Imaging of the mind involves an understanding of the brain and imaging technology. Much progress has been made over the past 400 years in defining the mind and developing tools for its investigation. However, its definition remains elusive and is perhaps one of the greatest scientific challenges. Scientific imaging has dramatically increased in power over the past 2 decades and is now a critical tool for studying the brain, the physical seat of the mind. Whether the mind can be imaged remains to be seen.
Imaging of the what? The MIND? That was the author’s first reaction to the title of this issue, and this question will also form the basis for a final challenge at the end of this article. This topic is most challenging, and will be introduced with a brief discussion of the real object of interest—the mind—intermixed with commentary on the investigative methodology—imaging, particularly as applied to the organ of interest, the brain. The author prefaces the comments by admitting that he knows far more about the latter than the former.
The mind: part 1
The key opening question is, “What is the mind?” This question is often rhetorical or philosophical, which is not sufficient for this discussion, because the mind is the discrete object of physical investigation of every succeeding article. This topic is traditionally broached by reference to Descartes , who elaborated on the so-called “mind/body relationship” almost 400 years ago . Descartes’ solution to this dilemma is commonly summed up as dualism, in that he separated the two: the soul (incorporating what is now thought of as mind) being purely mental and distinct from the corporal body. The soul was spiritual and closely related to God, whereas the body was physical and directly interacted with its earthly environment. The two met in some strange fashion in the pineal gland, which at least brought a part of the brain into play.
These early propositions of Descartes were, like nearly all such thoughts, a function of the contemporary knowledge, plus focused attention to novel information or questions. In Descartes’ case, God and Church dominated fifteenth century philosophy and religion, and little was known about the body, especially the brain. This intellectual environment heavily influenced the dualistic solution: the soul and mind were considered close to God and were separated from the body that is part of and relates to the physical world through “nerve fibrils and animal spirits” . These ideas were initially posed just before the condemnation of Galileo, whose political situation was known to Descartes.
The early acceptance of this dualistic separation of the soul/mind from the brain/body created several problems that defined and limited subsequent development of this topic for several hundred years. The definition of soul/mind as spiritual made it difficult, if not explicitly, impossible to study, and the linkage of the soul/mind to God made certain positions heretical. One of Descartes’ explicit experimental maxims was to “obey the laws and customs of my country and religion” . Hence, much effort of many great thinkers, including Spinoza and Leibniz , pursued the soul/mind problem through religion, philosophy, and metaphysics, but not science. Experimental scientific methodology was just being defined at this time. In fact, scientific imaging probably began at this time with Galileo. Although historically interesting, most of this early work is at best remote, if not irrelevant, to contemporary concepts of the mind, or certainly the brain.
Descartes’ dualism might better be considered a quadralism involving soul/mind/brain/body. Fortunately, during the Enlightenment, punctuated by the French Revolution, the role of the soul and God in this dialog was dramatically reduced, and the mind became the focus of intellectual attention, a subject to which we shall later return.
On the other side of the equation, the brain was becoming more intrinsically linked with the body in an operational sense. Structuralists (ie, anatomists) not only described the nervous system, but also experimentally showed abnormalities of bodily functions as a sequence of destructive lesions within it. Physiologists, including Galvani , Rolando , and Fritsch and Hitzig , showed an electrically excitable continuum from the cerebrum through nerves to muscle. The brain became recognized as a central control organ of the body by the mid-nineteenth century.
The brain
Given the current depth of knowledge about the brain, it is difficult to appreciate that barely 200 years ago this organ was almost a complete mystery, particularly as to its function. Although the brain has been recognized as an organ since antiquity, no functional role was ascribed to it until Descartes placed the soul in the pineal gland. Before this intriguing but erroneous concept, more functional importance was attributed to the fluid in the ventricles than to the brain itself. In the late fifteenth, the sixteenth, and the early seventeenth centuries, in parallel to but distinct from the philosophical musings on the mind, scientific investigations of the body, including the brain, commenced. Descartes’ nonscientific attribution of the soul to the pineal gland was, fortunately, quickly followed by the much more rigorous description of the structure of the brain and nerves by Willis . Although Willis’ application of scientific observations to the brain was seminal, the primitive scientific tools available limited his direct observations to anatomy, which unto itself doesn’t convey function. However, despite little direct evidence, Willis began to argue that certain functions, including mentation, reside in the brain, as do certain diseases such as epilepsy. The scientific tools necessary to prove his assertions through actual observation of physiology, molecular biology, and other functional aspects of the brain were still several centuries away.
The brain was found to have strong correlations between structure (anatomy) and function (behavior). This intimate relationship provided the basis for the still robust field of experimental neuroanatomy. Experimental neuroanatomy, specifically the destruction of a portion of the brain in an animal followed by observations of its altered behavior, allowed eighteenth and early nineteenth century scientists, such as Gall , to make structure/function correlations that documented the brain’s direct influence on the body. Unfortunately Gall subsequently extended his work into phrenology, a false science. Because performing debilitating experiments on human beings has never been appropriate, many fundamental questions pertaining to human brain function persisted until the natural science version of experimental neuroanatomy was introduced by clinicians such as Morgagni , who attributed neurologic deficits, such as hemiparesis, to grossly destructive lesions of patients’ brains found at subsequent autopsies. Broca , in 1860, applied this lesion/deficit correlation to a patient who had experienced the acute onset of aphasia and whose brain showed an infarct in the left frontal operculum at autopsy, thus localizing a component of speech to a particular cortical region. This dysfunctional imaging was subsequently used by many clinical scientists, particularly the nineteenth and early twentieth century neurologists whose names are attached to so many neurologic syndromes. Although lesion/deficit correlation has been a very informative means of studying the brain, it is limited by its morphologic basis, which does not provide any direct information about the brain’s physiologic or molecular mechanisms.
Although anatomists and physiologists clearly and tightly linked the brain to the body, the mind with its mental thoughts and abstractions remained recalcitrant to union with the brain. Spencer , with the publication of his Principles of Psychology in 1855, made major contributions to the mind/brain problem. He built on the concept of the simple reflex, which was viewed as a behavioral response of an organism to its environment; the physical substrate of that response was the nervous system. Arguing from an evolutionary perspective, Spencer proposed that any behavior, whether in a primitive organism or man, whether involving the simplest reflex or most complex thought, was an adaptation of an organism to its environment. He had two important supporting arguments: continuity and development. Life is a continuum from the simple to the complex. Development is the change from homogeneity to heterogeneity, from relative unity and indivisibility to differentiation and complexity. Although speaking of heterogeneity in general, Spencer explicitly extended the argument to spatial heterogeneity of the brain when he stated that, “no physiologist who calmly considers the question with the general truths of his science can long resist the conviction that different parts of the cerebrum subserve different kinds of mental action” . With this statement, Spencer not only unites the mind and brain but also establishes the importance of imaging, as shall now be shown.
The brain
Given the current depth of knowledge about the brain, it is difficult to appreciate that barely 200 years ago this organ was almost a complete mystery, particularly as to its function. Although the brain has been recognized as an organ since antiquity, no functional role was ascribed to it until Descartes placed the soul in the pineal gland. Before this intriguing but erroneous concept, more functional importance was attributed to the fluid in the ventricles than to the brain itself. In the late fifteenth, the sixteenth, and the early seventeenth centuries, in parallel to but distinct from the philosophical musings on the mind, scientific investigations of the body, including the brain, commenced. Descartes’ nonscientific attribution of the soul to the pineal gland was, fortunately, quickly followed by the much more rigorous description of the structure of the brain and nerves by Willis . Although Willis’ application of scientific observations to the brain was seminal, the primitive scientific tools available limited his direct observations to anatomy, which unto itself doesn’t convey function. However, despite little direct evidence, Willis began to argue that certain functions, including mentation, reside in the brain, as do certain diseases such as epilepsy. The scientific tools necessary to prove his assertions through actual observation of physiology, molecular biology, and other functional aspects of the brain were still several centuries away.
The brain was found to have strong correlations between structure (anatomy) and function (behavior). This intimate relationship provided the basis for the still robust field of experimental neuroanatomy. Experimental neuroanatomy, specifically the destruction of a portion of the brain in an animal followed by observations of its altered behavior, allowed eighteenth and early nineteenth century scientists, such as Gall , to make structure/function correlations that documented the brain’s direct influence on the body. Unfortunately Gall subsequently extended his work into phrenology, a false science. Because performing debilitating experiments on human beings has never been appropriate, many fundamental questions pertaining to human brain function persisted until the natural science version of experimental neuroanatomy was introduced by clinicians such as Morgagni , who attributed neurologic deficits, such as hemiparesis, to grossly destructive lesions of patients’ brains found at subsequent autopsies. Broca , in 1860, applied this lesion/deficit correlation to a patient who had experienced the acute onset of aphasia and whose brain showed an infarct in the left frontal operculum at autopsy, thus localizing a component of speech to a particular cortical region. This dysfunctional imaging was subsequently used by many clinical scientists, particularly the nineteenth and early twentieth century neurologists whose names are attached to so many neurologic syndromes. Although lesion/deficit correlation has been a very informative means of studying the brain, it is limited by its morphologic basis, which does not provide any direct information about the brain’s physiologic or molecular mechanisms.
Although anatomists and physiologists clearly and tightly linked the brain to the body, the mind with its mental thoughts and abstractions remained recalcitrant to union with the brain. Spencer , with the publication of his Principles of Psychology in 1855, made major contributions to the mind/brain problem. He built on the concept of the simple reflex, which was viewed as a behavioral response of an organism to its environment; the physical substrate of that response was the nervous system. Arguing from an evolutionary perspective, Spencer proposed that any behavior, whether in a primitive organism or man, whether involving the simplest reflex or most complex thought, was an adaptation of an organism to its environment. He had two important supporting arguments: continuity and development. Life is a continuum from the simple to the complex. Development is the change from homogeneity to heterogeneity, from relative unity and indivisibility to differentiation and complexity. Although speaking of heterogeneity in general, Spencer explicitly extended the argument to spatial heterogeneity of the brain when he stated that, “no physiologist who calmly considers the question with the general truths of his science can long resist the conviction that different parts of the cerebrum subserve different kinds of mental action” . With this statement, Spencer not only unites the mind and brain but also establishes the importance of imaging, as shall now be shown.
Imaging
Note that all early methods of studying the brain involved some form of imaging, albeit with the naked human eye visualizing gross anatomic structures. Imaging is a critical tool for science in general and the brain in particular. Why? Because of the nature of the universe. An intrinsic feature of the universe, and most objects within it, is spatial (and temporal) heterogeneity. The universe is not a homogeneous distribution of one thing that just sits there, but is rather an extraordinarily complex distribution of many things—some located here, others there, and all changing from now to then. Some elements of the universe are highly local, dense aggregates of matter, such as rocks that move slowly, whereas other elements are broader, looser distributions, such as energy fields that move at the speed of light. However, this very complex universe can be simply described (macroscopically) as: U = m/E ∗ x,y,z ∗ t , where U is universe; m is mass; E is energy; x, y, and z are spatial dimensions; and t is time. Stated verbally, the universe consists of locally varying distributions of mass and energy, and the intrinsic and intense spatial and temporal heterogeneity make imaging so important. The brain exquisitely exemplifies this nature and need.
One of the highest attributes of the human species is cognition, which allows individuals to learn and think about and interact with the environment. Scientific investigation of the surroundings is one of the most sophisticated activities of the human mind. Imaging is mandatory for the scientific investigation of that environment and has historically played a central role in the development of the scientific method and its applications. An image is a map, or spatially coherent display of spatially and temporally defined measurements. An image can be formalized as: I = fm/E ∗ x,y,z ∗ t , where I, an image, is a function of measurements of mass/energy, space, and time. Note the close correspondence of this equation to the prior formulation of the universe. An image is a visual display of measurements of mass or energy as a function of location. Imaging is the only means of fully describing and investigating a spatially heterogeneous object.
An early and excellent example of modern scientific imaging was Galileo’s discovery of the four moons of Jupiter . Galileo used a new imaging tool, the telescope, that improved the imaging capabilities of the naked eye through expanding the field of view and increasing spatial resolution. At this point Galileo had performed novel imaging but not science. He had a choice as to what to do with this new information; he could have mythologized the images he saw and empirically filled the telescopic sky with more fantastic creatures, much as Descartes fantasized the soul in the pineal gland. Fortunately, Galileo chose the then-unorthodox scientific approach. Instead of imagining minotaurs, he used his new imaging tool to make localized measurements of energy (visible light) in the spatial vicinity of Jupiter over time (several weeks). He exquisitely displayed these sequential observations with simple images—drawings. He then applied scientific reasoning to first hypothesize and then prove mathematically that these light signals were from four previously unknown moons.
This extraordinary example of scientific imaging not only expanded the knowledge of planetary space, but also fundamentally changed the concept of the universe and mankind’s position in it. History is replete with examples of the key role of imaging in scientific discovery, including many in biomedical science. From Leeuwenhoek’s discovery of microorganisms to Rosalind Franklin’s diffraction x-ray of the DNA molecule to today’s functional imaging of the human brain, imaging has played an absolutely necessary role in biologic discovery because of the spatial (and temporal) heterogeneity of all biologic systems.
Brain imaging
Given the spatially heterogeneous nature of the brain (both structurally and functionally), imaging of the brain is an absolute necessity to document the location of an anatomic structure, experimental or natural lesion, or physiologically active region of the brain. Only with this anatomic, spatial information could an observed neurologic, psychological, or cognitive function be linked to its physical source. In human beings, early scientific investigations were severely restricted by the unfortunate necessity of a patient having to experience an insult to the brain, and the additional burden that the patient must either die and have an autopsy or submit to a craniotomy. Until recently, these were the only ways to directly document the presence and extent of a brain lesion.
Despite the many drawbacks, experimental anatomy and clinical lesion/deficit research in the first half of the twentieth century provided the basis of much of the current understanding of functional localization in the brain. During the second half of the twentieth century, these early, primitive, but informative techniques were increasingly supplemented by sophisticated histologic, neurophysiologic, and molecular biologic techniques that have combined to yield the great depth of current knowledge about the brain, knowledge that extends from single cell events to highly integrated cognitive functions. However, many of these newer techniques also have restrictions to their application in human beings, particularly intact functioning human beings. Histologic techniques require tissue, never easily obtained from human brain, and almost never from multiple or large regions. Many neurophysiologic techniques require intrusion into the brain, such as for electrode recordings or cortical stimulation. Molecular techniques are seldom feasible in the intact functioning brain.
Although contemporary scientific techniques provide extraordinarily detailed information about small parts of the brain, few, if any, provide data from the entire functioning unit. This limitation is significant because many functions of the brain involve composite actions of its many spatially, physiologically, and biochemically disparate components. This fact is particularly true of complex behavioral tasks and cognition. The spatial heterogeneity of the brain has always begged for imaging of the whole organ, preferably in the intact, functioning state.
In 1974, clinical neuroscience experienced a profound change with the invention of the x-ray CT scanner, an instrument that for the first time could noninvasively produce images of the whole living human brain . X-ray CT images are a function of differential electron density of tissues, and only subtle differences of this parameter exist in the brain. For instance, the electron densities of gray and white matter differ by only 0.5%. Hence, clinical CT scans yield crude images of the brain. In addition, these images show only static anatomy and do not reflect any physiologic or molecular aspects of the brain. Determining a conventional CT scan of a cadaver’s brain from that of a living person is difficult. However, with this technology, more and better “dysfunctional” imaging can be performed, as illustrated by the extensive structure/function correlative work by the Dimasios , who, on the basis of their work, have published a refutation of Descartes’ dualism. Noninvasive brain imaging can be performed contemporaneously with detailed neurologic, neuropsychologic, and cognitive testing. Although many relationships between the gross structure and function of the brain are now understood, the overpowering need to be able to directly see physiologic and molecular function of the brain remains. After all, it is more important to know what the brain is doing than what it looks like!
X-ray CT imaging was quickly and unexpectedly followed by the development of MRI. MRI derives from nuclear magnetic resonance (NMR), a physical phenomenon related to the behavior of nuclei in the presence of a magnetic field that was described by Bloch and colleagues in 1946. During the 1940s and 1950s, many investigators developed techniques that allowed this physical phenomenon to be exploited for the study of chemical structure. Since Ernst and Anderson introduced the Fourier transform analytical technique, NMR has been able to elucidate the detailed chemical structure of even large molecules such as proteins. The addition of graded magnetic fields to the requisite static magnetic field of NMR allows the spatial definition of a sample, MR image. Aue and colleagues suggested this concept, but it was initially implemented and applied to biologic samples by Damadian, Lauterbur, and Mansfield in the early 1970s.
Conventional MRI relies on radiosignals emitted by nuclei of molecules, particularly water. Fortuitously, gray and white matter have an approximately 20% difference in this signal. Similar differences can be found between certain pathologic tissues and normal brain. This fact accounts for the exquisite images of normal neuroanatomy and pathology, such as multiple sclerosis plaques, produced with contemporary MRI. The first decade of clinical MRI was characterized by steady improvements in the morphologic imaging capabilities of this remarkable and completely noninvasive and safe technology. However, little useful physiological information is available from conventional MRI signal, except for that related to fast-flowing fluids, such as blood. More recent MRI advances have focused on the development and application of physiologic and molecular imaging capabilities. For instance, the development of diffusion MRI that detects the microscopic diffusion of water now allows the demonstration of white matter fiber tracks of the brain . These new MRI methods are key to contemporary investigations of the brain and perhaps even the mind.
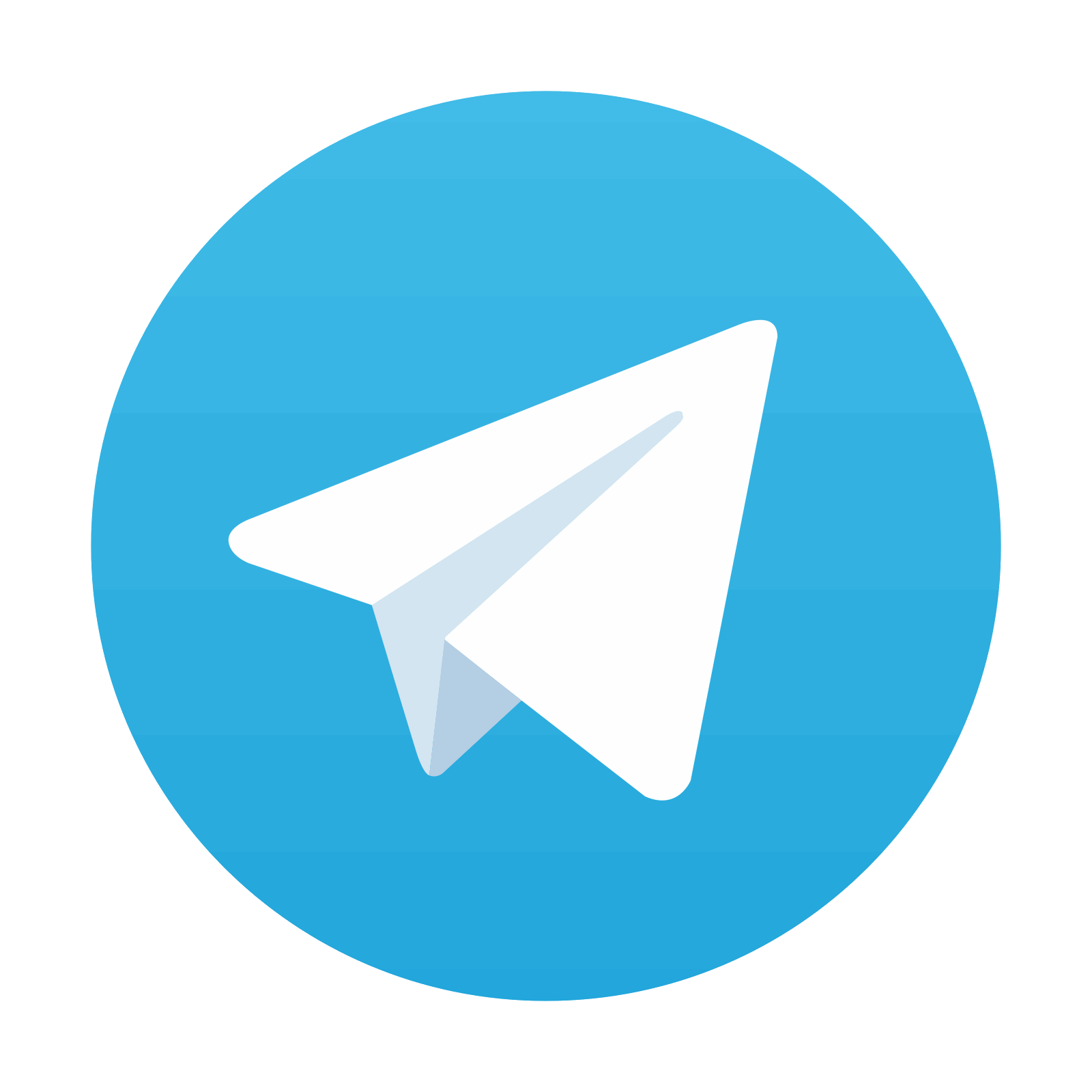
Stay updated, free articles. Join our Telegram channel

Full access? Get Clinical Tree
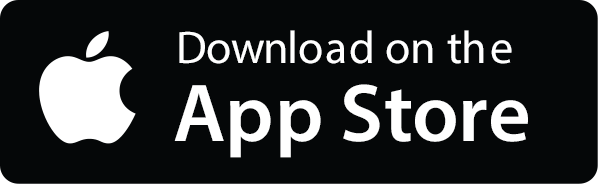
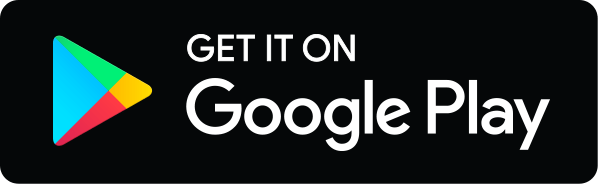