Ferenc A. Jolesz (ed.)Intraoperative Imaging and Image-Guided Therapy201410.1007/978-1-4614-7657-3_52
© Springer Science+Business Media New York 2014
52. Intraoperative Imaging in Cardiac Surgery
(1)
Vanderbilt Heart & Vascular Institute, Nashville, TN, USA
(2)
Department of Cardiac Surgery, Vanderbilt University Medical Center, 1215 21st Avenue South, Nashville, TN 37232-8802, USA
Abstract
Coronary artery bypass surgery is one of the most common surgical procedures in the USA and worldwide. The long-term outcomes of the procedure, such as survival, freedom from angina, and quality of life, are determined by graft patency and completeness of revascularization. On average, from 20 to 30 % of all grafts are no longer patent at 12 months after the surgery. From 3 to 12 % of grafts fail immediately or in the early postoperative period. To a great extent, the long-term success of the surgery is dependent on intraoperative graft patency evaluation, with detection and subsequent revision of failed grafts in a timely manner. A number of techniques have been developed for intraoperative graft assessment. Currently coronary angiography, intraoperative fluorescent imaging, and transit time flow measurement are utilized for this purpose. The last two techniques are most commonly used as they are relatively efficient and noninvasive while being of low risk to the patient. However, the information provided by these methods is fairly limited and inferior to the information obtained by coronary angiography. Coronary angiography is the “gold standard” for graft patency assessment. Unfortunately, it is not normally available in the majority of operating rooms. However, specially designed state-of-the-art hybrid operating rooms allow utilization of high-quality coronary angiography intraoperatively. A hybrid strategy combines conventional operating room capability with contemporary endovascular imaging, offering a full range of interventional, imaging, and surgical services in one place. As hybrid operating rooms become more available, increasing use of coronary angiography can be expected.
Background
Coronary artery bypass grafting (CABG) surgery is one of the most common surgical procedures with over 400,000 surgeries performed in the USA and over 800,000 surgeries performed worldwide each year. In appropriately selected patients, including those with multivessel coronary artery disease (CAD), with left main coronary artery disease, and with coronary artery disease not amendable by percutaneous intervention, CABG surgery remains the best treatment modality, resulting in increased survival, relief of angina, restored physical activity, and improved quality of life. However, the long-term benefits of CABG surgery are highly affected by both the CABG procedure itself and progression of CAD. Long-term success of CABG surgery is, to a great extent, related to the type and quality of the graft utilized. The left internal thoracic artery (LIMA) to the left anterior descending artery (LAD) is the only graft that has been shown to provide a long-term prognostic benefit in patients with CAD, primarily related to its resistance to thrombosis and atherosclerosis, and it is therefore considered the “gold standard” conduit in CABG surgery (LAD) [1, 2]. Early patency of LIMA to LAD grafts is almost 100 % followed by long-term patency of 92–99 % at 1 year, 90–95 % at 10 years [3–7]. Despite excellent outcomes of arterial graft utilization, saphenous vein grafts (SVG) remain the most frequently used conduit, despite the fact that they are prone to stenosis and closure. One-year SVG failure rates are about 26 % (range 7–30 %) [3, 7–9], and only about 50–60 % of SVGs remain patent at 10 years after CABG surgery [3, 10–14].
There are three main causes of vein graft failure: acute thrombosis within the first month after surgery, neointimal hyperplasia between 1 and 12 months, and progression of atherosclerosis during the late postsurgical period (more than 12 months). Thus, early graft thrombosis causes occlusion of 3–12 % of SVGs immediately, or early on after the surgery [15–17]. Graft thrombosis is primarily related to technical reasons, such as a small size of the target vessel, resulting in poor distal runoff, size mismatch between the graft and the target vessel creating turbulent flow, and disruption of the endothelial layer resulting from mechanical trauma and manual distention. There is an obvious need for intraoperative graft and anastomosis quality assessment in CABG surgery, in order to assure reestablishing blood flow in occluded or stenotic coronary arteries.
Intraoperative graft patency has conventionally been assessed indirectly using the following diagnostic criteria: absence of new ischemic electrocardiographic changes, absence of new wall-motion abnormalities in the area of the bypass on a transesophageal echocardiogram, good distal runoff on manual palpation, and absence of signs of hemodynamic instability. However, these methods are valuable only if significant myocardial ischemia develops. As a result, a number of different techniques have been developed for routine intraoperative evaluation of graft patency. These techniques include coronary angiography [18–20], intraoperative fluorescent imaging (IFI) [21], and transit time flow measurements (TTFM) [22, 23]. Other modalities such as electromagnetic flowmetry [24], Doppler ultrasound velocity measurements [25, 26], thermal coronary angiography [27], and epicardial color Doppler scanning [28] have also been utilized for graft patency evaluation but failed to reliably detect occluded grafts.
Coronary Angiography
Conventional coronary angiography is still the “gold standard” method for graft assessment. Unfortunately, it is not normally available in the majority of operating rooms (OR). However, specially designed state-of-the-art hybrid ORs allow utilization of high-quality coronary angiography intraoperatively. In 2005, at Vanderbilt Heart and Vascular Institute, we built one of the first hybrid ORs in the USA.
The Hybrid Operating Room
The hybrid operating room combines conventional operating room capabilities with contemporary endovascular imaging, offering a full range of interventional, imaging, and surgical services in one place. An integrated setting means savings in time and personnel, because more procedures can be performed in the same room by the existing staff, without relocating equipment or personnel from another department.
Effective design of the hybrid OR is a long complex process that requires a multidisciplinary team approach. From the beginning, this multidisciplinary team should include representatives from hospital administration and engineering, architects, nursing, anesthesia and radiology technologists, and cardiac perfusions. Staffing within the room includes cardiology, electrophysiology, surgical, anesthesiology, perfusion, and ultrasound teams, in addition to the nursing/support staff. Planners and architects need to recognize roles of each team of medical professionals, finding ways to balance each team’s requirements.
Firstly the size of the hybrid OR should be of sufficient dimensions. Ideally, it should be between 750 and 900 ft2 [29] with a minimum floor-to-ceiling height of 10 ft, in order to accommodate floor- or ceiling-mounted C-arms for 3D rotational angiography [29, 30].
The location of the equipment in the room is another critical problem. Each team of medical professionals brings a different set of equipment into the integrated suite, causing potential conflicts over room space. The challenge of finding locational compromises that preserve efficient use of that equipment can be solved by multidisciplinary teamwork. The primary equipment units required in the integrated suite are the C-arm for 3D rotational angiography and the operating table. Ceiling- or floor-mounted C-arms are currently available, with either possessing some advantages and disadvantages. Ceiling-mounted systems keep the floor space free for the conventional OR traffic, but they negatively impact the OR air filtration system and traditional mounting of the OR lightning. On the other hand, floor-mounted systems keep the ceiling free for traditional OR lightning but complicate OR traffic and flow. Integration of the operating table with the imaging system is essential for efficient use of the radiographic equipment in the hybrid OR. A nonmetallic, reduced-thickness carbon-fiber “OR imaging table,” which is highly stable, has been especially designed for integrated suites. These tables are able to rotate side to side and also recline into standard and reverse Trendelenburg positions. Moreover, the hybrid OR should be equipped with audiovisual and monitoring systems. All medical personnel in the integrated suite should have views of all imaging and monitoring sources. As a result, it is recommended to place at least four ceiling-mounted flat screens that display information from all imaging and monitoring sources in the four quadrants of the room. These screens have to be mounted without interfering with the operating lights. Additionally, the integrated suite should have sufficient storage space for all procedure-related equipment.
Other important matters that require careful consideration are radiation protection and special hygienic requirements. Radiation protection is achieved by two means. First, the hybrid OR has lead-lined walls of 2–3 mm thickness. Secondly, all medical personnel, including the surgical staff, are required to wear lead aprons during any procedure that involves even minimal amounts of imaging. Infection risk is higher in the integrated suite, due to the large size of the room and the large number of personnel and equipment, so it is required to have a laminar air-flow ceiling and carefully maintain sterile techniques. Because angiograms are produced with the X-ray camera moving about an open chest, sterile draping of the open wound and the camera is required. In our hybrid OR, we adopted the practice of triple sterile draping of the open wound and double draping of the camera.
Effective and safe utilization of the hybrid OR requires additional training of the surgical team in order to use the C-arm and the OR imaging table. One of the possible training solutions is simulator-based training in a simulated hybrid OR. This method shortens the learning curve and enhances a level of patient safety.
Depending on the amount of reconstruction and the equipment used to build the hybrid OR, its price range varies between two and four million dollars. This significant investment requires efficient and productive utilization that should be evaluated during the planning period.
At Vanderbilt Heart and Vascular Institute, we have had a very successful experience in designing, implementing, and utilizing the hybrid OR. During the process of implementing the hybrid concept, we learned the significance of close continuous collaboration between several teams of medical professionals, such as cardiology, cardiac surgery, cardiac anesthesia, imaging, and hospital administration. The alliance between the chief of cardiology, chief of cardiac surgery, chief of the catheterization laboratory, and cardiac anesthesia resulted in creating a new working environment and modernization of working space. Thus, we have united cardiology and cardiac surgery ICUs, colocated the cardiology and cardiac surgery offices, and organized interdisciplinary cardiology and cardiac surgery conferences.
We are currently using our state-of-the-art integrated suite to perform both diagnostic and hybrid procedures. The evaluation of clinical outcomes of completion angiography and hybrid treatment modalities is a continuous process. Our intermediate results were published in numerous papers [20, 31–33].
Operative Technique and Results
Our intermediate results with intraoperative completion angiography for graft assessment have been very encouraging. Thus, in selected patients, CABG surgery was followed by coronary graft angiography performed by the interventional cardiologist before chest closure. During this procedure a femoral sheath was placed in the left or right femoral artery via the Seldinger technique, prior to heparinization. At the end of the procedure, the heparin was reversed with protamine, the sheath was removed, and a Syvek Patch® (Marine Polymer Technologies, Danvers, MA) was applied, along with 12 min of manual compression on the groin. Completion graft angiography was contraindicated in patients with advanced vascular disease (grade 4–5 atheromatous disease in the descending thoracic aorta detected on transesophageal echocardiogram) and in patients with preoperative serum creatinine over 2.0 mg/dl. In all patients, iso-osmolar contrast medium iodixanol (VisipaqueTM) was used for angiography with a median dosage of 100 ml (range: 10–800 ml).
Surgical or percutaneous graft revisions were performed if graft defects were detected. Repeat angiography was then carried out to ensure correction of these defects before chest closure. Angiographic defects were divided into three categories: (1) conduit defects, (2) anastomotic defects, and (3) target vessel errors. Defect repair methods were subdivided into three categories based on the repair technique: (1) a minor but important adjustment of the graft without additional cardiopulmonary bypass or aortic cross-clamping, (2) intraoperative PCI, or (3) traditional surgical revision, requiring additional cardiopulmonary bypass or aortic cross-clamping, or off-pump revision of the surgical anastomosis. Patients who underwent open-chest PCI for graft revision received 300 mg of clopidogrel via a nasogastric tube at the time the decision of an unplanned hybrid procedure was made.
Since April 2005, 366 consecutive patients, median age 63 (range 32–89) years, underwent CABG surgery followed by intraoperative completion angiography. Of the 796 grafts, 12 % (97/796) had angiographic defects. These angiographic findings included 54 conduit defects (6.8 %), 30 anastomotic defects (3.7 %), and 13 target vessel errors (1.6 %). Identified defects were corrected with a minor adjustment in 22 (2.8 %) grafts, with intraoperative PCI in 48 (6 %) grafts, and with surgical revision in 27 (3.4 %) grafts. Twenty-five of 345 (7 %) LIMAs had a major defect, of which 10 were located in the conduit and 15 at the distal anastomosis. Thirty-seven of 445 (8 %) SVGs had a major defect, of which 25 were located in the conduit. Long-term (12-month) graft follow-up for these patients is in progress, and we hypothesize that correction of these defects at the time of surgery may have helped reduce the rate of graft failure. We experienced a very low rate of complications associated with completion angiography, observed only one patient with arterial access complication [20], an acute stroke rate of 1 % (5/366 patients), and a rate of acute renal failure of 4 % (14/366 patients) [20]. However, it has been difficult to determine whether the stroke was catheter or surgery related, as well as whether acute renal failure was due to contrast load or due to the surgery alone.
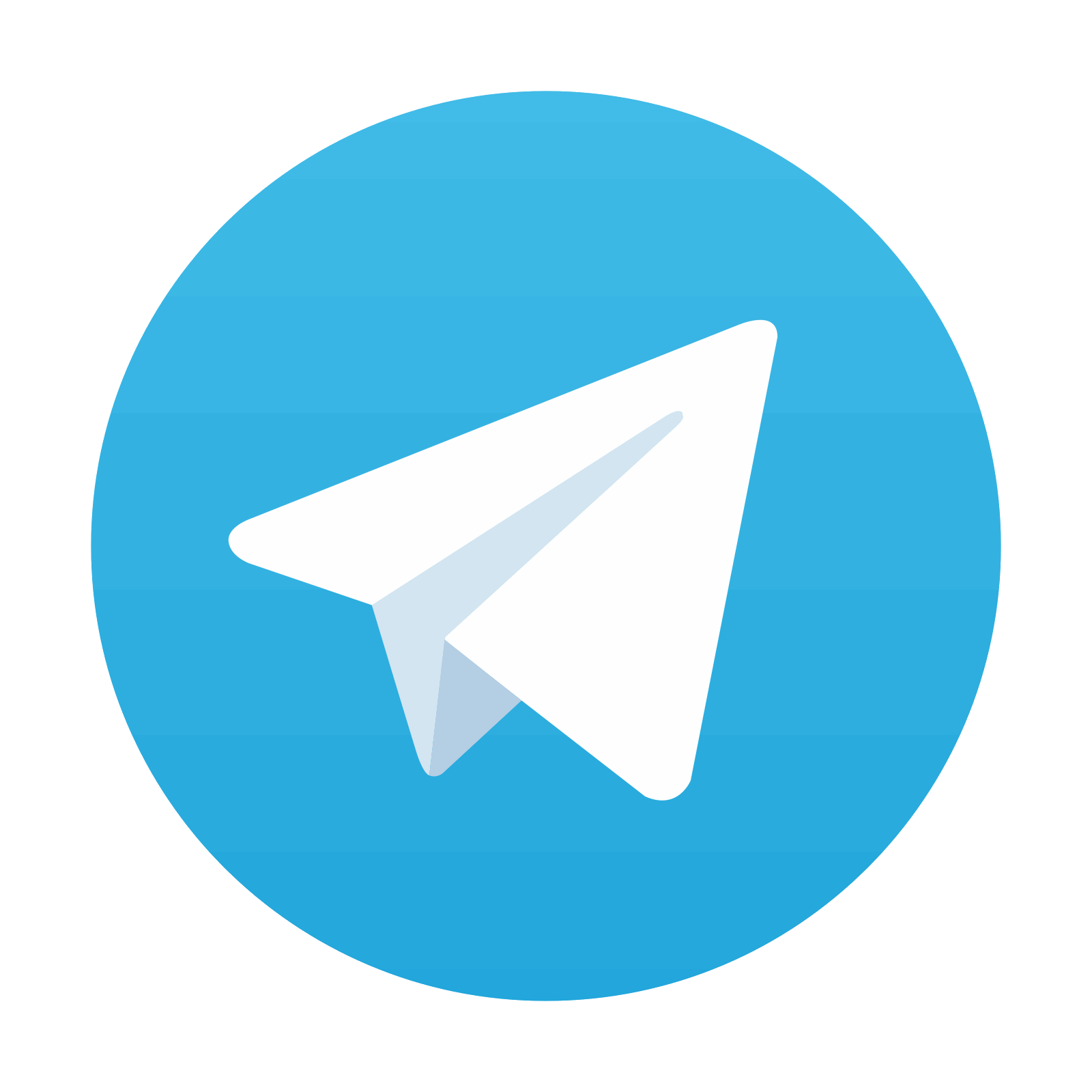
Stay updated, free articles. Join our Telegram channel

Full access? Get Clinical Tree
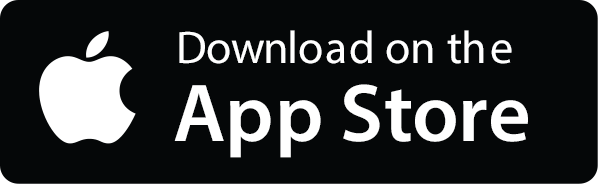
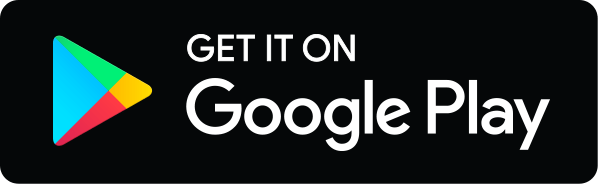