Fig. 7.1
Illustration of the anterior cranial fossa approach with skull base reconstruction using pericranial flap and dural patch, titanium mesh, and bone graft
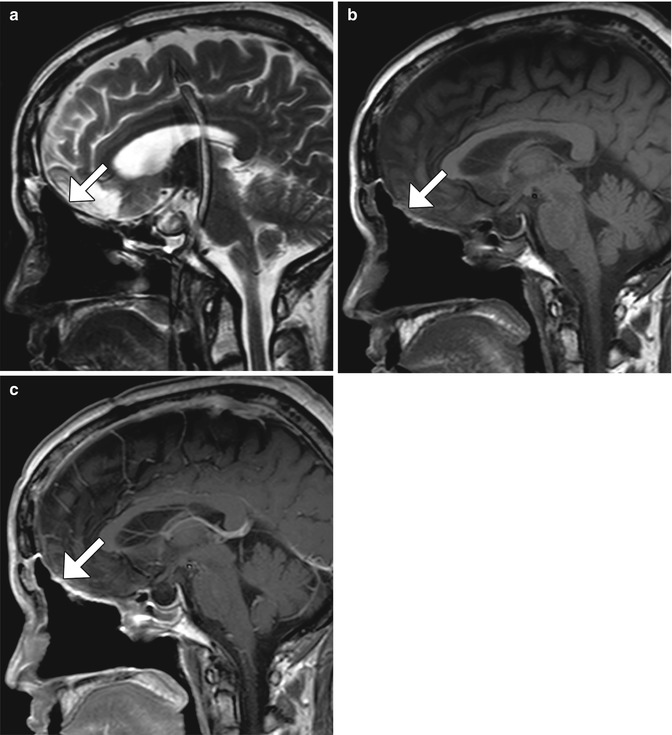
Fig. 7.2
Anterior cranial resection with vascularized pericranial flap. Sagittal T2-weighted (a), T1-weighted (b), and post-contrast T1-weighted (c) MR images show pericranial flap reconstruction of the anterior cranial fossa (arrows). The flap appears as a thin sheet that enhances
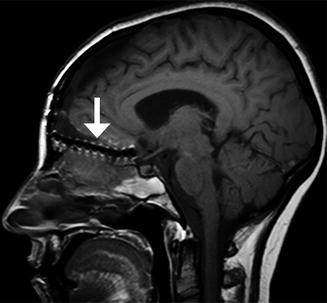
Fig. 7.3
Anterior cranial resection with mesh reconstruction. Sagittal (b) T1-weighted MRI shows the low-signal-intensity mesh positioned along the floor of the anterior cranial fossa (arrow)
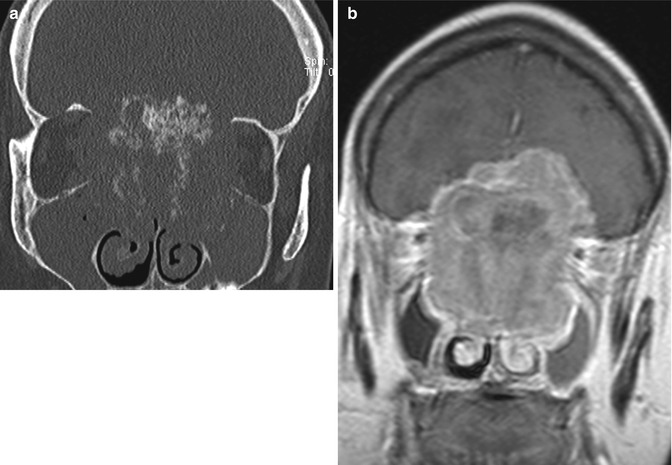
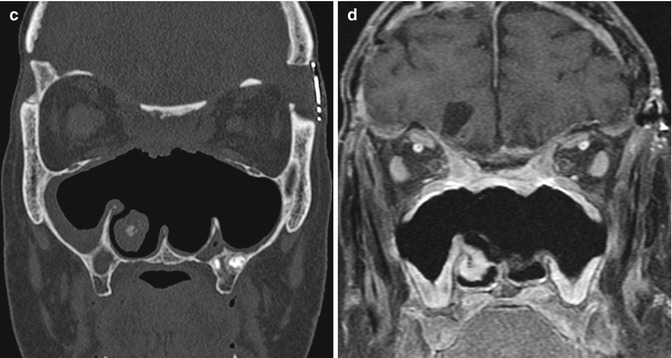
Fig. 7.4
Anterior cranial resection with bone graft reconstruction. The patient has a history of a large sinonasal undifferentiated carcinoma (SNUC) involving the anterior skull base treated via anterior craniofacial resection. Preoperative coronal CT image (a) and coronal post-contrast T1-weighted (b) MRI show the heterogeneously enhancing paranasal sinus mass extending through the cribriform plate and into the anterior skull base. Postoperative coronal (c) CT image shows extensive paranasal sinus and skull base resections. There are no residual ethmoid cells. A split calvarial bone graft harvested from the frontal bone was used to close the skull base defect. Postoperative coronal post-contrast T1-weighted (d) MRI also shows the extensive anterior craniofacial resection. The low-signal-intensity anterior skull base bone graft lies superior to the pericranial flap. There is mucosal thickening, but no evidence of residual or recurrent tumor
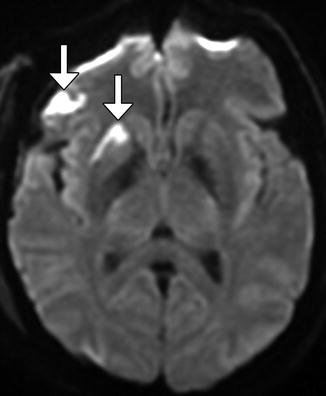
Fig. 7.5
Cerebral infarction. Axial diffusion-weighted image obtained after recent anterior cranial resection shows restricted diffusion in the right putamen and opercular region of the right frontal lobe, likely secondary to retraction (arrows)
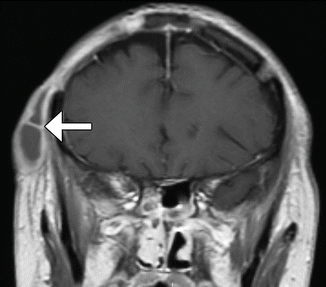
Fig. 7.6
Scalp abscess. Coronal post-contrast T1-weighted MRI shows a loculated, rim-enhancing collection in the right scalp adjacent to an osteotomy site (arrow)
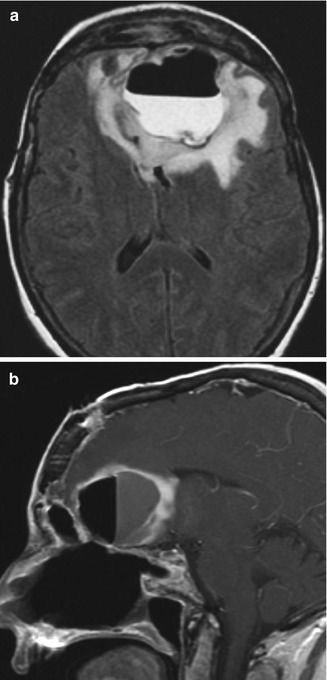
Fig. 7.7
Intraparenchymal abscess with fistula. The patient presented with fever after esthesioneuroblastoma resection. Axial T2 FLAIR (a) and sagittal (b) post-contrast T1-weighted MR images show a large left anterior frontal lobe rim-enhancing cavity containing an air-fluid level. There is extensive signal abnormality surrounding the abscess, which represents cerebritis
Follow-up imaging is important for monitoring tumor recurrence. MRI is the study of choice for postoperative surveillance (Fig. 7.8). Following craniofacial resection, MRI often demonstrates enhancing soft tissue related to granulation tissue formation at the resection site in the superior nasal cavity that is difficult to differentiate from residual or recurrent tumors, such as esthesioneuroblastoma. FDG-PET/CT can also be useful for evaluating for the presence of posttreatment tumor, although infection and inflammation of the resection bed can be hypermetabolic, similar to recurrent tumor.
Radiation therapy is often administered for malignant tumors treated via anterior craniofacial resection. This can result in radiation necrosis, which has a characteristic pattern of white matter signal abnormality and ring-enhancing lesions in the distribution of radiation field and mainly occurs 6 months to 1 year after treatment (Fig. 7.9).
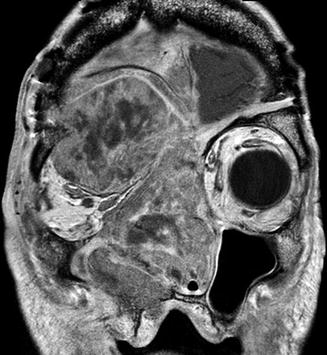
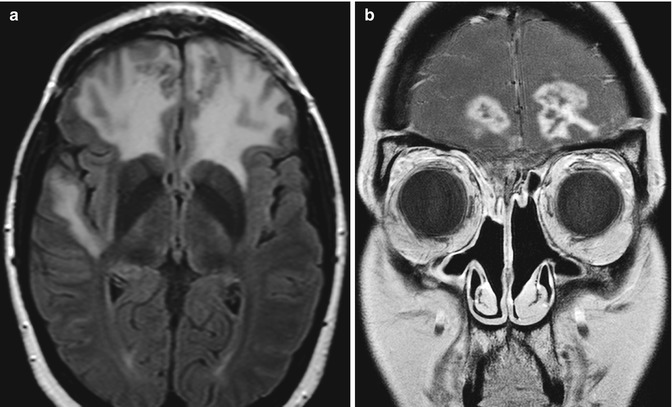
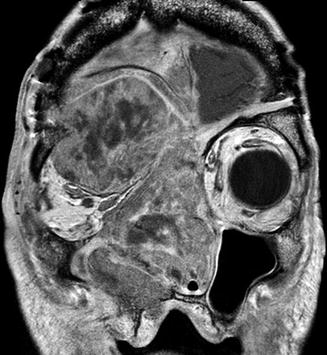
Fig. 7.8
Squamous cell carcinoma recurrence after anterior cranial fossa resection. Coronal post-contrast T1-weighted MRI shows a large heterogeneously enhancing craniofacial mass that extends across the craniotomy into the intracranial compartment and right orbit
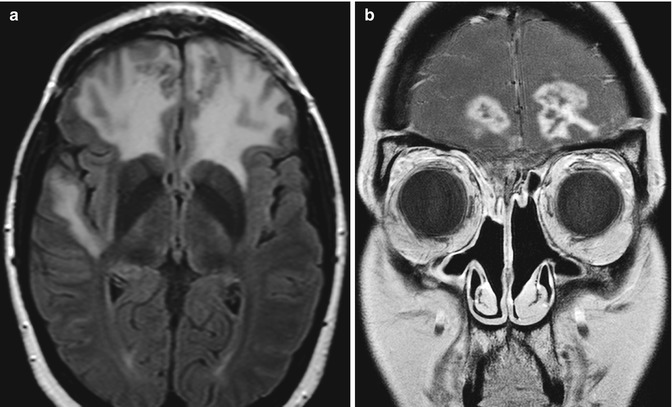
Fig. 7.9
Radiation necrosis. Axial T2 FLAIR (a) and coronal (b) contrast-enhanced T1-weighted MR images show extensive bifrontal edema and heterogeneous peripherally enhancing lesions, which are in the distribution of the radiation field after anterior cranial resection
Another important complication of anterior cranial fossa resection is encephalocele, particularly if only a pericranial flap was used to repair the skull base defect. On CT, a postoperative encephalocele appears as nonspecific soft tissue attenuation with variable amounts of surrounding cerebrospinal fluid attenuation. Thus, MRI is useful for making the diagnosis since the continuity of the lesion with the intracranial brain parenchyma can be readily established and differentiated from tumor recurrence or sinus mucosal disease (Fig. 7.11).
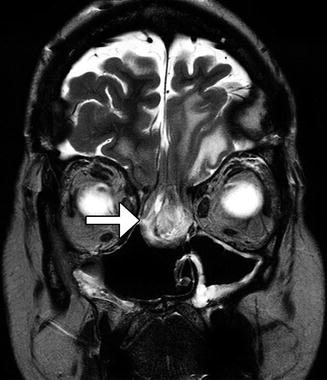
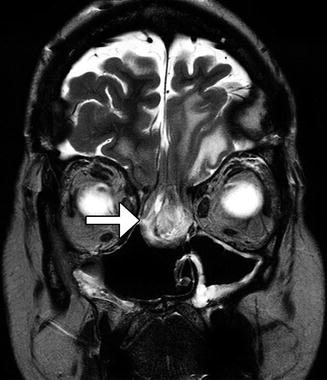
Fig. 7.10
Encephalocele after anterior cranial resection. Coronal T2-weighted MRI demonstrates a large encephalocele (arrow) through the anterior cranial fossa defect
Since anterior cranial fossa resection typically involves access through the paranasal sinuses in addition to craniotomies, there is the risk of transgressing the lamina papyracea and orbital entry. This may injure the rectus muscles and other orbital contents (Fig. 7.11). Other complications associated with FESS can also occur with anterior cranial fossa resection. As the normal air flow through the nasal sinuses is frequently disrupted, mucocele formation and chronic inflammatory changes in the paranasal sinuses are common.
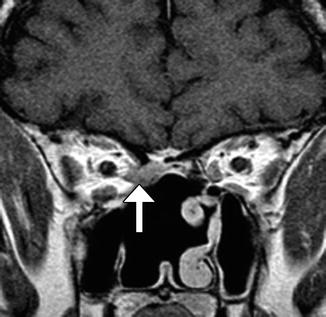
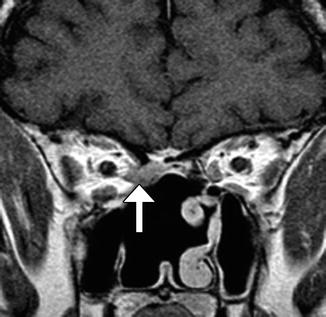
Fig. 7.11
Rectus muscle injury. The patient presented with right restrictive esotropia following anterior cranial resection and radiation treatment of a squamous cell carcinoma. Coronal post-contrast T1-weighted MR image shows enhancing, amorphous soft tissue material in the right posterior ethmoid air cells that represents scar, which retracts the medial rectus muscle (arrow) through a defect in the lamina papyracea
7.2 Decompression of Cystic Craniopharyngiomas
7.2.1 Discussion
Resection of craniopharyngiomas often poses a surgical dilemma since gross total resection is difficult to achieve with large tumors without injury to surrounding structures. Consequently, residual tumor often remains despite additional radiation and chemotherapy. Nevertheless, the main gain of surgery is to the associated reduce mass effect. Subtotal decompression can be accomplished via transsphenoidal or transcranial cyst fenestration, with or without permanent catheter implantation (Figs. 7.12 and 7.13), and can be a suitable alternative to resection for providing patients with symptomatic relief, such as visual recovery. While CT is adequate for confirming the positioning of catheters, multiplanar and multisequence MRI is better suited for delineating the cystic versus solid components, which can evolve considerably following treatment and have complex features on follow-up exams. Ultimately, the goal of follow-up imaging is to determine if growth has occurred with associated complications, such as hydrocephalus, and if there is a dominant cystic component that could be targeted in a minimally invasive manner (Fig. 7.14). Postoperative abscess can potentially mimic cyst progression on MRI, but the clinical presentation and presence of new restricted diffusion may help suggest infection (Fig. 7.15).
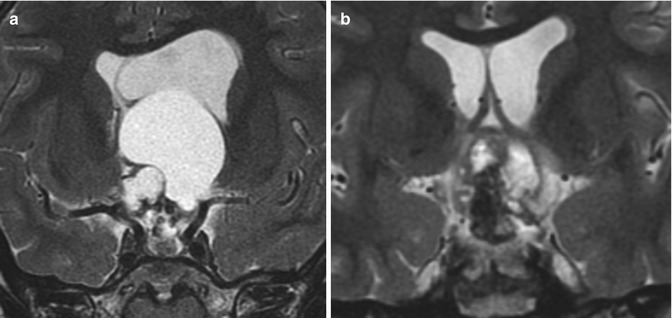
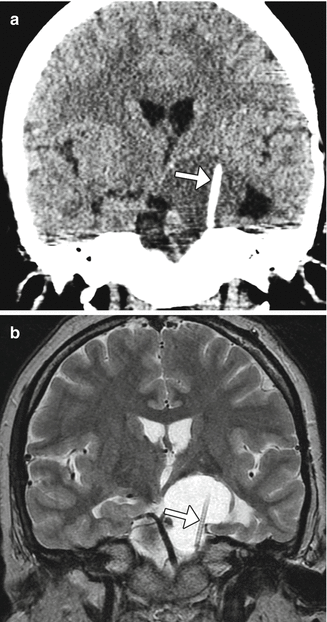
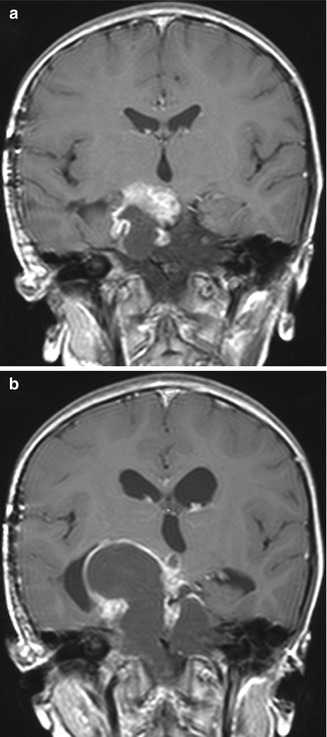
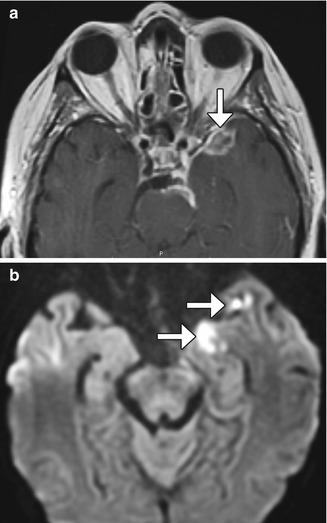
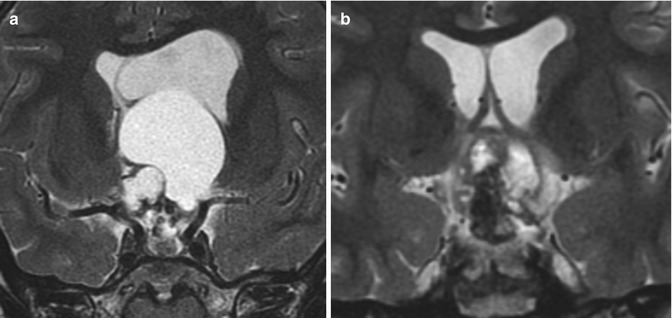
Fig. 7.12
Cyst fenestration. Preoperative coronal T2-weighted MRI (a) shows a suprasellar craniopharyngioma with a large cyst causing obstructive hydrocephalus. Postoperative coronal T2-weighted MRI (b) shows marked interval decompression of the cystic component. Although residual tumor is apparent, there is decreased mass effect
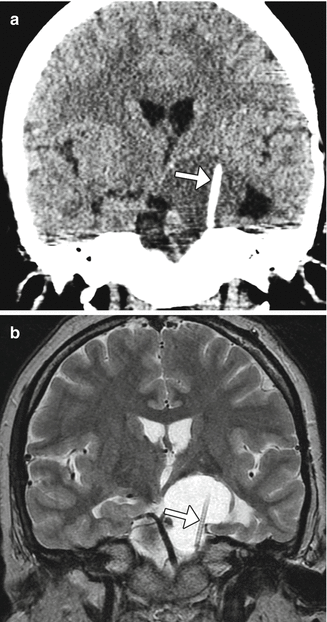
Fig. 7.13
Cyst drainage. Coronal CT image (a) and coronal T2-weighted MRI (b) demonstrate a drainage catheter (arrows) within the cystic portion of the craniopharyngioma
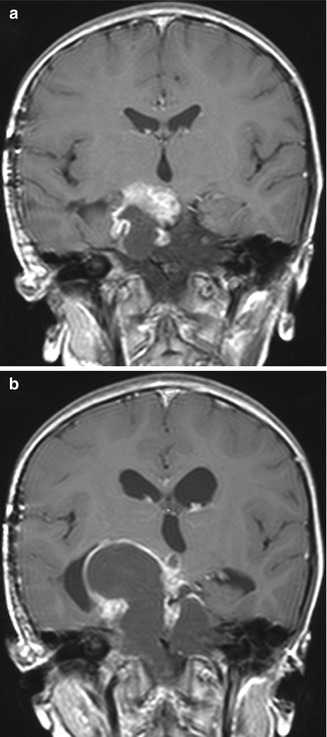
Fig. 7.14
Postoperative cyst growth. The patient underwent prior transcranial craniopharyngioma debulking, with residual enhancing and cystic suprasellar components (a). While the solid component decreased in size after radiation therapy, the cystic component increased in size and caused obstructive hydrocephalus (b), as shown on the coronal post-contrast T1-weighted MR images
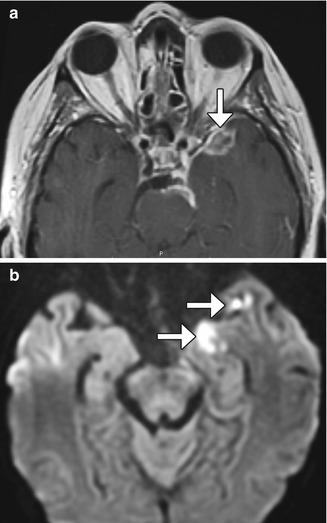
Fig. 7.15
Postoperative infection. Initial coronal contrast-enhanced T1-weighted MRI (a) obtained after anterior craniopharyngioma cyst fenestration shows a residual solid enhancing nodular component of the craniopharyngioma (arrow). Axial post-contrast T1-weighted MRI (a) and DWI (b) obtained after craniopharyngioma cyst decompression show leptomeningeal enhancement and a rim-enhancing fluid collection with restricted diffusion from abscess formation (arrows)
7.3 Transsphenoidal Tumor Resection
7.3.1 Discussion
The transsphenoidal approach is widely used for resecting pituitary tumors (hypophysectomy) and other sellar and parasellar lesions. Transsphenoidal surgery consists of accessing the sella via the nasal cavity and paranasal sinuses and typically involves some degree of resecting the posterior bony septum back to the sphenoid face and performing sphenoidotomy (Fig. 7.16). The process of drilling through bone during the transsphenoidal approach can leave behind metallic debris that has detached from the surgical instruments. These metal particles can be deposited anywhere along the path of the access route, such as in the nasal cavity and sphenoid sinus. Although it is usually too minute to be apparent on radiographs, the metal debris can cause noticeable artifact on MRI (Fig. 7.17).
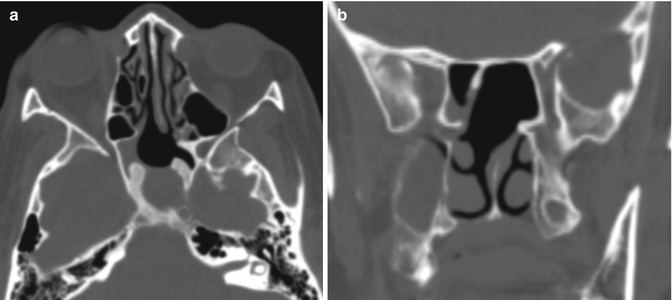
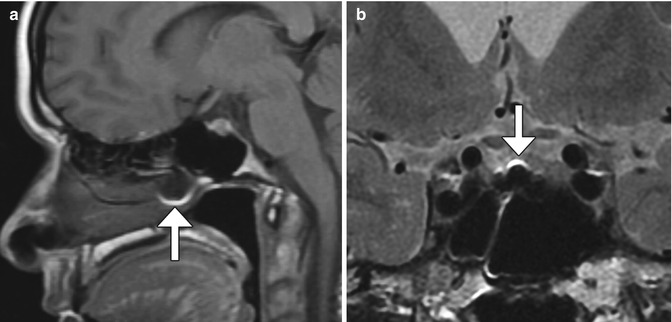
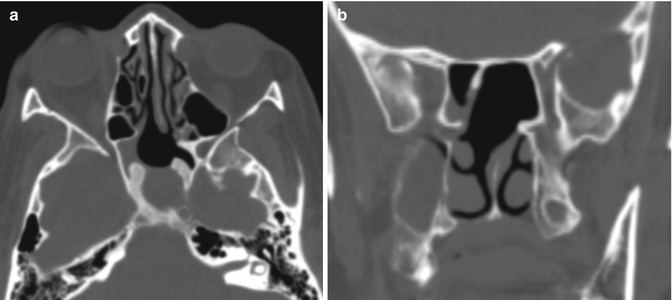
Fig. 7.16
Transsphenoidal approach. Axial (a) and coronal (b) CT images show posterior nasal septostomy and sphenoidotomy. There is also a surgical defect in the anterior wall of the expanded sella, which otherwise has thickened walls
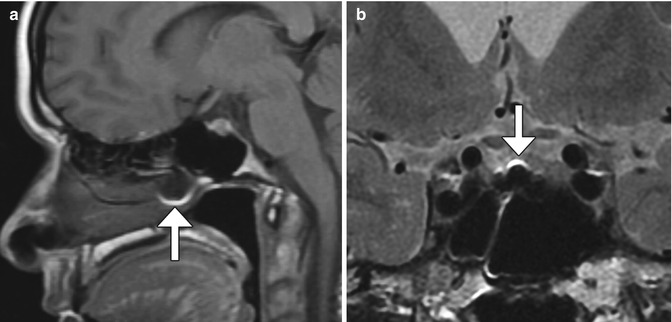
Fig. 7.17
Residual metal debris after transsphenoidal surgery. Sagittal T1-weighted MRI (a) shows metallic artifact in the posterior nasal cavity (arrow). Coronal T2-weighted MRI in a different patient (b) shows metal susceptibility artifact along the floor of the sella (arrow)
Giant adenomas or other large lesions of the pituitary region are sometimes not amenable to resection via transsphenoidal approach alone. Such tumors require craniotomy and/or a combined approach that includes transsphenoidal and transcranial routes (Fig. 7.18). Less invasive endoscopic transsphenoidal-transventricular combined approaches can also be performed in selected cases.
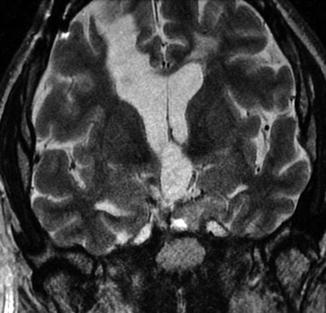
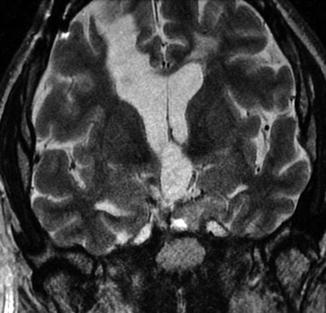
Fig. 7.18
Combined transventricular-transsphenoidal resection. Coronal T2-weighted MRI shows a linear passage through the right frontal lobe toward the sellar region, where there is residual tumor
Fat graft is commonly used to pack skull base defects after transsphenoidal resection of pituitary region tumors. The packing serves to prevent cerebrospinal fluid leakage, hemorrhage, and prolapse of intracranial contents into larger defects. Fat grafts are hyperintense on both T1- and T2-weighted sequences and decrease in size over time, such that in most cases, the fat grafts resorb completely after 1 year following surgery (Fig. 7.19).
Other materials used to seal and fill the sella include gelatin sponge (Fig. 7.20), mucosal pedicle flaps (Fig. 7.21), and titanium mesh (Fig. 7.22), each of which has particular imaging features. Then move it right after the sentence: Fat grafts are hyperintense on both T1- and T2-weighted sequences and decrease in size over time, such that in most cases, the fat grafts resorb completely after 1 year following surgery (Fig. 7.19). Bone remodeling is a chronic process that sometimes occurs after transsphenoidal resection. This phenomenon manifests as thickening, ossification, and high T1 signal intensity, most commonly along the planum sphenoidale (Figs 7.16 and 7.19).
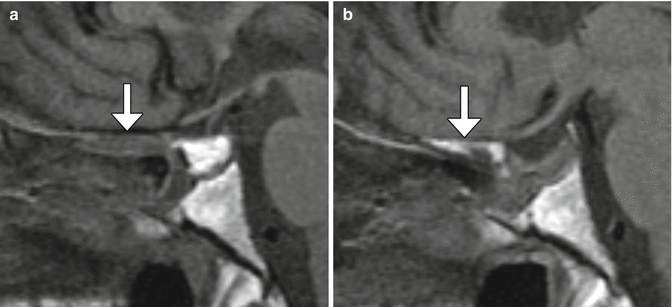
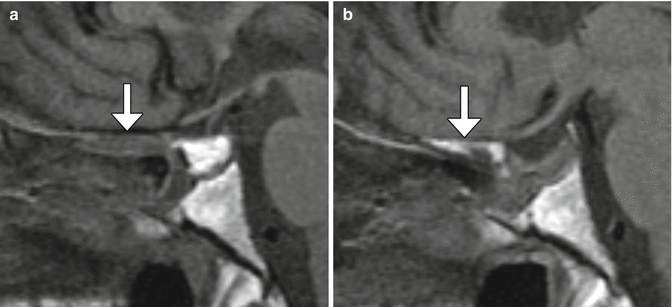
Fig. 7.19
Fat graft shrinkage and bone remodeling. Initial postoperative sagittal T1-weighted MRI (a) shows the T1 hyperintense fat graft within the sella and normal intermediate signal intensity of the planum sphenoidale (arrow). Postoperative sagittal T1-weighted MRI (b) obtained 2 years after surgery shows interval fat graft shrinkage and development of high signal intensity in the planum sphenoidale (arrow)
Nasal stents and sinonasal fluid related to bloody mucus drainage can be encountered on early postoperative imaging (Fig. 7.23).
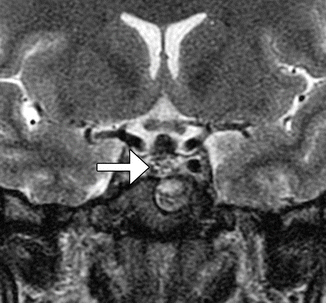
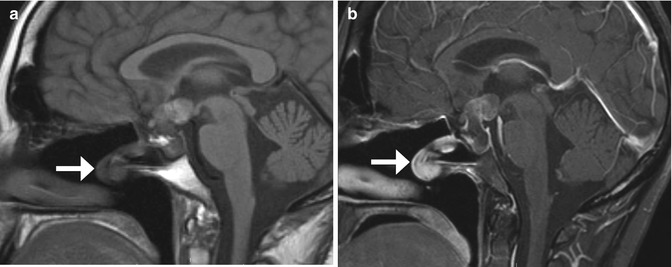
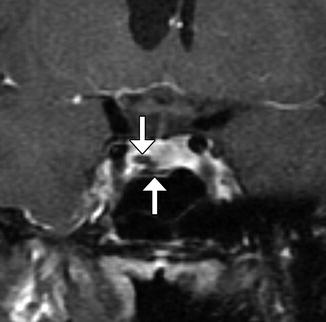
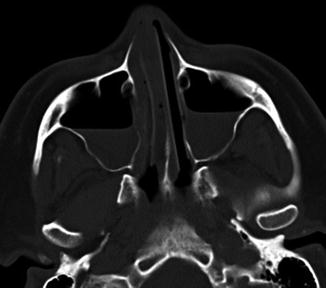
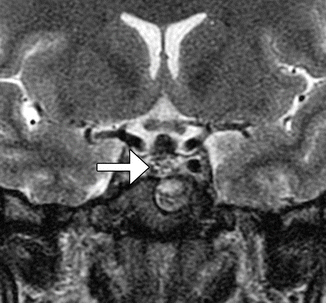
Fig. 7.20
Merocel packing. Coronal T2-weighted MRI shows the packing in the sella and sphenoid sinus, which appears as a heterogeneous blob (arrow)
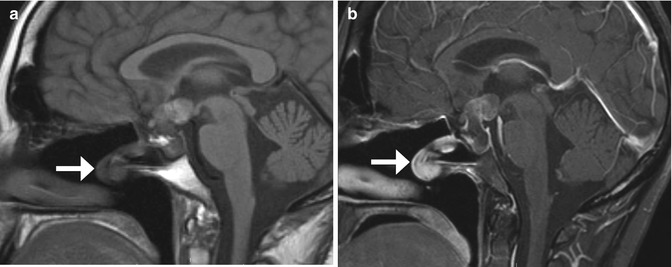
Fig. 7.21
Pedicled mucosal flap. Sagittal pre-contrast T1-weighted (a) and post-contrast sagittal T1-weighted (b) MR images show an enhancing pedicled mucosal flap (arrows) transposed into the sphenoid sinus
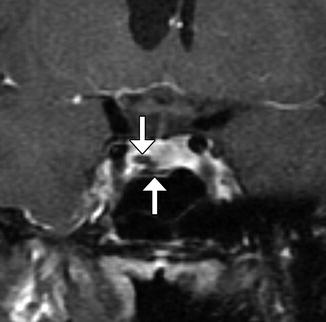
Fig. 7.22
Titanium mesh sellar reconstruction. Coronal T1-weighted MRI shows sheets of titanium mesh (arrows) along the floor of the sella
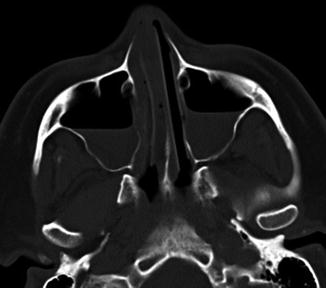
Fig. 7.23
Expected early posteroperative sinonasal findings after transsphenoidal surgery. Axial CT image shows fluid in the bilateral maxillary sinus and bialteral nasal stens
The early postoperative imaging appearance of the pituitary after transsphenoidal resection is variable, ranging from no enhancement to nodular enhancement to peripheral rim enhancement. There can also be postoeprative reexpansion of the normal pituitary gland, thickening of the pituitary stalk, and swelling of the optic apparatus. In addition, there may be a postoperative mass caused by residual tumor, edema, hemorrhage, implant material, granulation tissue, or a combination of these. In particular, granulation tissue can be difficult to differentiate from residual tumor on imaging initially. However, on follow-up, granulation tissue typically involutes, while residual tumor is expected to persist or grow (Fig. 7.24). In particular, early postoperative dynamic MRI after transsphenoidal pituitary adenoma resection can be useful for differentiating residual tumor from postoperative surgical changes. Residual tumor from subtotal resection of pituitary macroadenomas is usually distributed lateral to the sella, where it is difficult to attain and left behind in order to minimize complications (Fig. 7.25). Indeed, the primary goal of the surgery is not necessarily to remove the entire tumor, but to alleviate the mass effect upon the optic chiasm.
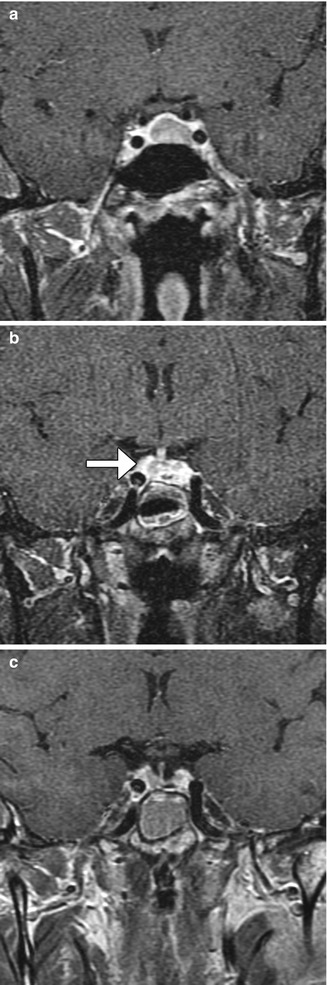
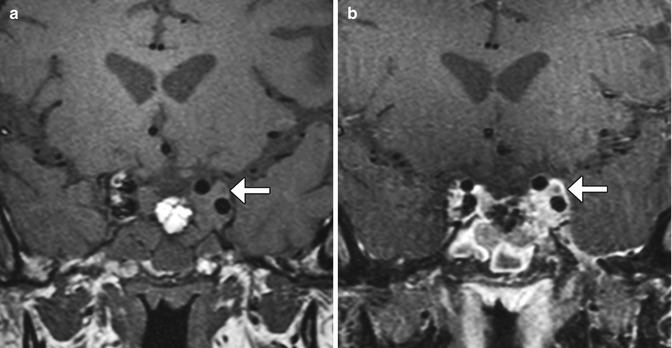
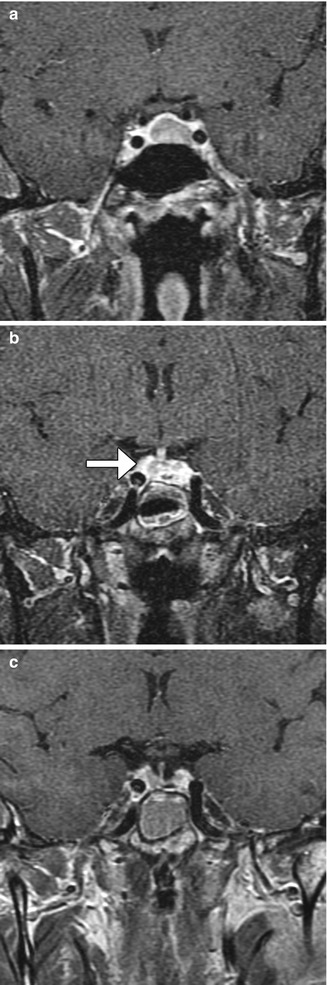
Fig. 7.24
Granulation tissue after transsphenoidal surgery. Preoperative coronal contrast-enhanced T1-weighted MRI (a) shows a pituitary adenoma. Postoperative contrast-enhanced T1-weighted MRI (b) obtained 3 months after surgery shows heterogeneously enhancing tissue in the sella (arrow). Postoperative contrast-enhanced T1-weighted MRI (c) obtained 1 year after surgery shows near resolution of the enhancing material in the sella
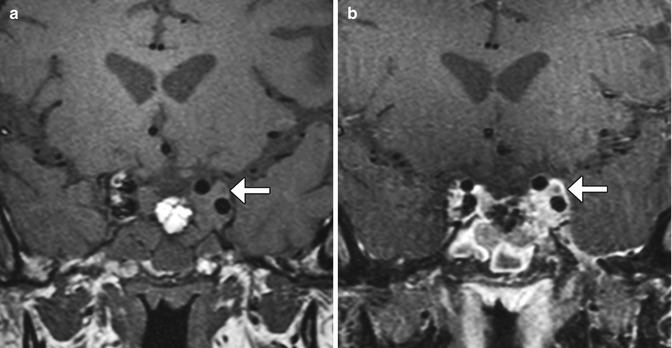
Fig. 7.25
Subtotal pituitary macroadenoma resection. Coronal T1-weighted (a) and post-contrast fat-suppressed T1-weighted (b) MR images show enhancing residual tumor extending into the left cavernous sinus (arrow), without mass effect upon the optic apparatus. There is fat packing in the sella, which drops in signal with fat suppression in contradistinction to the residual tumor, which enhances
7.4 Transsphenoidal Resection Complications
7.4.1 Discussion
Sellar hematomas are not uncommon after transsphenoidal resection. When large, these can cause mass effect upon surrounding structures and produce symptoms. Subacute hematomas in the sella can display high signal on T1- and T2-weighted MRI sequences and should not be mistaken for fat graft or residual tumor (Fig. 7.26). Gradient echo (GRE) or susceptibility-weighted imaging (SWI) techniques can sometimes be useful for identifying blood products on MRI, although susceptibility effects from air in the adjacent sphenoid sinus can limit assessment.
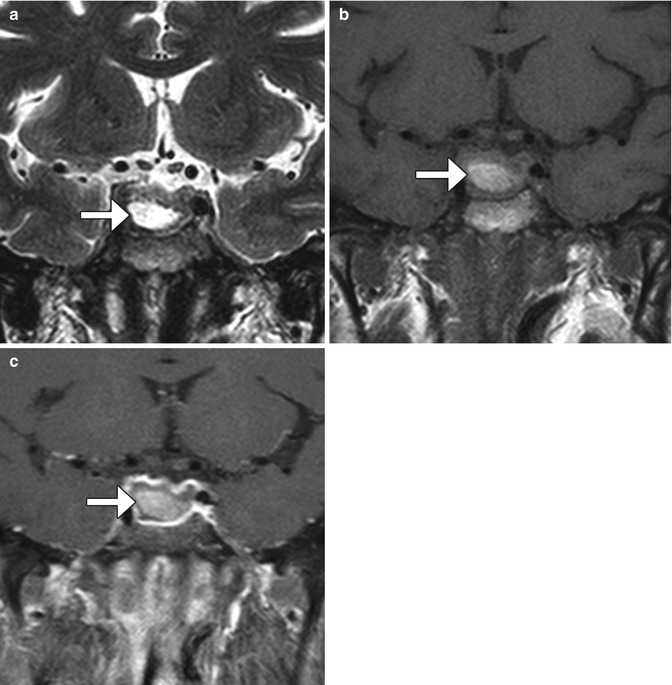
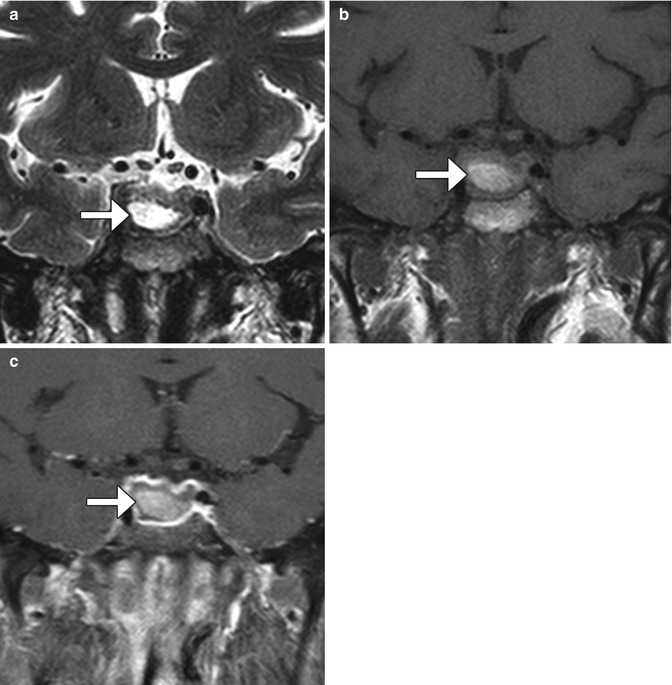
Fig. 7.26
Postoperative hematoma. Coronal T2-weighted (a), T1-weighted (b), and post-contrast T1-weighted (c) MR images show the intrinsically hyperintense fluid collection in the sella (arrows) after recent transsphenoidal surgery
Arterial injury during transsphenoidal resection is uncommon, but can manifest as pseudoaneurysm and/or subarachnoid hemorrhage, which can lead to vasospasm. Most arterial complications related to transsphenoidal surgery involve the internal carotid artery, but the ophthalmic, posterior communicating, and anterior cerebral arteries may also be affected. Arterial injury may occur during dural opening, tumor resection, or reconstruction of the sinuses and may be predisposed by anatomic variants of the sinuses and internal carotid arteries and large tumors that involve the cavernous sinus. Therefore, meticulous preoperative planning with imaging is important for minimizing arterial injury.
Once arterial injury is suspected during transsphenoidal resection, angiography is essential for identifying the presence of pseudoaneurysms. The speculum and packing material may be kept within the sphenoid sinus in order to prevent exsanguination, and excess packing may result in arterial stenosis or occlusion. Endovascular control of bleeding may be achieved by either balloon occlusion or coil embolization of the affected internal carotid artery, coil embolization of the pseudoaneurysm, or stenting alone of the affected segment of the internal carotid artery (Fig. 7.27). Peritumoral hemorrhage can lead to delayed cerebral vasospasm and associated progressive worsening neurological deficits.
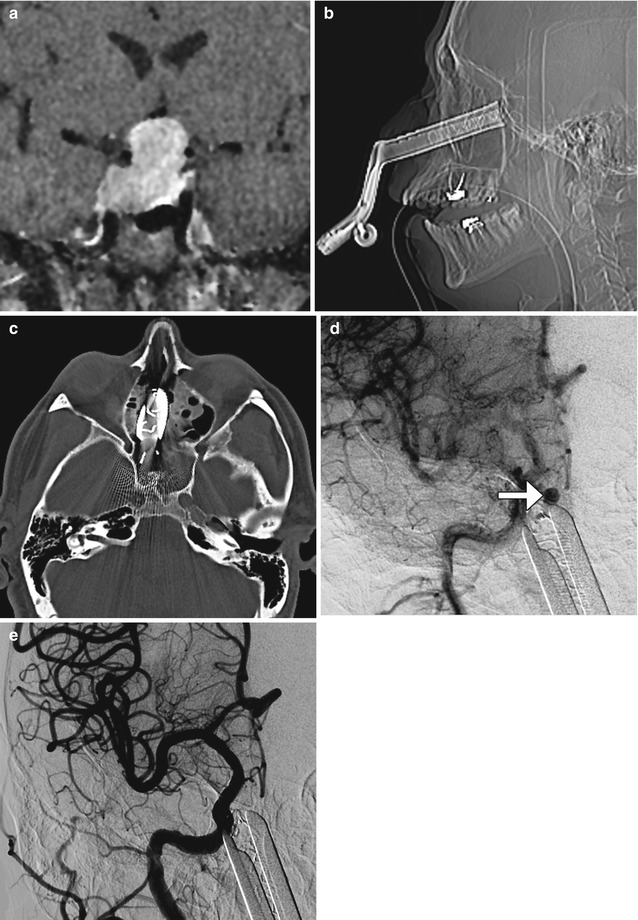
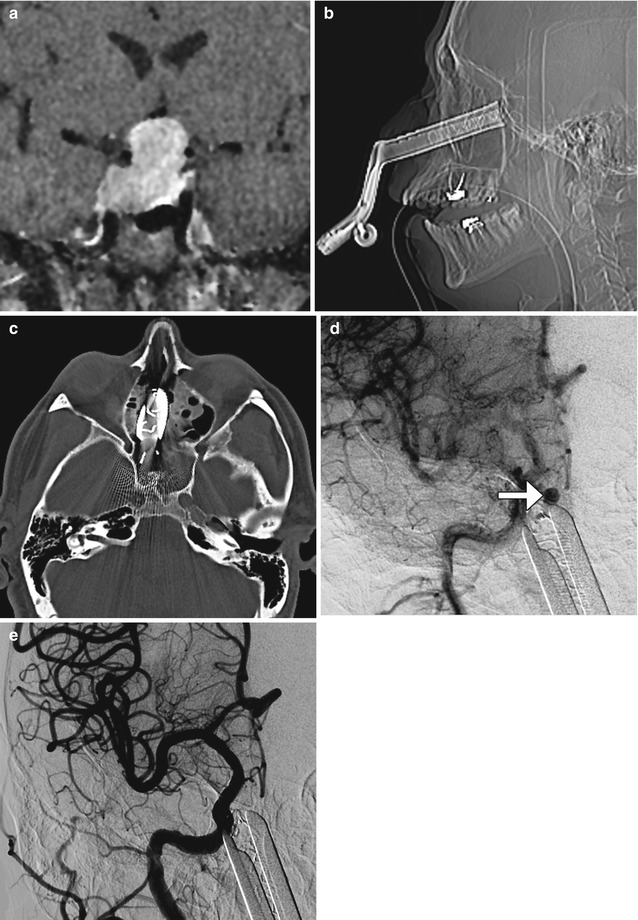
Fig. 7.27
Carotid artery injury. Preoperative coronal post-contrast T1-weighted MRI (a) shows a large pituitary adenoma that extends into the cavernous sinuses. Postoperative scout (b) and axial CT image (c) show transsphenoidal speculum. Digital subtraction carotid angiograms show a right cavernous carotid pseudoaneurysm (arrow) adjacent to the speculum (d). The pseudoaneurysm was successfully treated via endovascular coiling (e)
Malposition or migration of packing material for transsphenoidal resection is uncommon. The displaced packing material can exert mass effect upon the optic chiasm, resulting in visual symptoms that may differ from the preoperative deficits (Fig. 7.28). Alternatively, the packing material can extend posteriorly and compress the brainstem (Fig. 7.29). Such complications can be readily demonstrated on multiplanar CT or MRI. However, in some cases, displacement of packing material can potentially mimic tumor invasion.
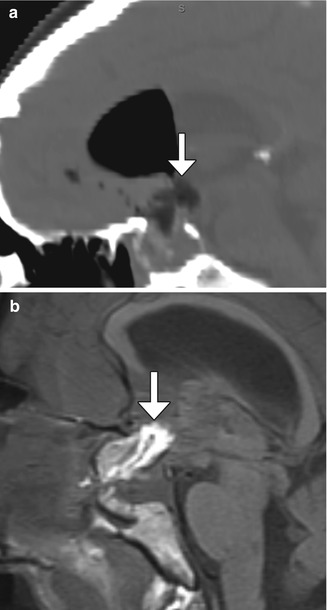
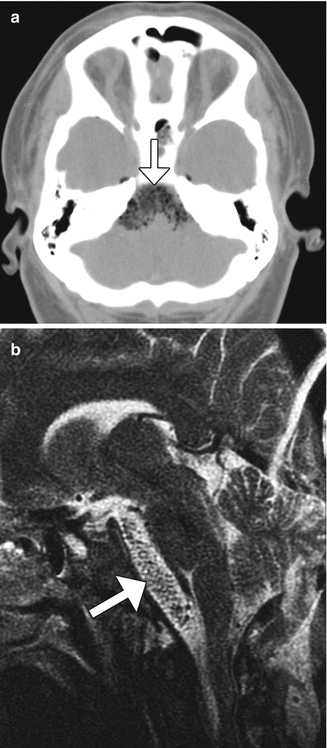
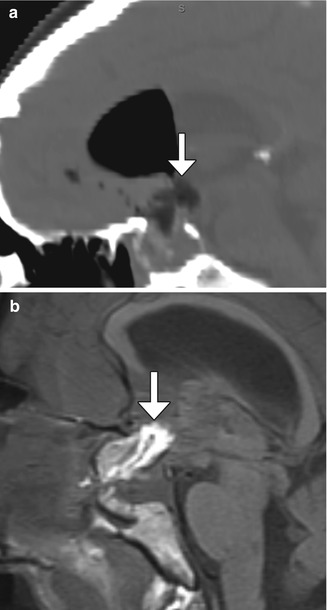
Fig. 7.28
Suprasellar fat graft compressing the optic chiasm. Within an hour of arrival in the recovery room after transsphenoidal pituitary resection, the patient was found to have new visual deficits, different from the preoperative symptoms. Sagittal CT image (a) and sagittal T1-weighted MRI (b) show the fat graft (arrows) extending into the suprasellar space. Pneumocephalus is also present
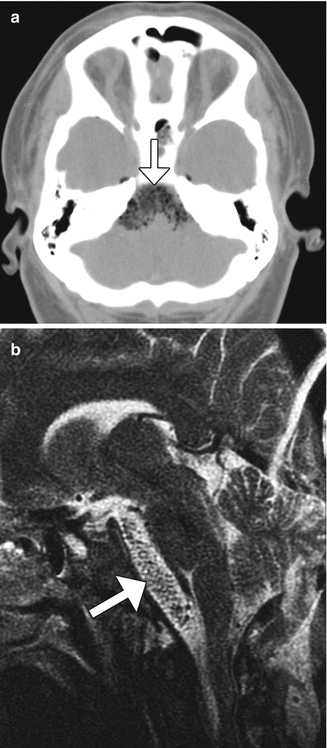
Fig. 7.29
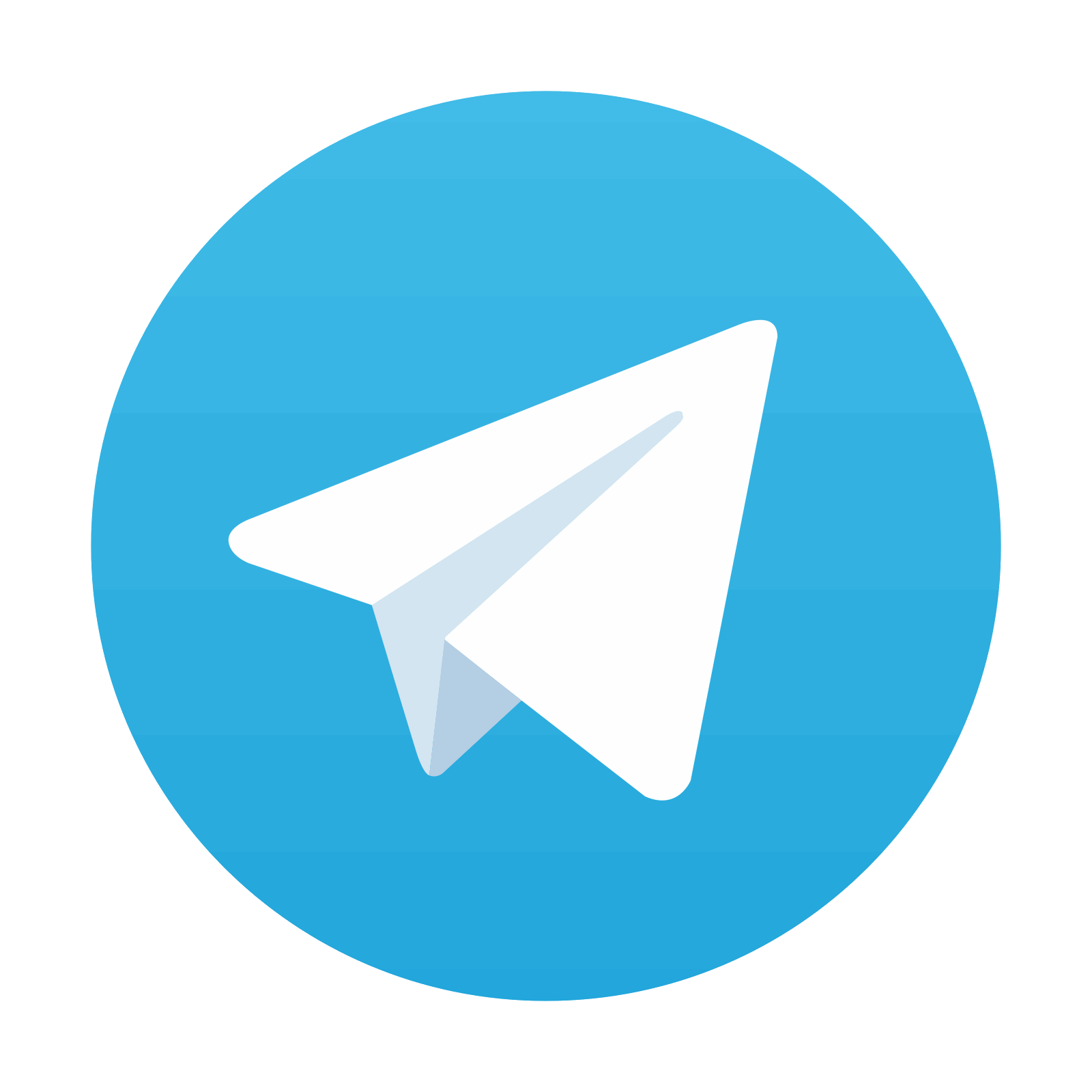
Merocel migration and brainstem compression. Axial CT image (a) shows low-intensity sponge-like material posterior to the sella that compresses the brainstem (arrow). Similarly, the sagittal T2-weighted MRI (b) shows the spongy hypointense packing material extending posteriorly, exerting mild mass effect upon the brainstem (arrow)
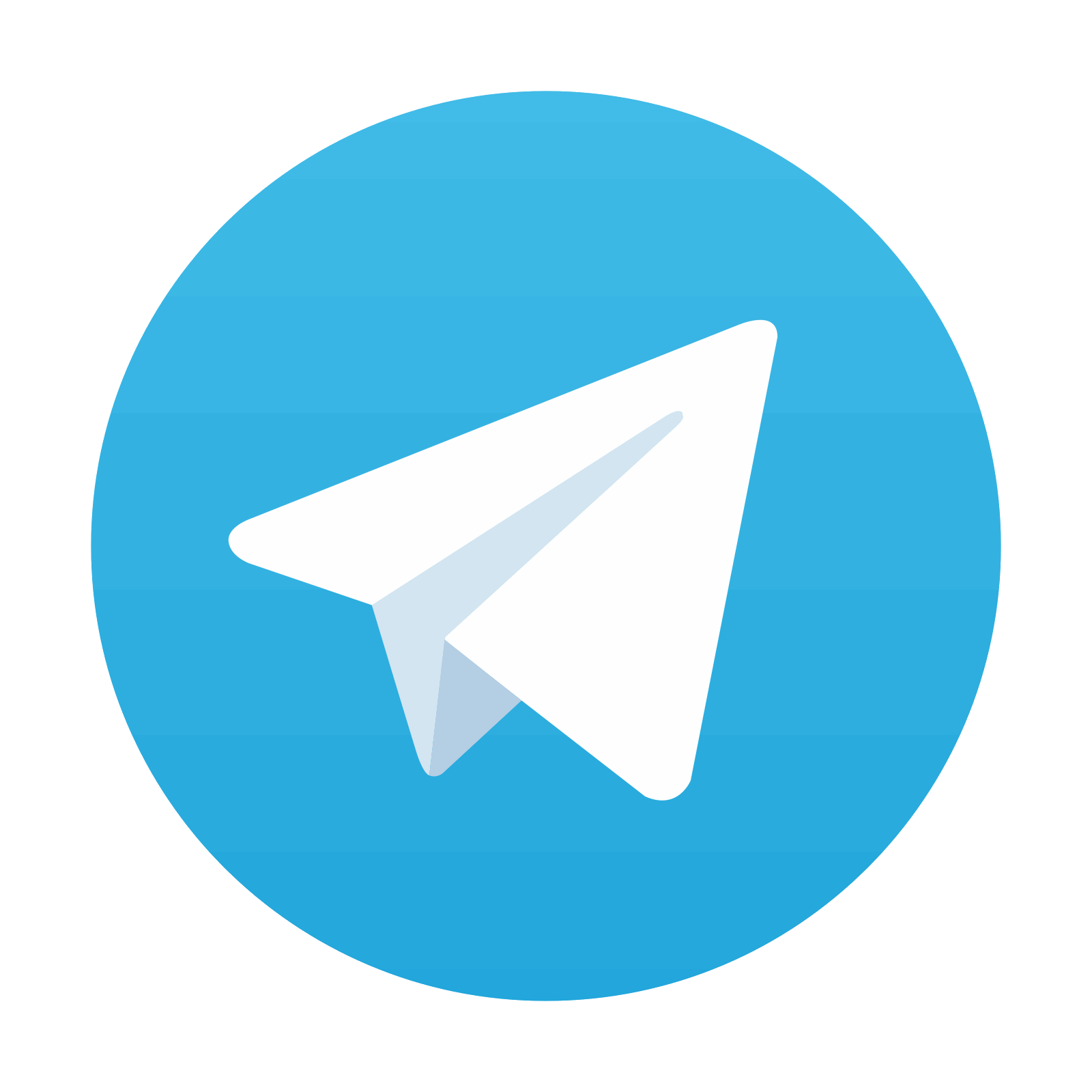
Stay updated, free articles. Join our Telegram channel

Full access? Get Clinical Tree
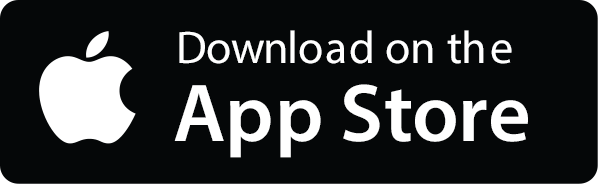
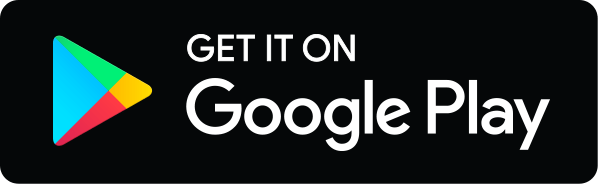
