Fig. 1.1
Photoelectric effect (left) and compton effect (right) are the physical basis of X-ray attenuation (Courtesy of GE Healthcare, Waukesha, U.S.A.)
Another important physical phenomenon should be taken into account while thinking of X-rays interactions: k edge.
K edge describes a sudden increase in the attenuation coefficient of photons occurring at a photon energy just above the binding energy of the K shell electron of the atoms interacting with the photons. The sudden increase in attenuation is due to photoelectric absorption of the photons. For this interaction to occur, the photons must have more energy than the binding energy of the K-shell electrons. A photon having an energy just above the binding energy of the electron is therefore more likely to be absorbed than a photon having an energy just below this binding energy.
The two X-ray contrast media iodine and barium have ideal K-shell binding energies for absorption of X-rays, 33.2 and 37.4 keV, respectively, which is close to the mean energy of most diagnostic X-ray beams.
X-ray absorption depends on the inner electron shells: dual-energy CT is sensitive to atomic number and density, but it is not sensitive to chemical binding.
Considering that any substance has a different photoelectric effect, Compton effect and k-edge at different energy levels, it is possible to understand how dual-energy CT works.
In particular at 80 kV, iodine has its maximum absorption; applying different X-ray spectra and analyzing the differences in attenuation, as we can see in dual-energy acquisition, iodine can be easily differentiated from other materials that do not show its behavior at 80 kV. Iodine is the unique material that doubles its HU values from 140 to 80 kV. At 80 kV, some other materials have higher CT values such as bone, metal, but do not double; at 140 kV, some materials have higher CT values, such as fat, plastic, uric acid; moreover, some materials have the same CT values at 80 and 140 kV, such as water, soft tissues, blood. Since these material behavior is known, tissues can be differentiated [3].
Considering iodine properties at 80 kV, in dual-energy CT a virtual-unenhanced image can be generated, and this data may be used for baseline density measurements, thereby making true unenhanced imaging unnecessary and saving radiation.
Using dual-energy post-processing software, the contrast agent can be digitally subtracted from the image. This can be done because the dual-energy index of iodine is significantly different from the dual-energy index of soft tissue and fat. The dual-energy data can also be used to generate a color-coded image that shows the distribution of iodine within the volume of tissue examined by CT. This color-coded display is very sensitive to subtle enhancement.
In abdominal imaging, for example, this can be useful in cases of incidentally detected renal lesions with high attenuation on unenhanced CT, the main differential diagnostic considerations being hyperdense cysts and renal masses (Fig. 1.2).
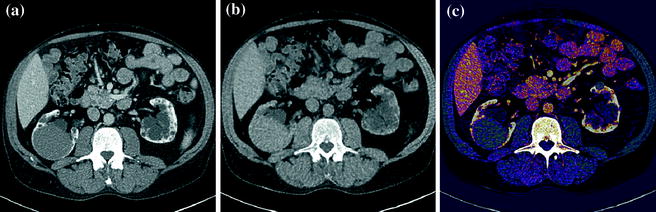
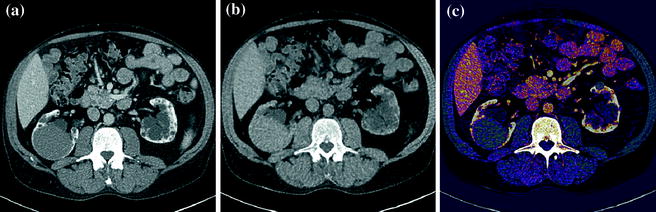
Fig. 1.2
An hyperdense mass can be seen in the diagnostic image (a), in the Virtual Non-Contrast (VNC) image (b), obtained with subtraction of iodine data, the mass results already hyperdense without contrast; moreover, in iodine map image (c) the mass has not iodine enhancement. Therefore, in a single dual-energy acquisition, the diagnosis can be obtained (hyperdense cyst. Courtesy of Siemens AG, Muenchen, Germany)
Immediately after reconstruction, the images can be semi-automatically post-processed, offering the radiologist a fast diagnosis.
In clinical application, dual energy helps to visualize and segmentate vessels, contrast media, tendons, ligaments, kidney stones, gout deposits, perfused blood volume, hard plaque, iodine content in small vessels. Any of these structure often has its dedicated software application for analysis. Moreover, post-processing applications can give additional information about body tissues composition.
Groups of two or three materials are usually analyzed; for example, iodine, soft tissue, and water, or water, calcium, and uric acid (kidney stones application); for the visualization of calcifications in the setting of a contrast-enhanced examination, the algorithm will analyze iodine, calcium, and soft-tissue densities [4].
1.2 Dual-Source CT
In dual-source CT system, released by Siemens in 2005, two X-ray tubes with two corresponding detectors are mounted onto a rotating gantry with a 90 angular offset (Fig. 1.3).
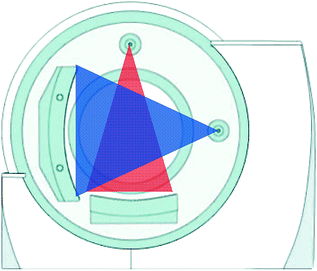
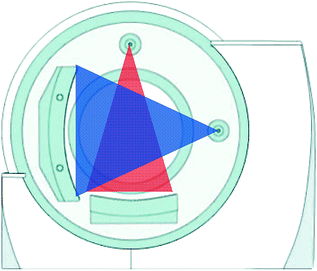
Fig. 1.3
Geometry of dual-source CT (Courtesy of Siemens AG, Muenchen, Germany)
For each tube, a 64-slice design (Siemens Somatom Definition) is present: the “A” detector, which is equal in size to a standard detector (50 cm), and the “B” detector, which also has a 64-slice design, but with a reduced field of view of 27 cm. This detector array provides high spatial resolution of isotropic 0.38 mm edge-length voxels and allows a rapid acquisition of a Z-axis volume.
In 2008, the second-generation DSCT “Siemens Somatom Definition Flash” was introduced. It features even faster gantry rotation (0.28 s), twice the number of detector slices and a larger field of view (332 mm) [5].
This CT system allows almost simultaneous acquisition of two energy data sets, with very few artifacts due to image misregistration.
The acquired projection data primarily have to be reconstructed by standard filtered backprojection, separately for the two simultaneously acquired spiral data sets (80 and 140 kV). Subsequently, materials can be analyzed; this data analysis is called post-reconstruction analysis, meaning that different materials are analyzed after standard reconstruction for each tube. This system differs from material decomposition obtained by GE.
For abdominal imaging, dual-energy CT acquisitions should employ a collimation of 14 × 1.2 mm rather than 64 × 0.6 mm as the latter configuration will cause increased image noise on the B detector images. Since a reconstructed slice thickness below 1.2 mm is usually not required for most applications in the abdomen, this typically does not represent a significant limitation in terms of spatial resolution. However, the data acquired are not isotropic.
Each dual-energy acquisition can generate the following types of data: pure 80 kVp data, pure 140 kVp data, and a weighted average 120 kVp data set that usually is a composition of 70 % from the A (high kV) and 30 % from the B (low kV) tube (Fig. 1.4). This relation can be manually adjusted on a dual-energy workstation.
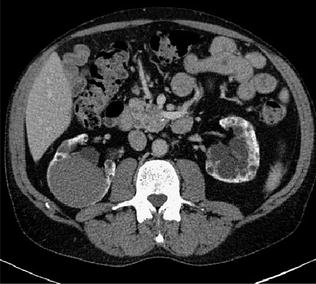
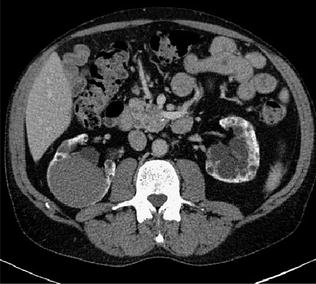
Fig. 1.4
Example of a mixed 140–80 kV image, resulting in a common 120 kV diagnostic image (Courtesy of Siemens AG, Muenchen, Germany)
Note that at same dose a dual energy mixed image has better contrast/noise ratio than 120 kV routine images, due to the contribution of 80 kV data, where the absorption peak of iodine is at its maximum (Fig. 1.5) [3].
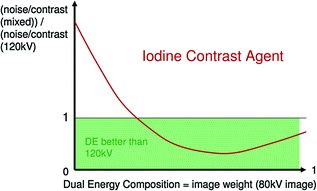
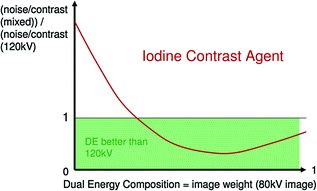
Fig. 1.5
When the contribution of 80 kV data in mixed images is correct (left part), high response of iodine to 80 kV tube current leads to better contrast to noise ratio than simple 120 kV standard images (Courtesy of Siemens AG, Muenchen, Germany)
Dual-source CT, when compared with a single-source system, presents two other possible applications. First, the tubes can be used at equal tube potentials, permitting increased photon flux in larger patients. Second, since an image can be acquired using 2 × 90 degrees of gantry rotation rather than 180, temporal resolution can be increased by a factor of two when using the two tubes at identical kVp levels [4]. Using this technique, a temporal resolution of 70 ms is possible; this is most useful for cardiac imaging.
1.3 Dose
To minimize patient exposure to ionizing radiation, abdominal dual-energy CT protocols operate using an online dose modulation system (CareDOSE 4D, Siemens) that adapts the tube current to the patient’s anatomy [6]. The image quality reference mAs values are usually set to 400 mAs on the B tube and 96 mAs on the A tube, thereby splitting the energy between the two tubes. These settings take into account that higher mAs values on the A tube would lead to increased image noise on the B detector due to scatter radiation. The calculated effective patient doses for abdominal scans will range from 4.5–12.5 mSv, which is similar to the effective dose of a standard abdominal CT acquisition using 120 kVp with 250 mAs [4].
Dual-energy CT is not recommended for patients whose body mass index is >30. In morbidly obese patients, the two tubes can both be operated at 120 kVp, which will help to decrease image noise in these very large patients. The system can be used to scan patients with a body weight of up to 500 lbs (220 kg).
1.4 Limitations
A limitation of first-generation dual-source CT in the abdomen and pelvis is that the smaller size of the B detector will prevent imaging of the entire FOV in larger patients. Therefore, patients may have to be positioned off center if the location of the lesion is in the periphery of the FOV (for example renal masses). Therefore, it is mandatory to acquire two topograms. Moreover, objects at the outer periphery of the B detector may be unable to undergo optimal post-processing due to the technical specifications of the post-processing algorithm. In detail, adjacent voxels have to be used for calculation of the DE properties of any voxel within the field of view. The reconstructed DE field of view is 5 mm smaller than the actual B detector FOV.
1.5 Rapid kVp Switching or Gemstone Spectral Imaging
The CT system released by GE is a single X-ray source system that employs fast kVp switching for dual-energy acquisitions. It is called Gemstone spectral imaging (GSI), and it is based on projection-based material decomposition.
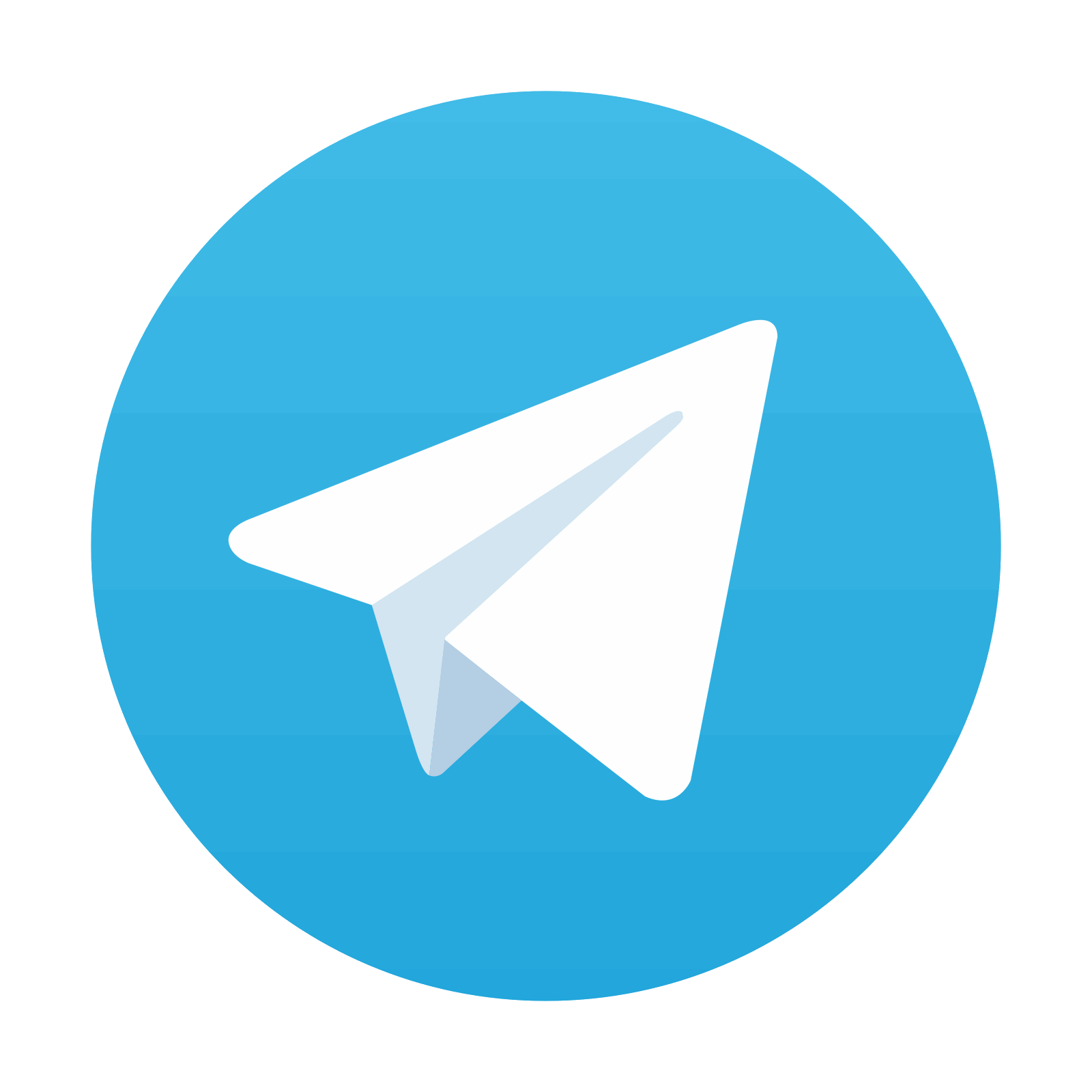
Stay updated, free articles. Join our Telegram channel

Full access? Get Clinical Tree
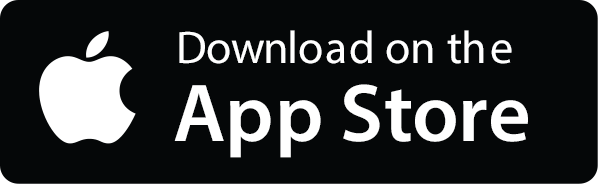
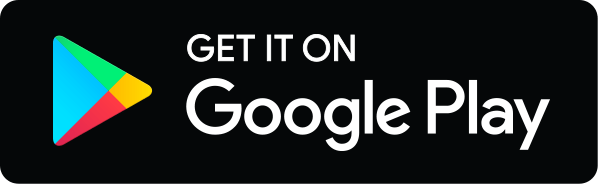