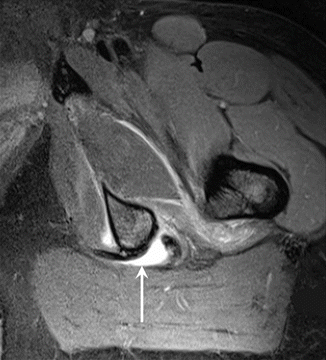
Fig. 10.16
Hamstrings tendon origin full-thickness avulsion. Oblique axial fat-suppressed proton density-weighted MRI in a 45-year-old male recreational soccer player shows a fluid gap (arrow) between the semimembranosus and conjoined tendon origins and the ischial tuberosity attachment
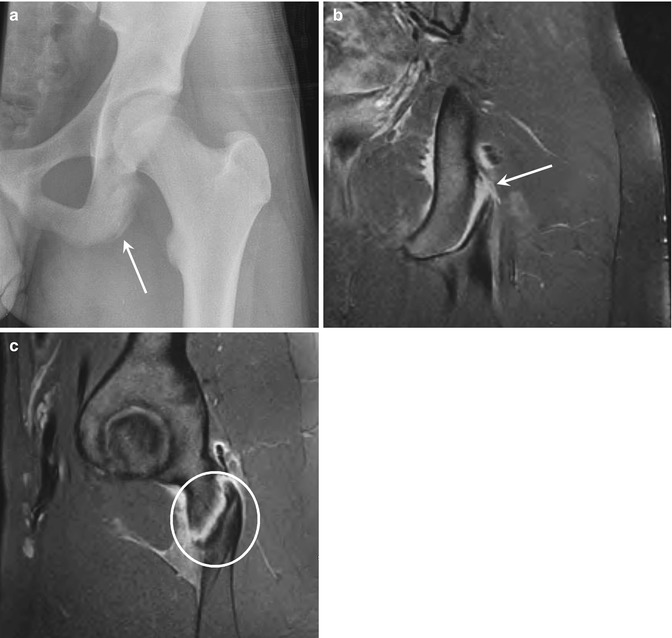
Fig. 10.17
Hamstrings (ischial tuberosity) apophyseal avulsion. (a) Radiograph and (b) coronal and (c) sagittal MRI of the left hamstrings tendon origin in a 15-year-old boy elite soccer player reveals a mildly displaced ischial tuberosity avulsion fracture (arrow in a) with subperiosteal fluid (arrow in b) and surrounding edema (circle in c)
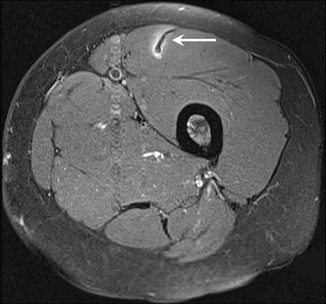
Fig. 10.18
Proximal quadriceps deep musculotendinous junction strain. Axial fat-suppressed proton density-weighted MRI of the left thigh in a 44-year-old male soccer player with pain after kicking a ball shows edema (arrow) about the proximal deep musculotendinous junction in a configuration known as the “bull’s-eye” sign (arrow)
10.4 Knee
10.4.1 Introduction
Knee injuries receive a great deal of attention in the sporting press, among soccer players, as well as from clinicians who treat their physical ailments, and for good reason. Although knee injuries are not as common as thigh strains and contusions or ankle sprains in most epidemiological studies of higher-level play, certain knee injuries have greater deleterious effect on return to play and post-injury level of play, as well as posing an inherent risk of accelerated osteoarthrosis post-injury. Furthermore, some analyses of injuries in amateurs, who constitute more than 99 % of the soccer-playing population worldwide, cite knee ligament sprains as the most common injury in this population (Herrero et al. 2014). As such, the spectrum of the most common and important knee injuries, their mechanisms, and imaging appearance should be familiar to the diagnostic radiologist. In this section, medial collateral ligament, anterior cruciate ligament, and meniscal tears in soccer players are reviewed.
Of all knee injuries in soccer, the medial collateral ligament (MCL) is the most frequently injured structure among all levels of play and all age groups. In addition to the injury mechanisms inherent in the game that place the MCL at risk, the MCL is also considered the weakest of the three main knee-stabilizing ligaments (Schweitzer et al. 1995). The UEFA injury study subgroup analysis of 346 MCL injuries found that MCL injuries are the second most common severe injury after hamstring injuries. At the professional level, a team with a roster of 25 players can expect 2 MCL injuries per season (Lundblad et al. 2013). MCL injuries are sustained during games more frequently than in training sessions at a ratio of 9:1, with the dominant leg afflicted in 60 % of MCL injuries. The mean time on the injured reserve is 23 days, less than that of other ligamentous knee injuries. Compared to other knee injuries, MCL injuries are more frequently associated with foul play and as many as 70 % of MCL injuries are a result of direct player contact. Generalized player fatigue combined with situational urgency and resultant increased intensity likely plays a major role, as a disproportionate 43 % of MCL match-related injuries occur in the last 15 min of either the first or second half. In that setting, the fatigued tackling player may be less precise with the tackle and the injured player may be less able to react to protect his body from the infraction.
Injuries to the anterior cruciate ligament (ACL), among all soccer injuries, are perhaps the most recognized and feared. Compared to the general population, soccer players are at a 100–1000-fold increased risk of tearing the ACL. ACL tears require surgical treatment more frequently than any other soccer knee injury (Arsht et al. 2005). A tear of the ACL is associated with a longer return to play than any of the other typical soccer knee injuries, averaging 194 days in the UEFA injury study, compared with an average of 52 days for posterior cruciate ligament (PCL) injuries and 23 days for collateral ligament injuries (Lundblad et al. 2013). More so than any other common soccer knee injury, an ACL tear can be career ending (Brophy et al. 2012). Although meniscal tears requiring meniscectomy are associated with an increased risk of subsequent osteoarthritis, the risk of osteoarthritis is even greater when the ACL is also torn (Neyret et al. 1993). The ACL can tear in both contact and noncontact settings. Multiple studies have found an increased incidence of ACL tears in women compared with men at an alarming multiple of two- to tenfold (Arsht et al. 2005; Gilchrist et al. 2008; Brophy et al. 2010). Analysis of a group of adolescent girls playing with older players found that 2–7 years after sustaining an ACL tear, more than 3 out of 4 had quit playing and as many as 4 out of 5 cited ACL-related symptoms as the reason (Soderman et al. 2002). A large number of etiologies have been posited across multiple analyses to explain the increased incidence in women. Hypotheses include a higher incidence due to decreased width of the intercondylar notch, greater knee joint laxity, decreased size of the ACL, differences in lower extremity alignment, hormonal differences, alterations in muscle activation patterns, and proprioceptive and neuromuscular differences (Arendt et al. 1999). Interestingly, proprioception-centered warm-up and conditioning programs have led to a decreased rate of ACL tears (Gilchrist et al. 2008).
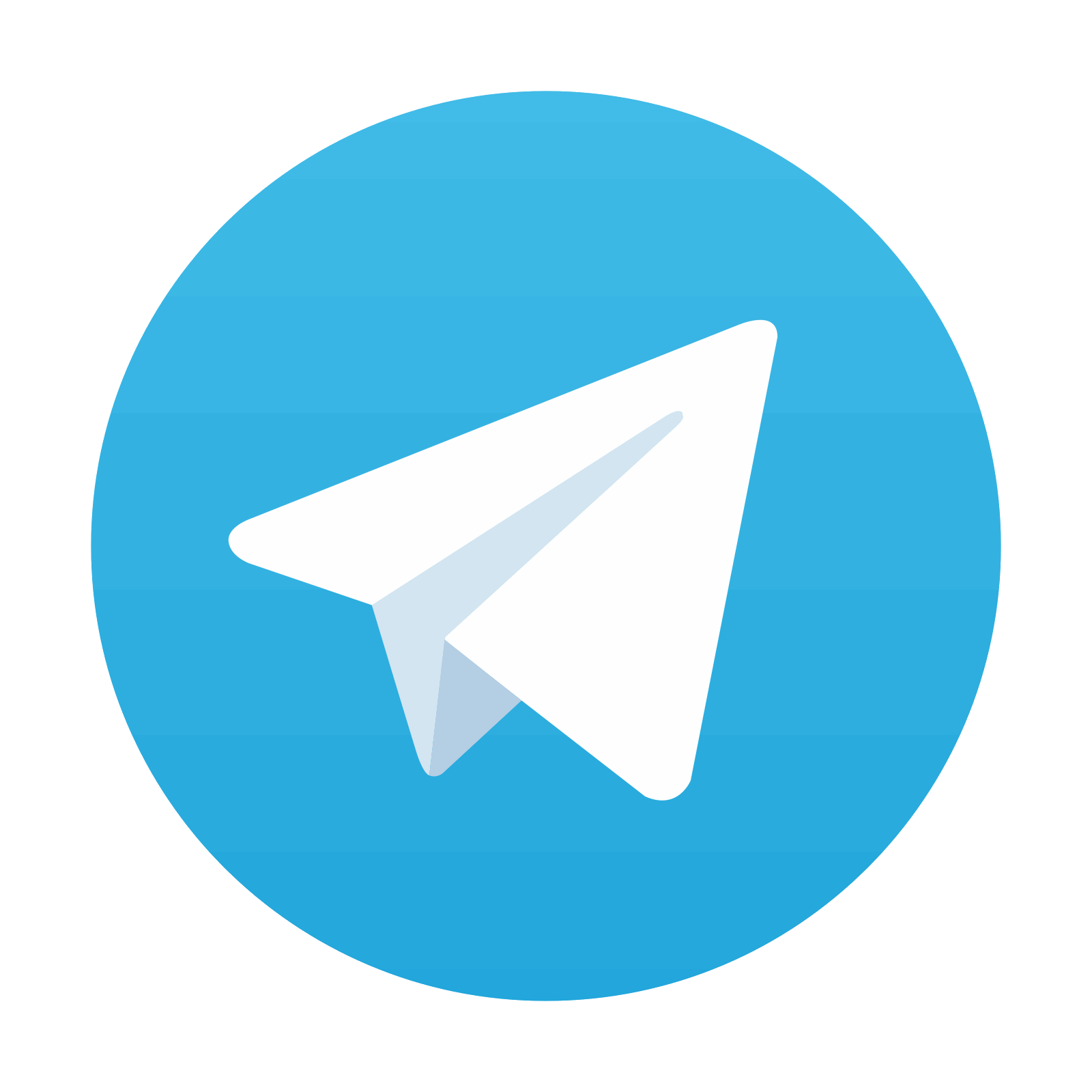
Stay updated, free articles. Join our Telegram channel

Full access? Get Clinical Tree
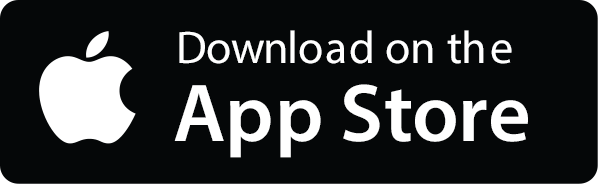
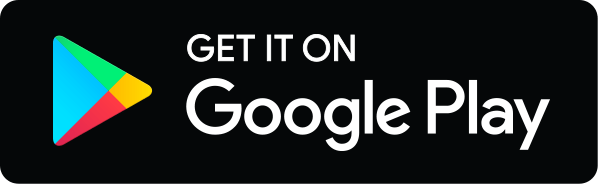