Interventional Ultrasound: Introduction and Historical Background
1.1 The Vienna Congress
The “First World Congress on Ultrasonic Diagnostics in Medicine” was held in Vienna in 1969. It marked the end of the pioneering age of diagnostic ultrasound that began after World War II. By that time ultrasonography had already found applications in ophthalmology as well as neurology (echoencephalography using A-mode ultrasound to detect intracranial hemorrhage, for example) and cardiology (TM-mode echocardiography for diagnosing valvular disease). Especially for the fields of obstetrics, gynecology, and internal medicine, the Congress marked the start of a burgeoning period of clinical research.
The Vienna Congress began as the Third International Symposium on Ultrasonic Diagnostics in Ophthalmology (SIDUO III) headed by Viennese ophthalmologists J. Böck, who served as president, and K. Ossoinig, who served as scientific secretary. The original symposium was changed to an interdisciplinary world conference when it was recognized that, for the first time, comprehensive reports on diagnostic ultrasound were available from a number of other specialties. A total of 190 papers were presented—48 in ophthalmology, 29 in neurology, 20 in cardiology, 19 in obstetrics and gynecology, and 23 in internal medicine.
Only two papers dealt with the capabilities of ultrasound-guided needle procedures, and both reflected the full spectrum of possible procedures and future diagnostic applications. A. Kratochwil1 reported on ultrasound-guided amniocentesis using a specially designed biopsy transducer (▶ Fig. 1.1).
Fig. 1.1 A-mode transducer with a central aperture for guiding the (amniocentesis) needle, introduced by Kratochwil in Vienna in 1969. (Source: With kind permission of the Vienna Medical Academy.1)
U. W. Blauenstein used ultrasound to localize tumor nodules in the liver and puncture them percutaneously, aided by markings drawn on the skin (▶ Fig. 1.2).2
Fig. 1.2 Example of an ultrasound-guided percutaneous liver biopsy. Same-scale views of the skin site and ultrasound image as presented by Blauenstein in Vienna in 1969. X marks the skin entry site over the enlarged hepatic left lobe. (Source: With kind permission of the Vienna Medical Academy.2)
To appreciate the significance of these reports, we must recall the conventional diagnostic tools that were available at that time. The relatively simple percutaneous drainage of relatively large fluid collections was traditionally guided by anatomical landmarks (e.g., for draining ascites) or percussion (e.g., for thoracentesis). But these methods were difficult or impossible to use in patients with small fluid collections, adhesions, or septations. A relatively large organ like the liver could be sampled to investigate presumed diffuse disease using a standard technique following localization by percussion. The development of the Menghini needle in 1958 had made percutaneous liver biopsy a simple procedure.3 On the other hand, a suspected tumor was considered at least a relative contraindication to needle biopsy, so that technique could not be used for the selective sampling of tumor nodules in the liver.
The only imaging modality available at that time for the percutaneous biopsy of nonpalpable organs and structures was classical radiography. But this method was problematic for several reasons. First, the relationship of the intended target (e.g., the inferior renal pole) to anatomical landmarks like the spinal column was measurable but the distance from skin to target (i.e., the second plane) was not. Moreover, the kidney had to have sufficient residual function that it could be opacified by intravenous contrast medium. Radiographic imaging also required costly radiation safety measures for the patient and staff.
Kratochwil described in his paper how ultrasound guidance could make standard needle procedures easier and safer (here, by avoiding the placenta), even in unusual situations. The sectional images produced by ultrasonography enable the target site to be visualized and localized in all three dimensions. As early as 1961, G. M. Berlyne4 suggested that the two-dimensional pyelogram obtained before percutaneous renal biopsy could be supplemented by an A-mode scan to measure the depth from skin to target. In 1970, G. Rettenmaier noted the advantages of using two-dimensional real-time ultrasound to track the respiratory movements of the kidney and provide a third localizing dimension.5
Martin and Ellis6 were the first authors to report on the aspiration biopsy of suspected neoplasms. Traditionally, however, a targeted aspiration biopsy could be used only for palpable masses, thoracic tumors accessible to radiographic guidance, or during (exploratory) laparotomy.
Blauenstein was the first author to show that ultrasound could be used not only to identify suspicious lesions in organs but also to biopsy them selectively for further differentiation. Thus began the development of a relatively simple diagnostic method for the detection and differentiation of even small, nonpalpable suspected neoplasms. By that time authors had already reported positive experience with the cytologic analysis of material sampled by percutaneous aspiration (e.g., from lymph nodes, the thyroid gland, the breast, or lung tumors) and of tumor lesions (e.g., of the pancreas) sampled by intraoperative needle biopsy.
Meanwhile, both Kratochwil and Blauenstein had demonstrated the two main options available for performing ultrasound-guided punctures. Blauenstein used a kind of freehand technique in which he first measured the location of the intended target and marked it on the skin, then advanced the needle to the target without observing it directly (▶ Fig. 1.2). Kratochwil used a special biopsy transducer (▶ Fig. 1.1), which provided an A-mode display of the structures (in this case the placenta) previously defined by a slow B-mode scan.
1.2 The Introduction of Ultrasound into Routine Clinical Use
1.2.1 The Evolution of Ultrasound Imaging Techniques
Over the next 10 to 15 years, two-dimensional sectional imaging with ultrasound was increasingly implemented in almost all medical specialties. At first the bistable compound scan was still the (international) standard and was supplemented by A-mode scanning for “structural” analysis. The introduction of gray-scale technology in 1974 significantly improved the images generated by this type of system. Even so, the slow compound scan was increasingly superseded by fast (real-time) B-mode scanning, which replaced it completely by about 1980. As early as 1965, R. Soldner of the Siemens company7 had developed the Vidoson 635 as the first mechanical fast B-mode scanner. This device was widely used starting in 1970 and became especially popular in internal medicine and obstetrics. An electronic linear-array scanner was produced by ADR in 1974. By 1980 at the latest, all major ultrasound companies had developed mechanical or electronic real-time scanners. Real-time B-mode imaging was the technical prerequisite for the increasingly rapid implementation of diagnostic ultrasound during this period.
Some more theoretically oriented scientists believed that technical advances in ultrasonography would make it possible to discriminate among different tissue types, thus enabling a reliable benign–malignant differentiation. As a result, “tissue characterization” became a key topic of discussion at international conferences, which were now being held at regular intervals. Meanwhile, further technical advances in ultrasound-guided biopsy were pursued initially at centers of diagnostic ultrasound. Clinical ultrasound specialists favored that modality as a simple, low-risk method for establishing a morphologic diagnosis. Hans Henrick Holm stated this viewpoint succinctly in his book, Interventional Ultrasound8:
When considering the difficulties frequently encountered with conventional microscopy, it does not seem likely that tissue characterization with ultrasound or with any other imaging modality will make it possible, with clinically acceptable accuracy, e.g. routinely to distinguish between a benign and a malignant intra-abdominal lesion.
1.2.2 Technical Evolution of Ultrasound-Guided Biopsies
From a technical standpoint, Kratochwil’s concept of using a single transducer with a central aperture on a compound scanner was easily moved from the design stage to practical reality (▶ Fig. 1.3). Biopsy transducers for compound scanners became available commercially only a short time after the Vienna Congress.9,10 These devices were used in many early studies.
Fig. 1.3 Commercially available biopsy transducer (A-scan) with a central needle aperture, mounted on a compound scanner arm circa 1972.
On the other hand, this technique was still somewhat laborious for percutaneous biopsies: First, an image of the intended target was acquired slowly with a bistable compound scanner (this took at least several seconds), and the image was stored in memory. After the desired puncture site had been marked on the skin, a sterilized biopsy transducer was fastened to the scanner arm and applied to the marked skin site. Next, the biopsy transducer was swept through a sector arc to redefine the desired plane. The only orientation guide available beyond this point was the static (frozen) B-mode image. The target and needle path could be directly observed during the biopsy itself only by viewing an A-mode display (▶ Fig. 1.4).
Fig. 1.4 Biopsy technique with a compound scanner.
a The intended target is displayed on a storage cathode-ray tube with a slow B-mode scan.
b The transducer is replaced with a (sterile) biopsy transducer (schematic illustration) (▶ Fig. 1.3).
c The intended target is again visualized in a sector-shaped scan (3 = needle tip).
d Direct observation of the biopsy needle in A-mode (← signal of the needle tip).
Needle insertion with the fast B-mode Vidoson system was done using freehand technique: The intended target was first defined in the B-mode image. The most favorable entry site in the second plane was located and marked on the skin by noting the acoustic shadow cast by a small wooden stick placed between the skin and transducer (▶ Fig. 1.5). The depth from skin to target was measured on the monitor screen and could be preset on the needle with a set screw. Whenever possible, the cumbersome (transducer placed in a water bag!) Vidoson was positioned next to the entry site so that the intended target and needle could be monitored directly in the B-mode image.11 Other authors used a needle guide mounted on the side of the Vidoson. This design was available as a prototype developed by Siemens in about 1974,12 or users could create their own homemade version13 (▶ Fig. 1.6).
Fig. 1.5 Needle guidance with fast B-mode ultrasound (Siemens Vidoson). A small wooden stick placed between the transducer and skin (a) casts an acoustic shadow (b), which provides orientation in the second plane. The depth from skin to target is measured in the image. (Source: With kind permission from Springer Science+Business Media B.V.77)
Fig. 1.6
a Vidoson (fast B-mode) with needle guide.
b Needle echo in a renal cyst (↑). (Source: Presented by Afschrift at the World Congress of Ultrasound in San Francisco in 1975. With kind permission from Springer Science+Business Media B.V.13)
This principle was adopted in the easier-to-use handy sector scanners (with direct contact to the skin) that were developed later. In 1978, Saitoh and Watanabe worked with the Aloka company to develop an adapter for a sector scanner (▶ Fig. 1.7),14 which was then marketed commercially. At about the same time, Kretz Ultrasound also developed an adapter for their Combison 100, which was widely used in Europe at that time.
Fig. 1.7 Transducer with side-mounted needle holder and guide manufactured by Aloka. Developed in Japan by Saitho in 1978.14
A relatively simple, homemade linear array transducer with a central aperture (▶ Fig. 1.8) was first used by H. H. Holm in 1974 for applications such as amniocentesis. Under favorable conditions, the needle could be identified in the image. In 1976, Pedersen introduced the alternative design of a linear array device with a side-mounted needle guide,15 which featured an adjustable needle angle (▶ Fig. 1.9). In 1980 Toshiba developed a special biopsy transducer with a triangular needle channel (▶ Fig. 1.10) for its SAL 20 linear array scanner. The channel was open on one side for introducing the needle guide and needle and allowed the needle to be inserted at an oblique angle to the ultrasound beam.16 This principle was advanced further by the development of disposable, plastic needle guides. The transducer could be placed within a sterile sheath and the sterile needle guide could be introduced from the side. In both systems, the needle path predefined by the needle guide was displayed in the image as an electronic line (▶ Fig. 1.11).
Fig. 1.8 Simple electronic linear array scanner with a central aperture for a biopsy needle, “homemade” in the ultrasound laboratory at Gentofte Hospital (H. H. Holm; first used in 1974).
Fig. 1.9
a Electronic linear array scanner with side-mounted needle guide, “homemade” in the ultrasound laboratory at Gentofte Hospital.
b Original image obtained during amniocentesis, and corresponding drawing. The arrows indicate the needle echoes.
Fig. 1.10 Linear array transducer. Prototype of the Toshiba biopsy transducer made for the SAL 20, first available in 1978.
Fig. 1.11 Nephropyelostomy performed with a mechanical sector scanner and lateral needle guide. The needle path is marked electronically in the image. The needle tip (←) is clearly visible inside the cyst.
As special biopsy transducers were being developed and used, many examiners continued to use freehand technique for percutaneous biopsies (and many still do so today). This was facilitated by the development of smaller, lighter transducers that could be placed next to the puncture site. The needle could be observed as clearly as with a side-mounted adapter.17 Freehand technique also simplified hygiene (transducers did not always have to be sterilized or placed in a sterile wrapper), and it was often possible to use shorter, less expensive needles.
Initially, material for cytologic analysis was aspirated from solid tumors with thin (≤0.7 mm), flexible Chiba-type needles using the technique described by Franzen.18 A special syringe holder (Cameco, Sweden) was often used that allowed aspirations to be performed with one hand. Scandinavian authors in particular reported positive early experience with the cytologic analysis of well-preserved aspirated cells.19–22 The basic equivalence between aspiration cytology and histologic biopsy had already been demonstrated in earlier studies, such as the study on liver biopsies published by Sheila Sherlock in 1967.23 Later comparative studies confirmed these initial results, even showing a slight superiority of aspiration cytology with regard to the rate of nondiagnostic biopsies, for example.24
As the ultrasound-guided aspiration of solid tumors became more widely practiced, there was also a growing desire to obtain thin-needle core tissue samples for histologic examination. This desire arose mainly from pathologists, who generally preferred to work with histologic material or at least found it easier to identify malignant tumor types from histologic samples. Isler et al subsequently introduced a 22 gauge cutting needle in 1981.25 The following year a commercially available modification of the Menghini needle called the Surecut needle26
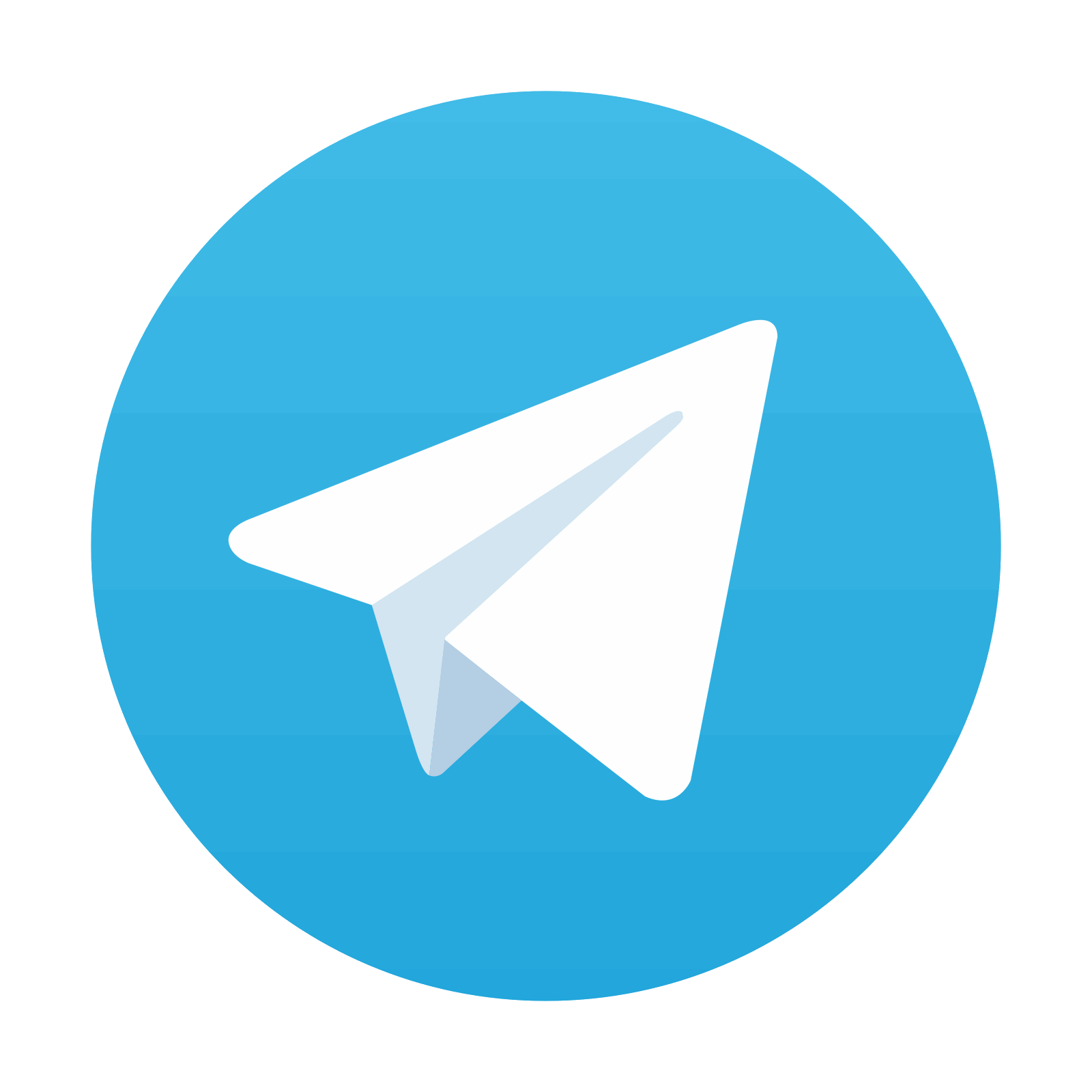
Stay updated, free articles. Join our Telegram channel

Full access? Get Clinical Tree
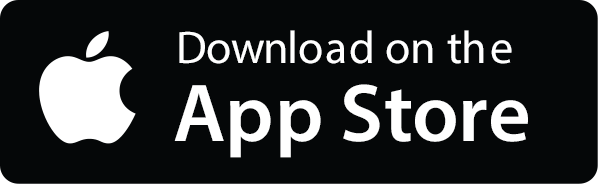
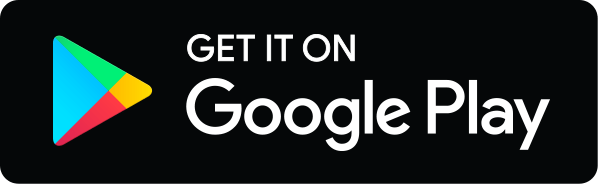