CHAPTER 11 INTERVENTIONAL RADIOLOGY
Instruments and Tools of the Trade
Diagnostic Percutaneous Arteriography
Hemodialysis Access Interventions
Percutaneous Biliary Drainage and Stenting
Interventional radiology (IR) is a diverse practice of patient care using minimally invasive, imaging guided procedures to diagnose and treat disease nonoperatively. Percutaneous diagnostic and therapeutic procedures are performed using fluoroscopy, ultrasound, computed tomography (CT), or magnetic resonance (MR) imaging for guidance. These procedures, which may be categorized as vascular (i.e., arteriography, venography) and nonvascular (e.g., decompression and drainage of obstructed kidneys and bile ducts), are performed in an IR suite and are often done on an outpatient basis. Many procedures that were previously performed surgically are now accomplished by an interventional radiologist with less morbidity and a shorter hospital stay.
Since 1958, when Dr. Seldinger described a method of percutaneous vascular access using a hollow core needle, guidewire, and catheter, IR has continued to evolve, as new techniques and devices are developed to enhance patient care. Technical advances have led to significant improvements in patient safety and procedural diversity. As these rapid changes in endovascular technologies continue to expand, so will the possibilities of image-guided, minimally invasive procedures.
Because IR is procedural, interventional radiologists tend to be more involved in patient care. Referred patients are routinely worked up by the IR service and are subsequently followed up postprocedure. The preprocedure workup consists of patient assessment as well as evaluation of previous imaging studies (Table 11.1). Postprocedure follow-up is essential to determine whether the procedure has been successful and free of complications. This all-inclusive clinical service underlines that there is more to IR than simply doing procedures. Many IR practices offer an active inpatient and outpatient consult service and also employ specially trained nurse practitioners and physician’s assistants as physician extenders. Because procedures performed by interventional radiologists are invasive, there are associated risks for developing complications. It is important that the patient be aware of these risks so that an informed decision can be made by weighing the possible risks of a procedure against its benefits. A physician should never place a patient in a position of risk unless the risks, benefits, and alternatives of the planned procedure have been discussed, understood, and consented to before the procedure. It is in the physician’s best interest to be honest and forthright when dealing with patients and their expectations about the outcomes of a procedure.
The aim of this chapter is to explain the background, indications, and basic techniques of the procedures commonly performed in IR so that the reader will gain an understanding of how IR contributes to patient care.
INSTRUMENTS AND TOOLS OF THE TRADE
Interventional radiologic procedures are performed in imaging suites with fluoroscopy and digital subtraction angiography. Ultrasound, CT, and MR imaging are also utilized by the interventional radiologist.
Each angiography/interventional suite is staffed by the operating radiologist and at least one assistant. A radiology technologist provides imaging expertise and a nurse provides patient sedation, monitors vital signs, and attends to patient needs during the procedure.
Endoluminal procedures require administration of a contrast agent. Nonionic iodinated contrast is most frequently used to delineate, radiographically, the lumen of an artery, vein, and biliary duct, gastrointestinal (GI) or urinary tract. Carbon dioxide or gadolinium can be used in patients with renal insufficiency or allergy to radiographic contrast agents.
INTERVENTIONAL RADIOLOGY PREPROCEDURE CHECKLIST
Indication for procedure/question(s) to be answered from procedure
Contraindications for procedure
Review prior imaging and noninvasive studies
Check for contrast allergy
Written informed consent
Check coagulation parameters and serum creatinine
Need for prophylactic antibiotics
Patient should be fasting and well hydrated
Discontinue heparin infusion
There is a variety of commercially available catheters, sheaths, guidewires, balloon catheters, vascular stents, and caval filters. Familiarity with these instruments requires significant training and experience. There are numerous preformed shapes and types of angiographic catheters, most of which are made from flexible plastic material such as polyethylene or polyurethane. Wire braiding may be incorporated into the catheter shaft to increase stiffness and improve its torque. Catheter diameters are measured in French (F) size, where 3F = 1.0 mm (outside diameter). Most angiographic catheters are in the 4F to 7F range. Aortic angiography is performed with pigtail catheters that have several side holes proximal to the tip allowing rapid flow of a contrast bolus while the pigtail loop stabilizes the catheter preventing recoil (Fig. 11.1A, B). Selective angiography (renal, celiac, and superior mesenteric arteries) is performed with a curved end-hole catheter such as a Cobra C2 (Fig. 11.1C). A variety of catheters and guidewires may be necessary during a procedure, and placement of a vascular sheath with a hemostatic valve at the site of access reduces vessel trauma and facilitates rapid catheter and guidewire exchange.
Catheters used in the drainage of abscesses, obstructed kidneys (percutaneous nephrostomy), and bile ducts are made of polyurethane and are of greater diameter than angiographic catheters (8F to 12F). These drainage catheters are usually placed using the Seldinger technique after which they are secured in position by deploying a locking pigtail mechanism formed by pulling on a suture that runs in most of the catheter shaft and is attached to its tip. The pigtail loop itself contains large side holes for drainage. The smaller diameter catheters occlude more easily with debris and should be routinely changed over a guidewire every 6 to 8 weeks when continued drainage is required.
FIGURE 11.1 A–F: Tools of the trade. A: Pigtail catheter. B: Angled pigtail catheter. C: Cobra catheter. D: J-tipped guidewire. E: Straight (Bentson) guidewire. F: An 18-gauge needle for vessel puncture.
Guidewires increase the ease and safety of catheter placement. The outer shell of a guidewire consists of a very tightly wound but flexible metal spring coil. A stiff central core provides rigidity over a variable length of the guidewire. The balance between these two components dictates the handling characteristics of the guidewire. For example, the distal 15 cm of a Bentson guidewire is floppy, allowing easy coiling (Fig. 11.1E). A J-tipped guidewire reduces the risk of damaging the vessel wall because of its blunt tip (Fig. 11.1D). Guidewires usually range in diameter from 18 thousandths of an inch (0.018 in.) to 38 thousandths of an inch (0.038 in.). The standard length for most wires is 145 cm, whereas longer guidewires (260 cm) are available to facilitate catheter exchange.
Needles used in arteriography range in size from a 21-gauge needle through which a 0.018-in. guidewire will pass to an 18-gauge needle that accepts a 0.035-in. guidewire (Fig. 11.1F).
Angiography is a technique of imaging blood vessels, usually by injecting contrast material via an intraluminally placed catheter. Blood vessels may also be visualized noninvasively using computed tomography angiography (CTA) or magnetic resonance angiography (MRA), which takes advantage of the inherent contrast between flowing blood and stationary tissue.
Diagnostic Percutaneous Arteriography
Diagnostic arteriography begins by catheterizing an artery using Seldinger technique (Fig. 11.2). This technique of vascular catheterization was initially described by a radiologist, Sven Seldinger. After placing a hollow core needle into an artery (femoral or brachial), a guidewire is inserted through the needle and into the artery. Sonographic and fluoroscopic guidance is often necessary. The needle is exchanged for a vascular catheter or vascular introducing sheath. Subsequent catheter movement and exchange is performed over a guidewire. Large vessel arteriography is performed using flush catheters (pigtail). Smaller arteries are selectively cannulated using catheters of various shapes and sizes. Microcatheters are used for sub- or superselective arteriography.
After the catheter is safely positioned in the artery of choice, the guidewire is removed and a contrast agent injected through the catheter during image acquisition. Mask images obtained before injection of contrast allow for subtraction of nonvascular structures. Many images are acquired as the contrast agent flows through the lumen of the vessel producing the arteriogram. The catheter can be exchanged or repositioned for additional imaging. After completion of the procedure, the catheter is removed and hemostasis obtained at the arteriotomy site using manual compression or a percutaneous closure device. Recovery time for the patient is 2 to 6 hours.
FIGURE 11.2 A–E: Seldinger technique. A: The vessel is punctured with the needle. B: A guidewire is advanced through the needle into the vessel. C: The needle is removed leaving the guidewire in place. D: A catheter is advanced over the guidewire into the vessel. E: The guidewire is removed and the catheter flushed.
Noninvasive angiography (MRA/CTA) is replacing most percutaneous diagnostic arteriography, except where intervention is expected or other examinations are indefinite. Pulmonary arteriography performed by venous catheterization and crossing the right side of the heart has largely been replaced by CTA, which has an acceptably high specificity and sensitivity. Both CTA and MRA are widely used in the evaluation of aortic, visceral, renal, and peripheral arterial disease (PAD). However, the administration of gadolinium chelates, commonly used in MR angiography, is associated with a higher incidence of nephrogenic systemic sclerosis in patients with renal impairment.
Generally, the diagnosis of PAD, or peripheral vascular disease, has already been made by the time an arteriogram is requested. The evaluation includes an assessment of the patient’s symptoms, a physical examination, and a review of the noninvasive imaging tests, such as CT, MR, duplex ultrasonography, and segmental limb pressures before proceeding to angiography. Rather than being an end point, the angiogram helps formulate a comprehensive plan in the patient’s subsequent treatment as it evaluates the extent and severity of disease and provides a road map for intervention (balloon angioplasty, stenting, surgery, etc.). Patients with diabetes may present with a more advanced stage of ischemia as they are prone to developing peripheral neuropathy that may mask the above symptoms. They also tend to have a greater prevalence of small vessel disease, which is more difficult to treat surgically and contributes to a less favorable long-term prognosis compared to other causes of PAD.
Arteriographic examination of patients with PAD may be divided into three anatomic regions: aortoiliac, infrainguinal, and infrageniculate. Aortic aneurysms occur most commonly below the level of the renal arteries. The number of renal arteries should also be noted, as should the presence of stenoses in these vessels. Bilateral oblique views of the pelvis should be obtained during the arteriogram, as hemodynamically significant stenoses can be missed if only a frontal view is performed.
In general, arterial stenoses are not regarded as significant unless they reduce the lumen diameter by 50% angiographically. Measuring a pressure gradient across it can more accurately assess the significance of a stenosis. The presence of a 10 mm Hg gradient or greater across a stenosis is regarded as significant and worthy of further treatment such as angioplasty or stenting. If the gradient is less than 10 mm Hg, a vasodilator such as nitroglycerin may be given intra-arterially to simulate exercise and possibly unmask a significant stenosis. Most endovascular interventions are performed in the carotid, renal, aortoiliac, and femoropopliteal arteries.
In the absence of satisfactory femoral pulses bilaterally, either the brachial or the axillary arteries can be used for percutaneous access.
Diagnostic venography is performed in all extremities, as well as centrally, for surgical planning or evaluation of deep venous thrombosis when vascular ultrasound is indeterminate. Most venography is performed in conjunction with interventional procedures (placement of caval filters and central venous catheters, renal vein sampling).
Thrombolysis is the process of dissolving blood clot in order to establish patency of an occluded (thrombosed) vessel, using drugs such as urokinase and tissue plasminogen activator (t-PA). These drugs are infused directly into the thrombosed grafts and vessels to ensure a very high local concentration of the drug. Active internal bleeding, recent intracranial hemorrhage, or surgery is absolute contraindication for thrombolysis (Table 11.2).
Complications of thrombolysis include bleeding and distal embolization of thrombus. The cumulative probability of major complications increases with duration of infusion, rising from less than 10% after 16 hours to more than 30% at 40 hours. Once thrombolysis is complete, balloon angioplasty or surgery can be used to treat any underlying vessel stenoses that contributed to the occlusion.
CONTRAINDICATIONS FOR ARTERIAL THROMBOLYSIS
Absolute
Active gastrointestinal (GI) or genitourinary (GU) bleeding
Recent (<12 months) cerebral hemorrhage/infarct/surgery
Irreversible limb ischemia
Relative
History of GI or GU bleeding
Recent thoracic/abdominal surgery
Recent trauma
Severe uncontrolled hypertension
Balloon Angioplasty
Percutaneous transluminal balloon angioplasty (PTA) has become an established technique in the treatment of vascular stenoses due to atherosclerotic plaque and fibromuscular dysplasia. The precise pathophysiologic mechanism of PTA in atherosclerotic plaque is controversial. However, most agree that PTA results in a controlled plaque and intimal fracture with localized dissection into the underlying media thereby increasing the intraluminal diameter. The plaque, intima, and media are subsequently remodeled to give a smoother endoluminal surface. The appropriate angioplasty balloon catheter should be chosen so that its inflated diameter is the same size or slightly larger than the adjacent nondiseased vessel. Initially, the stenosis is crossed with a guidewire that is left across the lesion until the procedure is finished. Heparin and nitroglycerin may be given intra-arterially to prevent thrombosis and vessel spasm, respectively. The angioplasty balloon is advanced across the stenosis, inflated, and deflated slowly under fluoroscopic guidance. Repeat angiography and pressure measurements should be obtained to evaluate the results of angioplasty. Suboptimal angioplasty results may require placement of an endovascular stent.
Iliac artery angioplasty improves inflow to the lower limb and requires balloons that are 7 to 10 mm in diameter. Again, a guidewire is left across the stenosis during the procedure, the success of which is judged on angiographic and hemodynamic criteria. Stent placement should be considered if the postangioplasty pressure gradient is greater than 10 mm Hg, there is residual stenosis of greater than 30%, or if a flow-limiting dissection is present (Fig. 11.3). Simultaneous PTA of both common iliac arteries, known as the kissing-balloons technique, is effective in treating bilateral proximal common iliac artery stenoses.
Infrainguinal angioplasty (superficial femoral and popliteal arteries) is gaining clinical acceptance as patency outcomes for PTA and stenting rivals outcomes of surgical bypass procedures (Fig. 11.4A, B).
Infrageniculate angioplasty (anterior/posterior tibial and peroneal arteries) is usually performed for limb salvage or to reduce the extent of an impending below-the-knee or forefoot amputation for ischemia. This technique requires a fine diameter guidewire (0.010″ to 0.018″) and angioplasty balloon (2 to 3 mm in diameter) because of the smaller vessel size.
Renal artery angioplasty is usually performed with a 5- to 7-mm-diameter balloon. Atheromatous disease usually involves the proximal or ostial portion of the vessel in contrast to fibromuscular dysplasia that usually affects the midportion of the vessel. The improvement in renal function and hypertension following renal artery angioplasty is equivalent to that obtained after surgical revascularization (Fig. 11.5). Renal artery stenting is performed if there is a residual stenosis or significant dissection postangioplasty (Fig. 11.6). Ostial renal artery stenoses are often stented primarily, without balloon predilatation.
FIGURE 11.3 A–G: Thrombolysis, balloon angioplasty, and stenting of a common iliac artery occlusion. A: Aortogram/pelvic angiogram shows occlusion of the right common iliac artery (arrow). B: Partial recanalization of the right common iliac artery following thrombolysis performed via an infusion catheter (arrow). C: Balloon angioplasty was performed showing residual narrowing of the balloon (arrow). D: The common iliac stenosis persisted postangioplasty (arrow). E, F: A balloon expandable stent was deployed across the stenosis. The undeployed stent (arrow) can be seen on the distal portion of the angioplasty balloon. G: Poststenting, no residual stenosis is present.
FIGURE 11.4 A, B:Balloon angioplasty. This left knee angiogram shows tandem stenoses (arrows) and multiple collateral formation in the popliteal artery. After angioplasty of the stenoses with a 3 mm × 2 cm balloon there is improved flow in the popliteal artery.
FIGURE 11.5 A, B: Renal artery angioplasty. A: Flush aortogram showing right renal artery stenosis (curved arrow). B: Residual stenosis persists postballoon angioplasty. Note that the guidewire (arrow) is left across the stenosis.
FIGURE 11.6 Renal artery stenting. A Palmaz stent (arrow) has been placed across a left renal artery stenosis.
FIGURE 11.7 Palmaz stent mounted on an angioplasty balloon and in its expanded form. (Photograph courtesy of Cordis Corporation.)
Peripheral Endovascular Stents
There are two main indications for endovascular stent placement: (a) a residual pressure gradient of more than 10 mm Hg postangioplasty, which is regarded as an indication for either repeat angioplasty or stent placement; and (b) postangioplasty flow-limiting dissection, in which the goal of stent placement is to appose the dissected flap against the wall and improve flow. The balloon–stent combination is placed across the stenosis and the balloon is inflated, thus opening and deploying the stent. The balloon is then deflated and removed (Fig. 11.7). There are two general types of metallic endovascular stents, balloon-expandable and self-expanding. Deployment of the balloon-expandable stent is described above. Deployment of the self-expanding stent, which does not require delivery on an angioplasty balloon, involves withdrawal of a covering sheath, after which the stent expands. Postdilatation with an angioplasty balloon may be necessary. Self-expanding stents are usually more flexible than balloon-mounted stents, which is an advantage when stenting tortuous vessels (Fig. 11.8). Covered (polytetrafluoroethylene (PTFE), Dacron) stents are available for treatment of vascular injury resulting in pseudoaneurysm, hemorrhage, or arteriovenous fistula. Drug eluting stents are currently being investigated to determine efficacy in treatment of arterial stenosis. These stents are designed to reduce neointimal hyperplasia which develops and covers the inside of the stent.
Aortic Stent Grafts
Stent grafts have revolutionized the treatment of abdominal aortic aneurysms (AAA), reducing the severity and duration of the procedure and postprocedure morbidity and hospital stay. Ninety percent of patients are discharged within 48 hours of the procedure, which is usually done in the operating room or combined operative/fluoroscopy suite, under general or epidural anesthesia. Through bilateral femoral artery surgical cutdowns, the various components of the aortic stent graft are introduced and deployed in the abdominal aorta and iliac arteries, under fluoroscopic guidance. A preprocedure CT scan of the aorta is essential for precise vessel measurement and localization of arterial branches. The device, which is composed of woven polyester on a wire exoskeleton frame, is placed in the infrarenal aorta and extends down to the common or external iliac arteries (Fig. 11.9). Postprocedure follow-up with CT scans every 6 months is important for the detection of an endoleak, which is a leak into the aneurysm sac. In the absence of such an endoleak, the aneurysm sac should reduce in size (Fig. 11.10A–D).
FIGURE 11.8 The Wallstent is self-expanding and flexible. (Photograph courtesy of Boston Scientific.)
FIGURE 11.9 Aortic stent graft device is composed of three components—a main body and two iliac extensions, which can be custom made to suit each patient. The components are placed through femoral artery cutdowns (Courtesy of Cook Medical, Inc.)
FIGURE 11.10 A–D:Aortic Endograft Follow-up. Serial CT studies show an infrarenal abdominal aortic aneurysm (AAA) measuring 4.5 cm in diameter (A). Six months post-AAA endograft placement, the excluded aneurysm sac now measures 4.0 cm (B) and at 18 months postendograft, it has further reduced to a diameter of 3.2 cm (C). CT abdomen in a patient post-AAA endograft shows contrast within the aneurysm sac but outside the endograft (arrow) (D). This is an endoleak which occurs because of persistent blood flow into the aneurysm which in this patient is due to retrograde flow from a lumbar artery.
Complications (Table 11.3)
Complications of diagnostic angiography are infrequent, with access site vascular injury and hematoma comprising the overwhelming majority. Risk factors for this complication include hypertension and obesity. Prevention involves meticulous technique including vessel puncture over the femoral head and constant manual pressure directly over the puncture site after removal of the catheter until hemostasis is achieved. The hematoma may extend into the retroperitoneum when the puncture site is above the inguinal ligament (Fig. 11.11A). Incomplete or intermittent compression over the puncture site may also result in the formation of a pseudoaneurysm (Fig. 11.11B). Dissection of vessel wall may occur if the needle/wire or catheter is introduced subinitmally (Fig. 11.11C), and distal embolization of mural plaque or thrombus is a risk following any endovascular manipulation (Fig. 11.11D). Complications following brachial or axillary arterial puncture are more common than with femoral artery puncture because of the smaller vessel size and the close proximity of the vessels to nerves within a common sheath in the arm. Dissection, thrombosis, or pseudoaneurysm of the access artery may require endovascular intervention or surgical repair.
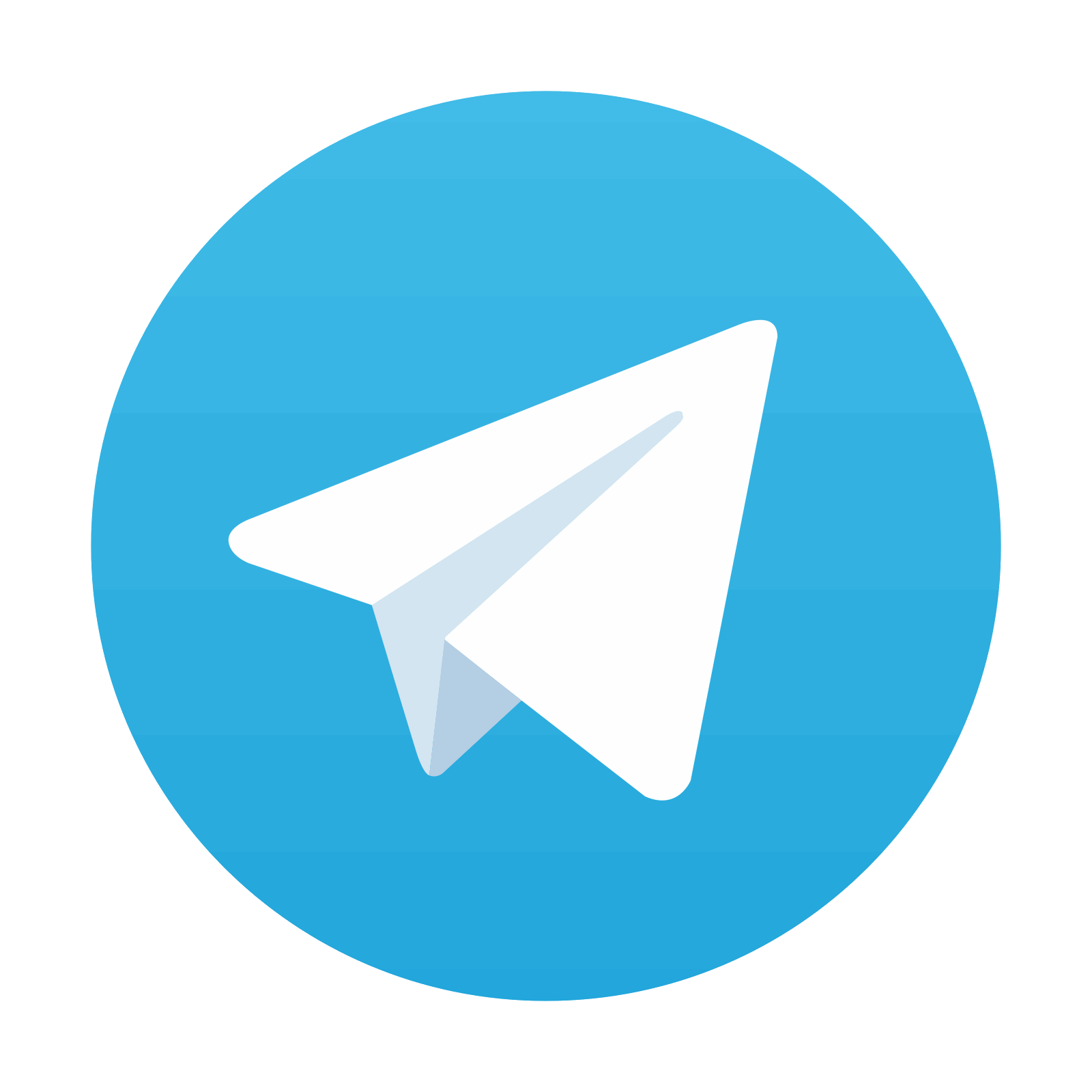
Stay updated, free articles. Join our Telegram channel

Full access? Get Clinical Tree
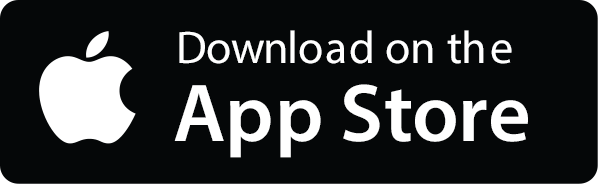
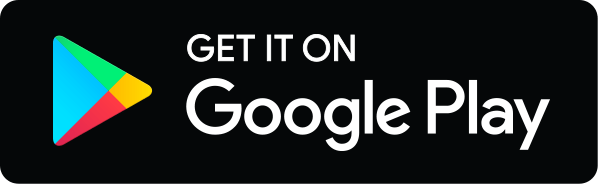