Fig. 38.1
Vertically open configuration midfield intraoperative MRI (SIGNA SP, GE Medical System, Milwaukee WI)
This type of ioMRI configuration allows images to be generated at any time and quite quickly: a set of T2 images over the anatomy of intent adequate to answer questions about localization, lesion residual, or hardware placement can be generated in about 1 min and without manipulation of the patient’s operative site or position. Rapid, single slice acquisition is possible at a rate of up to one image every 3 s. The first pediatric brain tumor craniotomy using iMRI was performed at the Brigham and Women’s “double doughnut” ioMRI suite in 1997 by Drs RM Scott and TM Moriarty. The patient was a 3-year-old with an apparent deep-seated cerebellar tumor. The lesion had been previously unsuccessfully approached using frameless stereotactic navigation. No tumor was identified intraoperatively, and postop MRI showed a surgical defect near, but not within, the lesion. The patient was then taken to the iMRI where near-real-time MR navigation provided a precise path to the lesion and a gross total resection was performed and confirmed by ioMRI. This first case explored the technical considerations related to positioning, instrumentation, and anesthesia for pediatric neurosurgery in an ioMRI.
At the Kosair Children’s Hospital, a part of Norton Healthcare (Louisville, KY), we have a similar GE double doughnut ioMRI system (GE Medical Systems, Milwaukee, Wisconsin) [11]. For 9 years, we further explored the use of ioMRI for pediatric patients. Between 2000 and 2009 this system was used in 282 pediatric cases. The majority of these cases were craniotomies (44 %) followed by cyst management (25 %) and CSF diversion (16 %). During the first 2 years, the cases were mostly craniotomies – the vast majority at 76 %, with only 24 % of cases being cyst drainage or CSF diversion. As time passed, experience yielded lessons on ioMRI usage: not all tumors required ioMRI, and the ergonomics and physical structure of the double doughnut limits some approaches. As a result only 35 % of ioMRI cases between 2007 and 2009 were craniotomies, while 65 % were complex cysts or CSF diversion [12, 13].
Sample Cases
The following three cases highlight some optimal uses of ioMRI technology. Case 1 is a 6-month-old recently relocated to Kentucky who carried a fetal diagnosis by US of an arachnoid cyst. Immediate postnatal MRI and repeat MRI 6 months later showed a large but stable arachnoid cyst. On follow-up in our center, an MRI was obtained which revealed a large arachnoid cyst centered in the interpeduncular and pontine cisterns and extending anteriorly to the sub-frontal space and caudally approximately to the mid-clivus. Ophthalmological and endocrine evaluations were within normal limits, and the patient had no focal deficits and was meeting developmental milestones. However, because of its large size, the parents elected to have the child taken. The child was taken to the ioMRI suite for image-guided placement of a cyst-peritoneal shunt. Figure 38.2a is a preoperative diagnostic MRI showing the large cyst. In the ioMRI but before incision, (Fig. 38.2b) shows a good catheter trajectory and (Fig. 38.2c–e) shows the image-guided placement of a drainage catheter. It is not difficult to appreciate the precision and interactive nature of truly intraoperative MRI in the “double doughnut.” The catheter is passed through the brain and precisely into the foramen magnum to the apex of the cyst. In Fig. 38.2f is a 3-month postoperative MRI showing resolution of the arachnoid cyst. There were no complications and the patient demonstrated a dramatically accelerated acquisition of milestones of neurologic improvement postoperatively.
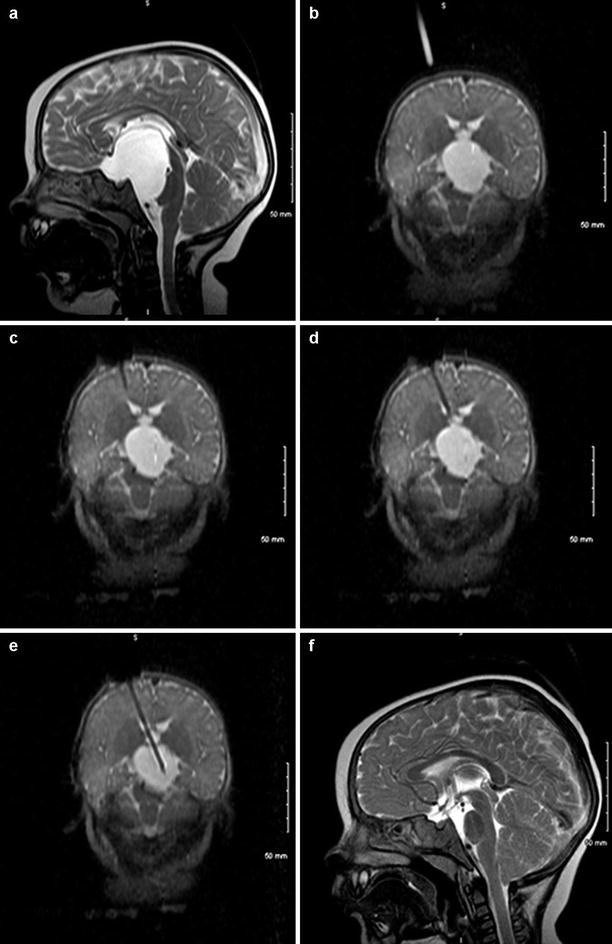
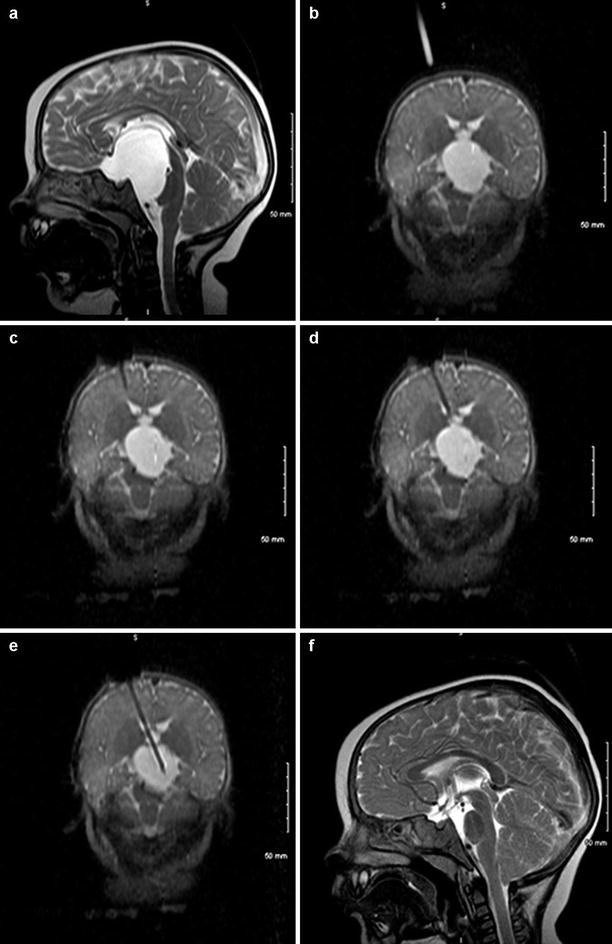
Fig. 38.2
(a) Preoperative diagnostic MRI showing a large cystic lesion before incision. (b) Shows a catheter introduction trajectory. (c) Shows the image-guided placement of a drainage catheter. (f) 3-month postoperative MRI showing resolution of the arachnoid cyst
Case two is an example of how ioMRI can aid in tumor navigation and resection control. This patient was a 13-year-old female complaining of a headache for which she received an MRI which demonstrated a right-sided anteromedial temporal lesion. The patient was without neurologic deficit but, because of the family anxiety provoked, the non-eloquent location and the indeterminate histopathology, resectional biopsy was recommended and elected. Once in the ioMRI, the craniotomy location was determined by pre-incision MR (Fig. 38.3a). A small craniotomy was turned, and a catheter was passed through brain parenchyma under image guidance (Fig. 38.3b, c). After localization with the catheter, a small corticectomy was made, and successful resection is shown in (Fig. 38.3d). Pathology returned low-grade glioma with neuroepithelial features. This case re-demonstrates the precision possible with interactive, real-time imaging of ioMRI with the patient not having to be transitioned for imaging purposes – this exemplifies the “minimally invasive” potential of ioMRI.
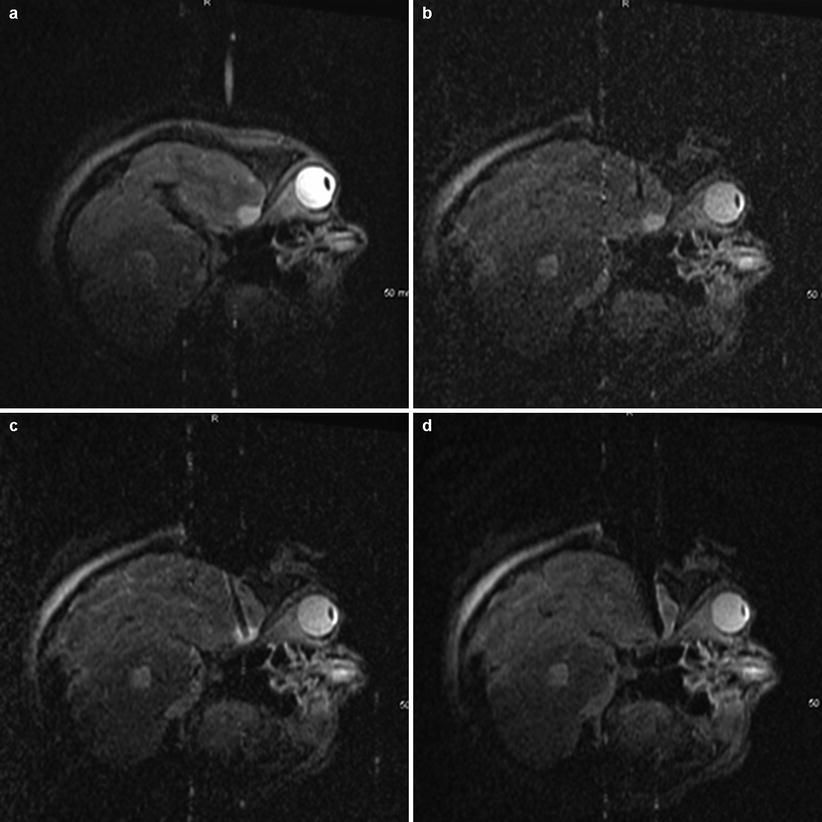
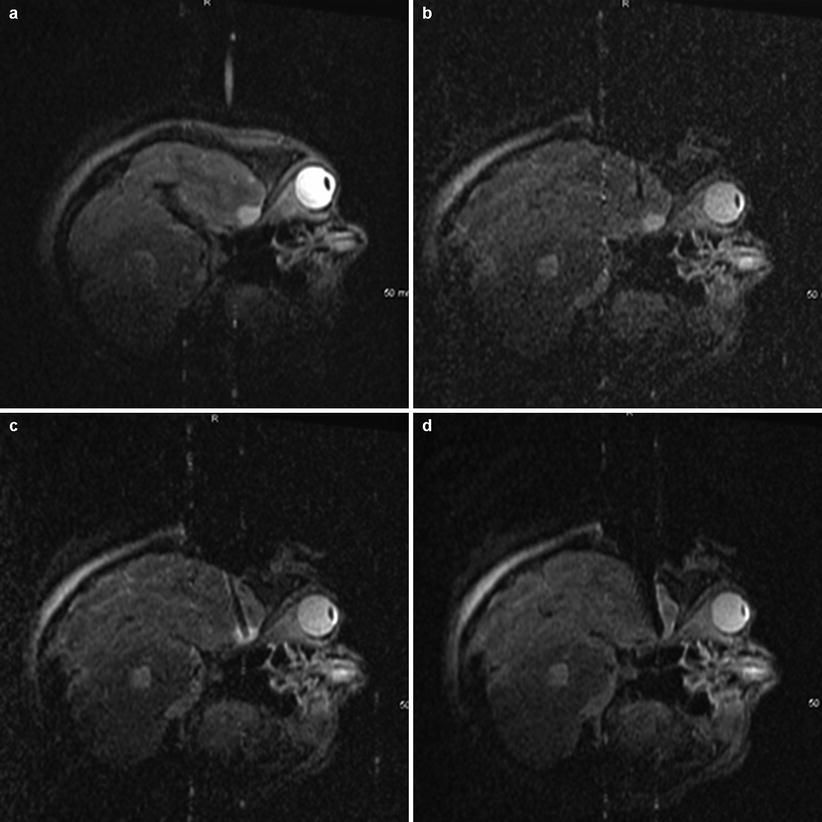
Fig. 38.3
Intraoperative images of a low grade glioma resection. (a) A catheter passed through brain parenchyma under image guidance. (b, (c) After localization with the catheter, a small corticectomy was made. (d) Successful resection
Case three illustrates the use of ioMRI in minimizing the impact of an operation on eloquent tissue. This patient was a 14-month-old male with a headache and a rapidly progressing right-sided hemiparesis. MRI showed a large heterogeneous lesion involving the white matter of the left frontotemporal region (Fig. 38.4a). Starting anterior to the precentral gyrus, the ioMRI was used to precisely navigate to the tumor (Fig. 38.4b, c). Figure 38.4d shows complete resection over four sequential coronal slices. Pathology returned atypical teratoid/rhabdoid tumor. This patient is now 2 years out from surgery without evidence of disease.
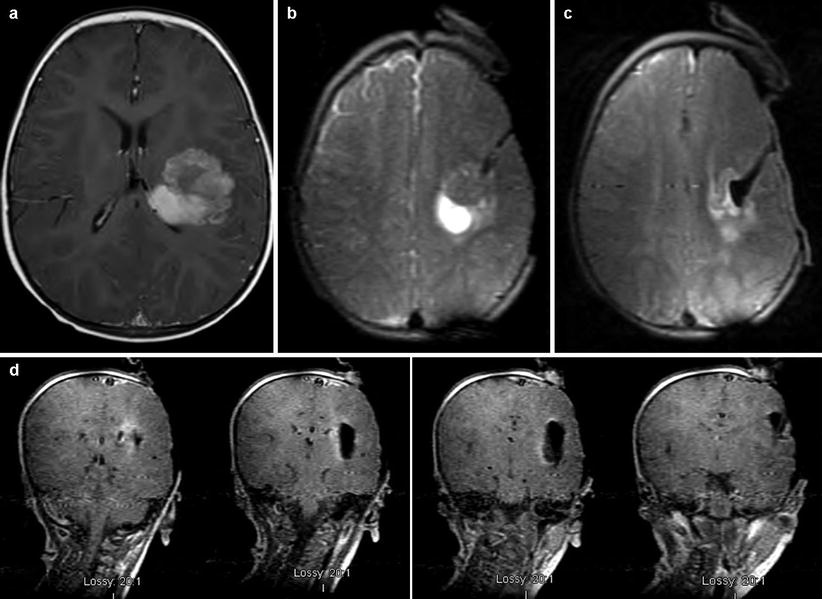
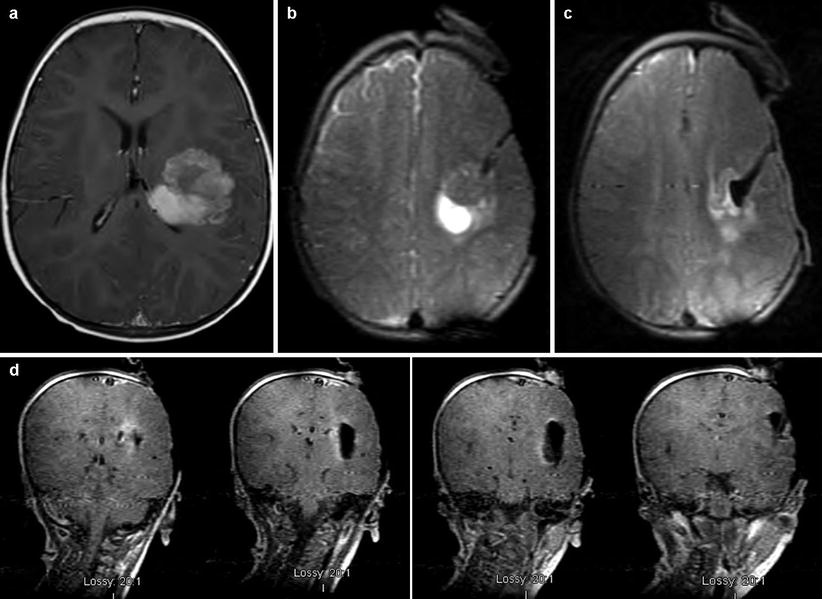
Fig. 38.4
Large heterogeneous lesion involving the white matter of the left frontotemporal region (atypical teratoid/rhabdoid tumor). (a–c) ioMRI is used to precisely localize and approach the tumor. (d) Shows complete resection over four sequential coronal slices
Limitations of the Double Doughnut ioMRI
However, experience revealed several aspects of this configuration which limited its viability. The double doughnut design presents real ergonomic challenges and completely precludes some angles of approach. Instruments compatible with operating within a 5-G line were often of inferior quality, and this environment imposes suboptimal lighting and magnification solutions. Perhaps most importantly, experience in the double doughnut revealed that continuous imaging during most neurosurgical procedures is not necessary, raising the possibility of operating near a 5-G line rather than inside one. Because of its limitations, the double doughnut configuration is no longer commercially available. The market currently offers two types of solutions for the 5-G line constraint.
The first is to reduce the power of the magnet (low-field MRI) in order to keep the patient proximate to the magnet, sacrificing image quality for ease of imaging. There are currently two OR designs using the low-field solution. The first design grew out of work done at Tel Aviv University in Israel by Hadani et al. using a 0.12 T ioMRI consisting of two discs with a gap in between accommodating the OR and area of the patient to be imaged [14]. The discs are mounted directly onto an OR table linked to both an optical and MRI tracking system. When not in use, the apparatus is kept under the table and generates a minimal magnetic field, allowing the use of standard surgical instruments. When in use, the discs are raised to the level of the patient’s head, restricting the angle to the patient and requiring the use of MRI-compatible retractors and clips which minimize the hassle of transitioning to imaging. This system has the significant benefit of a much lower cost since the unit does not require major renovations and RF shielding of the OR. This system has been further developed and marketed by Medtronic (Medtronic, Minneapolis, MN) as the PoleStar Surgical MRI System. The other low-field alternative was developed in Erlangen, Germany, by Fahlbusch et al. and makes use of a two-room suite and an open 0.2 T MRI (Magnetom Open 0.2 T; Siemens AG, Erlangen, Germany) [15]. In one room, standard operative equipment is used well away from the magnet. In the adjacent ioMRI room, there are two operative positions. The first is outside the 5-G line which allows most standard surgical instruments to be used but requires MRI-compatible retractors and clips as well as a modified microscope. From this position, the patient is easily and quickly transitioned into the scanner. The second position is with the patient within the magnet, allowing for immediate imaging. All surgical instrumentation in the second position must be fully compatible.
Data for initial series of patients in both of these configurations has been published in the last decade. For the Polestar, two series of patients, totaling 52 procedures and 49 pediatric patients, have been published by Roth et al. in 2006 and Samdani et al. in 2005 [16, 17]. In Roth’s series, using the PoleStar N-20, there were 20 craniotomies for 18 tumors and 2 cavernomas, 8 shunt placements, 1 biopsy, 1 ETV, and 1 temporal lobectomy for epilepsy. Of the 20 craniotomies, 5 had complete resection on first scan, 10 patients had residual tumor with 8 having a discrepancy between amount on imaging and surgeon judgment, and 5 patients with intraoperative images that were of too low quality to be informative (assumed to be unidentified electromagnetic interference by the authors). In each case, lesion resections were confirmed by postoperative imaging. All 8 intracranial catheters were successfully placed using the iMRI in patients with either difficult or multicystic targets. The biopsy successfully produced diagnostic tissue, and a lack of post-biopsy confirmations was easily confirmed in the OR. Finally, the iMRI distorted the endoscopic images, and ioMRI guidance was abandoned. No infections or complications attributable to the ioMRI setting were reported, though the authors found that use of the iMRI adds 30–45 min of positioning time and 10–15 min of imaging time. While the authors state that the posterior fossa is now able to be imaged by the N-20, none of the patients in this series received an operation for posterior fossa tumors.
In Samdani’s series, a PoleStar N-10 was used, also at 0.12 T. The authors performed 15 craniotomies, 2 shunts, and 1 each of transsphenoidal, craniotomy/transsphenoidal, cranioplasty, and endoscopic biopsy/fenestration. In 8 patients, the operative plan was modified secondary to iMRI information. In 4 patients additional tumor was revealed and safely resected; in 1 patient, additional hippocampus was removed (for unclear reasons); in 1 patient, fenestration of compartmentalized hydrocephalus was confirmed; in 1 patient lack of communication between cyst and ventricle was visualized intraoperatively; and in 1 patient, intraoperative imaging visualization delineated tumor adherence to the optic chiasm. Operative times were increased using iMRI: by an average of 138 min for the first 10 patients but reduced to an extra 84 min for the last 10 patients. No complications or infections attributable to ioMRI were noted in this group, though 1 patient did have an ioMRI of too low quality to be used for decision-making. These authors did report successful ioMRI management of a posterior fossa pilocytic astrocytoma in this series in which total resection was obtained following an iMRI-identified residual. Of note, both authors make mention that the PoleStar is uniquely suited to the pediatric population since they fit more easily between the discs of the under-table MRI system, which allows a greater ease of positioning and imaging of the skull base and posterior fossa.
Similar to the PoleStar system, the open 0.2 T configuration has two series of patients totaling 74 patients and 78 procedures published by Nimsky et al. in 2003 and Kremer et al. in 2006 [18, 19]. In Nimsky’s series of 33 pediatric cases, 9 patients had either cyst drainage or catheter placement, 6 had resection for pharmacoresistant seizures, 6 had sellar tumors (4 treated transsphenoidally, 2 by craniotomy), and 12 had tumors treated by craniotomy. ioMRI contributed passively to decision-making in all patients by confirming goal completion; in the following 5 patients, intraoperative imaging modified their surgical strategy. In 1 patient, intracystic application of contrast showed no communication requiring an additional catheter; 1 patient received intraoperative repositioning of an errant freehand catheter; 1 patient demonstrated real-time collapse of a craniopharyngioma cyst with significant infolding of the cortex, and aspiration was safely halted; in 2 tumor patients ioMRI prompted further resection. No complications were noted; however, two patients developed delayed infections; the authors felt this was not attributable to the ioMRI environment since only 1 other patient in the entire 330 ioMRI patient series (including adults) had an infection, yielding an overall ioMRI infection rate of <1 %. In no case was ioMRI imaging hampered by low image quality. The authors found that for procedures conducted in the adjoining room, transit times were 10–15 min each way for imaging, while for procedures done just outside the 5-G line, transition times were 1–2 min. These authors did not comment on the feasibility of posterior suboccipital approaches in this ioMRI environment.
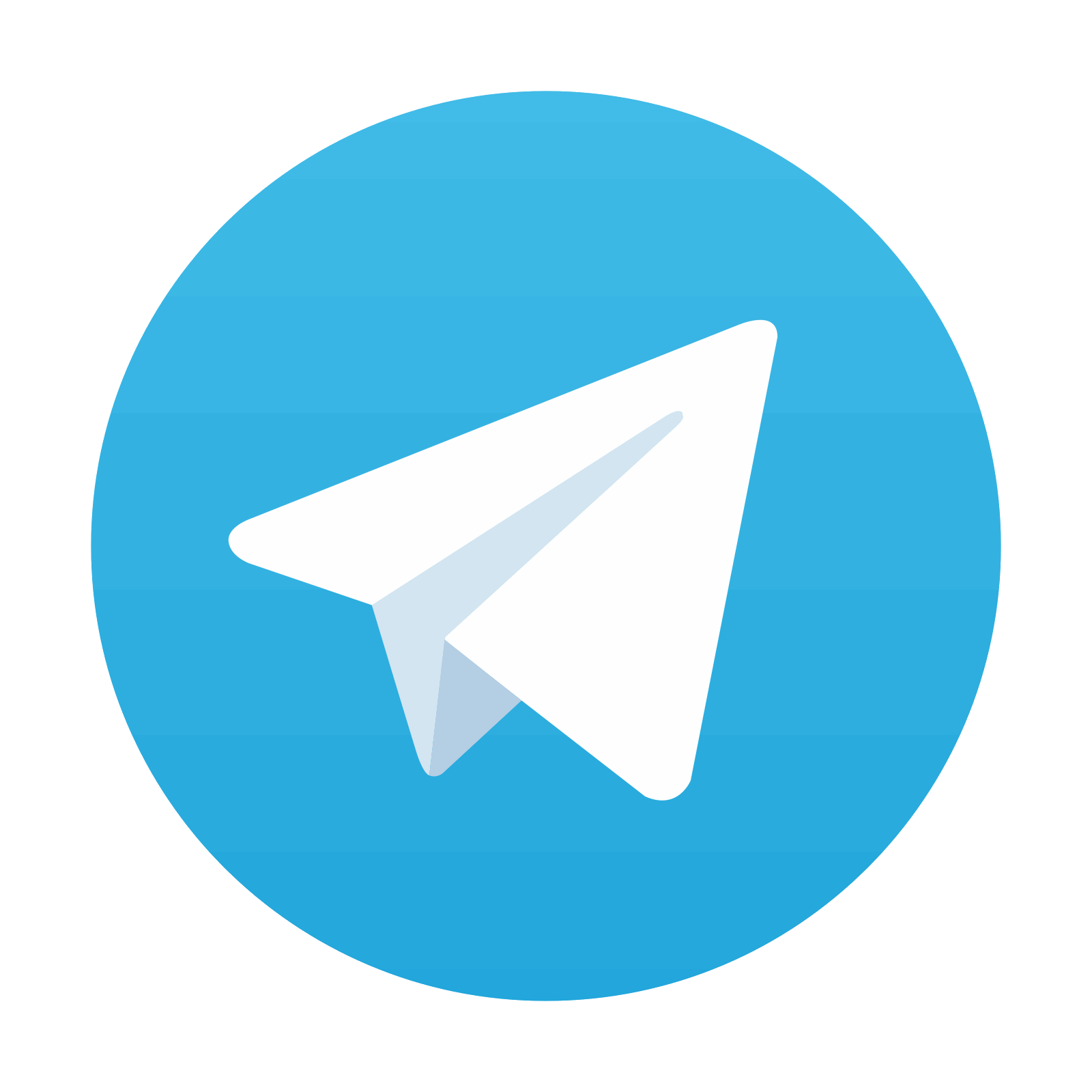
Stay updated, free articles. Join our Telegram channel

Full access? Get Clinical Tree
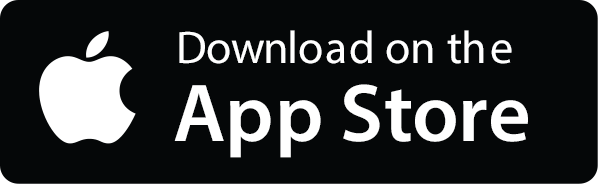
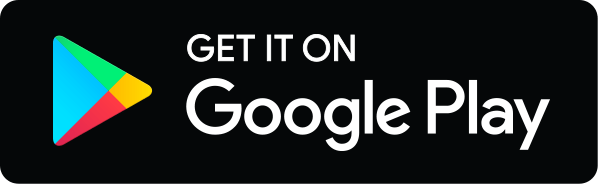