Fig. 59.1
A drawing depicting the developmental changes in the femoral head size, articular cartilage thickness, and epiphyseal vascular anatomy. As the proximal femur matures, the bony epiphysis becomes larger and the cartilage becomes thinner. The blood vessels also do not cross the growth plate cartilage to supply the bony epiphysis. Instead, they enter the cartilaginous epiphysis at the femoral head and neck junction. The onset of ischemic osteonecrosis at an older age would imply less growth and remodeling potentials and a larger bony epiphysis to revascularize and heal (This figure was reproduced with a permission from Texas Scottish Rite Hospital for Children)
59.2.1 Anatomy and Histology of Immature Femoral Head
The femoral head of a newborn is completely cartilaginous on histological assessment. Furthermore, the cartilage contains vascular canals (also called cartilage canals) where blood vessels are distributed throughout the cartilaginous epiphysis [5, 9]. The vessels provide nutritional support to the cartilaginous structure. Around 4–6 months after birth, a secondary center of ossification (also called bony epiphysis or ossific nucleus) forms via endochondral ossification in the central region of the cartilaginous epiphysis. At the same time, the deep layer of the articular cartilage (also called epiphyseal cartilage) overlying the ossific nucleus becomes established as a spherical growth plate around the secondary center of ossification [10] (Fig. 59.2a). This growth cartilage is responsible for the circumferential growth of the secondary center until a full size of the femoral head is reached. As the secondary center enlarges, the articular cartilage thickness decreases and the cartilage canals regress and disappear [11–13]. In humans, their presence has been observed in early childhood, but it is unclear when they disappear [5]. Since a portion of the articular cartilage is responsible for the growth of the secondary center, the thinning of the cartilage during maturation represents decreasing growth and remodeling potentials of the immature femoral head. At skeletal maturity, the endochondral ossification stops, and the cartilage becomes an adult articular cartilage.
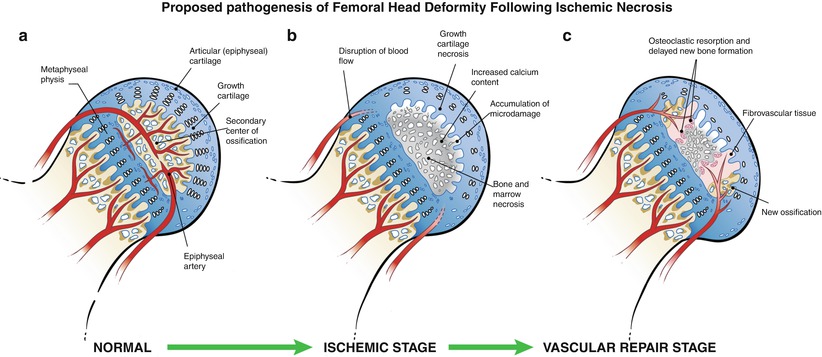
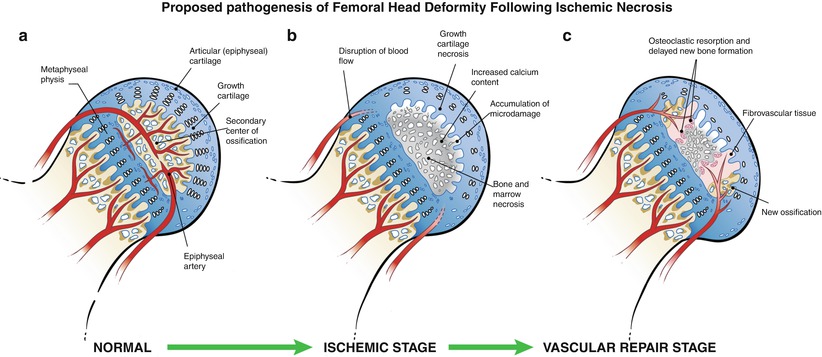
Fig. 59.2
An illustration of the pathological changes observed in the piglet model of ischemic osteonecrosis. (a) Normal immature femoral head showing the growth cartilage surrounding the bony epiphysis. This growth cartilage is responsible for the circumferential growth of the epiphysis through endochondral ossification. (b) Ischemic injury produces extensive cell death in the bony epiphysis (osteonecrosis) and the deep layer of the articular cartilage (chondronecrosis). The ischemic damage to the deep layer of the cartilage produces a growth arrest of the secondary center. Ischemic osteonecrosis is also associated with an increased calcium content of the necrotic bone, which makes the bone more brittle and more prone to microdamage accumulation with hip joint loading. Subchondral and compaction type fracture seen in the initial stage of LCPD may be a result of microcrack accumulation and mechanical loading. (c) Revascularization of the infarcted epiphysis is associated with a predominance of osteoclast-mediated resorption and a delayed bone formation, which further contribute to the femoral head deformity (This figure was reproduced with a permission from Texas Scottish Rite Hospital for Children)
The cessation of endochondral ossification at the femoral head maturity produces histological changes in the subchondral region of the femoral head and, most likely, mechanical changes as well. In the immature femoral head, the subchondral region consists of primary and secondary spongiosa (newly formed immature cancellous bone) due to the ongoing endochondral ossification at the cartilage-bone junction. In the mature femoral head, the subchondral region consists of compact bone (also called subchondral bone plate), and a tidemark forms at the junction of the cartilage and the bone [14]. In the mature femoral head, the nutritional support for the articular cartilage, including its deep layer, is derived mainly from the synovial fluid [15]. In the immature femoral head, the articular cartilage is thicker, and the deep layer of the articular cartilage is dependent on the cartilage canals and the subchondral vascularity for its nutrition [16–19]. Because of this difference, a loss of blood supply to the femoral head in children not only produces a necrotic damage to the bone but also damage to the growth cartilage (chondronecrosis) surrounding the secondary center of ossification as a part of the ischemic damage [20, 21]. The consequence is a growth arrest of the secondary center which is an early radiographic feature of LCPD (Fig. 59.3a). One significant implication of this damage is a potential for growth disturbance of the secondary center once the endochondral ossification is reestablished during healing [17]. Unless the restoration of growth of the secondary center is symmetrical, a further deformity of the femoral head can ensue if the restoration of the growth is asymmetrical (Fig. 59.4). This represents a separate source of deformity from the growth disturbance of the metaphyseal physis which is responsible for the length and the alignment of the femoral neck. The growth disturbance of the metaphyseal physis produces coxa breva (short neck) and coxa vara in LCPD.
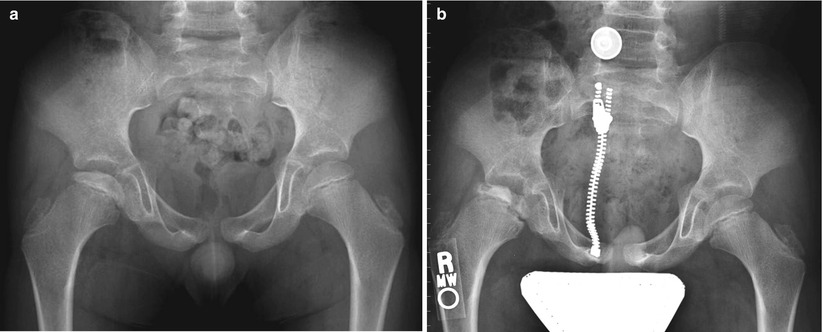
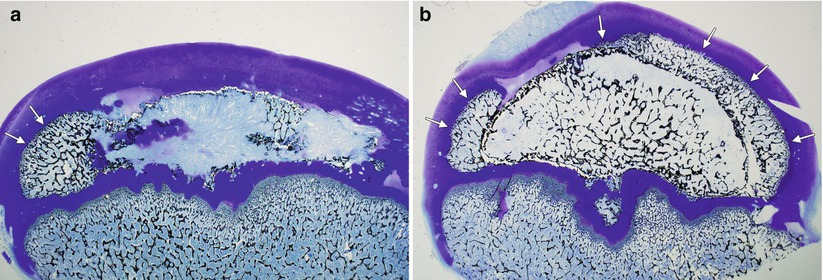
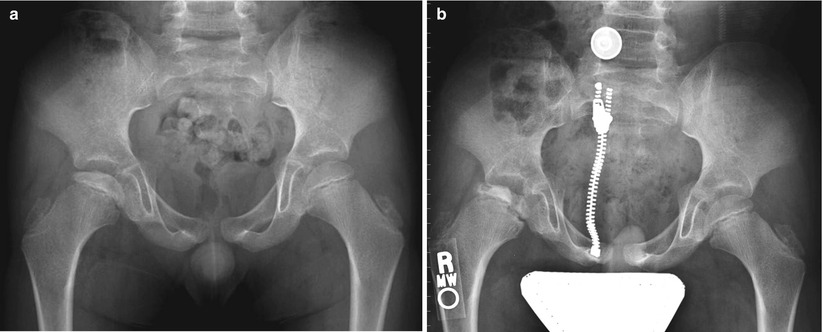
Fig. 59.3
Eight-year-old boy with right hip pain for 5 months. (a) AP pelvis radiograph showing a smaller epiphysis due to growth arrest of the epiphysis. Note the increased radiodensity of the epiphysis. The femoral head is in the initial stage of LCPD. (b) AP pelvis radiograph obtained 5 months later showing fragmented and resorptive changes in the affected epiphysis with further flattening of the femoral head. Most of the deformity occurs during the stage of fragmentation
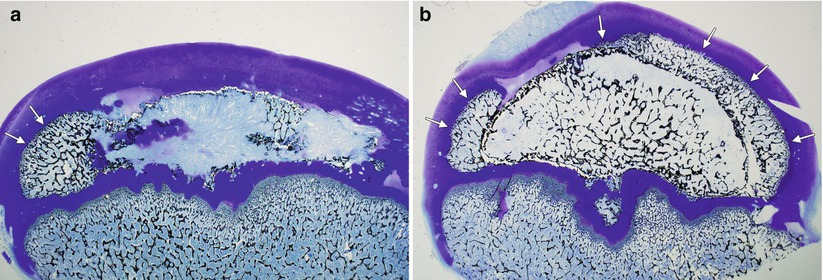
Fig. 59.4
Photomicrographs of the piglet model of ischemic osteonecrosis of the femoral head. (a) A deformed femoral head with restoration of endochondral ossification occurring only at the periphery of the epiphysis (arrows). This asymmetric restoration of epiphyseal growth will promote a deformed femoral head. (b) A spherical femoral head with restoration of endochondral ossification occurring all around the affected epiphysis (arrows). This symmetrical restoration of epiphyseal growth will promote a spherical growth
59.2.2 Healing Potential of Immature Femoral Head
A further distinction between the immature and the mature femoral head comes from an observation that the superficial layer of the articular cartilage shows evidence of a hypoxic and angiogenic repair response acutely after the induction of ischemic necrosis in a large animal model of LCPD [22]. The levels of hypoxia-inducible factor-1, a master regulator of hypoxic adaptation response, and the vascular endothelial growth factor, a potent angiogenic factor, were significantly increased in the cartilage after the induction of ischemic necrosis in the experimental model of ischemic osteonecrosis [22, 23]. Vascular invasion of the cartilage preceded revascularization of the necrotic bony epiphysis. Thus, the cartilage appears to play an active role in the revascularization process in the immature femoral head from the early stage of the disease process. In contrast, the involvement of the articular cartilage in the adult femoral head osteonecrosis is thought to be a late feature of joint degeneration and osteoarthritis [24–26]. Since the adult articular cartilage derives its nutritional support from the synovial fluid, it is unaffected by the ischemic process in the bone. The cartilage involvement occurs at a later stage when the femoral head collapses and degenerative osteoarthritis ensues. It is also believed that the adult articular cartilage does not play an active role in the repair process. An experimental study suggests that the potential for femoral head healing is greater in younger animals compared to older, immature animals because of robust hypoxic and angiogenic responses from the cartilage [27].
The age of onset of FHO is clearly an important variable that influences the femoral head healing potential. For instance, the majority of children with the onset of LCPD before age 6 is known to heal, remodel, and restore the growth of the necrotic femoral head and have a good outcome (defined as a round femoral head at skeletal maturity) [28]. Older patients with LCPD, however, do not heal and remodel their necrotic femoral heads as well as the younger patients, and their prognosis is guarded [29]. The healing potential of FHO in adolescents is generally poor and their outcomes more closely resemble those of adults than those of younger children [7, 8]. While there are appreciable differences in the morphology and the histology of the immature and adult femoral heads as noted above, the molecular mechanisms responsible for the difference in the healing potential remain unknown. Understanding how the onset of osteonecrosis at different ages or stages in childhood (infantile, toddler, preschool, juvenile, teenager, and adolescence) affects the healing potential and the outcome will be important for developing new treatment strategies to improve the poor outcome observed in older children (after age 8) and adolescents affected with LCPD.
59.2.3 Blood Supply to Immature Femoral Head
In addition to the morphological changes taking place in the femoral head with growth and maturation, the blood supply to the femoral head also changes over time. At birth, the lateral half of the head is supplied by the lateral circumflex artery and the medial half by the medial circumflex artery, which are generally branches of the deep profunda artery from the superficial femoral artery [3, 4]. By 18 months of age, the majority of vessels supplying the femoral epiphysis are from the medial femoral circumflex artery, and no vessels cross the metaphyseal physis. Thus, the femoral neck vessels supplying the secondary center of ossification traverse through the articular cartilage at the junction of the femoral head and neck to gain access to the secondary center of ossification. It has been postulated that the portion of the vessels traversing through the cartilage is at risk for disruption from trauma to the hip joint. By 3 years of age, the medial femoral circumflex supplies the entire proximal femoral epiphysis and the metaphyseal physis. The lateral femoral circumflex supplies the greater trochanter and the trochanteric physis. This leaves the femoral head susceptible to vascular injury particularly at the watershed regions in the lateral and anterior quadrants of the femoral head. An additional predisposing factor for femoral head osteonecrosis is the marginal collateral circulation in the femoral head.
With skeletal maturation, the arrangement of the vasculature within the femoral head changes significantly. The regression and the disappearance of the metaphyseal physis allows the vessels from the femoral neck to supply the femoral head without having to traverse through the articular cartilage (Fig. 59.1). In adults, cadaveric dissection studies have shown that the deep branches or posterosuperior branches of the medial femoral circumflex artery perforate the hip capsule at the level of the superior gemellus and travel up the femoral neck as retinacular vessels and enter the femoral head through vascular foramina at the femoral head and neck junction in the superior region of the neck to provide the majority of the blood supply to the femoral head [30, 31]. These studies have been essential for the development of a safe surgical technique to dislocate the femoral head to visualize and treat various intra-articular hip pathologies in adults without causing FHO.
59.3 Pathology and Pathophysiology
It is generally accepted that a disruption of blood supply to the femoral head is a key pathogenic event associated with the initiation of the disease process. Selective angiography [32–34], bone scintigraphy [35], and perfusion MRI [36] provide evidence of a partial or complete disruption of the blood supply to the femoral head. Histological studies of the biopsy specimens [37] obtained in the early stages of LCPD also show findings consistent with ischemic tissue damage. Furthermore, a disruption of the blood supply to the femoral head in a large animal model produces histopathological and radiographical changes resembling LCPD, including growth arrest of the epiphysis, subsequent fragmented appearance of the epiphysis, and the epiphyseal collapse resembling coxa plana [38].
59.3.1 Histopathological Studies of LCPD
A limited number of histopathological studies on LCPD have been performed. These studies consist of case reports and limited surgical biopsy studies of LCPD patients in the active phase of the disease providing a limited view of the disease which is known to have a wide clinical variability. Only a handful of whole femoral heads have been examined and reported to date [20]. Very little is known about the pathological changes in the preclinical stage of LCPD and the temporal changes that occur within each femoral head over time.
The findings from the limited number of histopathological studies [20, 21, 37, 39–44] can be summarized as follows: LCPD affects the articular cartilage, the bony epiphysis, and, in some cases, the physis and the metaphysis. The articular cartilage damage is observed in the deep layer of the cartilage which is a growth cartilage responsible for the spherical growth of the bony epiphysis. The necrosis in the deep layer of the cartilage produces a cessation of endochondral ossification. This explains why the affected epiphysis is smaller than the unaffected epiphysis in a patient who is growing. Other changes observed in the deep cartilage layer include a separation of the cartilage layer from the underlying subchondral bone, degenerative changes in the cartilage matrix, vascular granulation tissue invasion of the cartilage, and appearance of new accessory ossifications. In the affected bony epiphysis, the necrosis of marrow and bone cells, compression fracture of trabeculae, osteoclastic resorption, fibrovascular granulation tissue invasion of the necrotic head, absence of osteoblasts, empty lacunae in the trabecular bone, and thickened trabeculae have been reported. The metaphyseal growth plate changes are most often seen in the anterior part of the femoral head with focal areas of growth cartilage columns extending below the endochondral ossification line. Since premature growth arrest of the growth plate is seen in about 30 % of patients, it is questionable whether the growth plate damage is a primary feature of LCPD. Another possibility is that the growth plate damage is a result of the mechanical damage from overloading of the femoral head as the mechanical load is transmitted from the compressed, hard necrotic bone to the underlying growth plate. An experimental study in piglets showed that a total disruption of the epiphyseal blood supply does not produce growth arrest in a majority of the animals, suggesting that the metaphyseal growth plate is not dependent on the epiphyseal blood supply [45]. Metaphyseal radiolucent changes are sometimes seen in the early stages of LCPD. The mechanisms responsible for the appearance of these lesions are unclear. Various tissue types have been reported including columns of normal or degenerated cartilage extending down to the metaphysis, fibrocartilage, fat necrosis, vascular proliferation, and focal fibrosis [20]. Some have found an association between the presence of radiolucent metaphyseal changes and poor prognosis while others have not.
59.3.2 Pathophysiology Based on Experimental Studies
The lack of availability of clinical specimens to systematically investigate the pathophysiology of LCPD is a significant hindrance to our understanding of LCPD. To circumvent this obstacle, large animal model s have been developed to study the pathophysiology of ischemic osteonecrosis. Of these models, a piglet model of ischemic necrosis has been extensively investigated [17, 38, 45–48]. While the animal model is not a perfect model of LCPD, no perfect model exists currently. The model does provide some new insight about the ischemic bone injury and repair process in the immature femoral head.
Following the induction of ischemic FHO in immature pigs, the earliest histological changes are seen in the marrow space with the death of various cells present, such as the hematopoietic cells, adipocytes, endothelial cells, and stromal cells [17, 49]. Death of osteoblasts lining the trabeculae, osteocytes within the trabecular lacunae, and osteoclasts also takes place in the area of ischemia. The mechanisms of cell death include apoptosis (programmed cell death) as well as necrosis [49]. The appearance of empty lacunae in the trabecular bone, which signifies osteocyte death, is a classic feature of osteonecrosis but a late histological sign taking a few weeks to develop.
Cartilage necrosis (chondronecrosis ) is a consistent feature of ischemic injury to the immature femoral head. Induction of ischemic necrosis in the piglet model produces cell death in the growth cartilage surrounding the secondary center (i.e., the deep layer of the articular cartilage) (Fig. 59.2b) [17, 22, 23]. This explains the cessation of endochondral ossification at the growth cartilage-bone junction in the initial stage of LCPD. The other significance is that in order for the epiphyseal growth to resume, the necrotic cartilage has to be repaired and the endochondral ossification reestablished. If the epiphysis becomes deformed over time, the resumption of epiphyseal growth may not be symmetrical. The revascularization and repair of the necrotic cartilage preferentially occur in the periphery of the deformed femoral head and not at the central region. Thus, the reestablished epiphyseal growth may not be spherical as seen in the normal epiphysis and further deformity due to the growth disturbance may occur. In contrast to the growth cartilage in the epiphysis, the metaphyseal physis (the proximal femoral growth plate) is generally spared [45]. In most of the animals, the growth plate did not develop a physeal bar. Endochondral ossification continued following a total disruption of blood flow to the immature femoral head, albeit at a slower rate than the growth plate on the normal side.
In the piglet model of ischemic osteonecrosis, revascularization and healing of the necrotic femoral head come in the form of fibrovascular granulation tissue invasion of the necrotic cartilage and the necrotic marrow space [38]. The new invading vessels arise from the existing vessels on the femoral neck. The angiogenic process is stimulated by increased production of a potent angiogenic factor called the vascular endothelial growth factor (VEGF) which is actively expressed by viable chondrocytes in the superficial region of the articular cartilage [22, 23]. Hypoxia is a potent stimulus for the activation of the hypoxia-inducible factor-1 (HIF-1) which increases VEGF production and angiogenesis. New vessels invade through the cartilage to reach the necrotic secondary center from the periphery of the epiphysis [22]. In general, the invading vessels do not cross the metaphyseal physis.
The fibrovascular granulation tissue consists of inflammatory, mesenchymal (fibroblast-like in appearance), and endothelial cells. In the revascularized regions of the bone, increased presence of osteoclasts is observed with increased osteoclastic resorption of the necrotic bone. The predominance of bone resorption produces a net loss of bone [38]. New bone formation is absent in these areas where the necrotic bone is replaced by a fibrovascular tissue. Radiographic and histological studies of the patients with LCPD also show resorptive changes in the necrotic bone with delayed reossification of the resorbed areas [50]. In LCPD, the resorptive phase of the disease represents the fragmentation stage where radiolucent changes are seen on the radiographs, producing fragmented and resorptive changes in the sclerotic epiphysis (Fig. 59.3b). During the fragmentation stage, most of the deformation of the femoral head takes place indicating that this is the most mechanically vulnerable stage of the disease. As seen in the piglet model, new bone formation (termed reossification in LCPD) does not occur until many months later. Furthermore, the reossification stage is the longest stage of LCPD, especially in the older patients [51].
59.3.3 Pathology of Adult Femoral Head Osteonecrosis
In adult FHO, most of the infarcted region of the femoral head remains necrotic over time with the extent of revascularization and repair taking place being limited to the border of the viable and the necrotic bone, which is called the reparative or hyperemic zone [24–26, 52]. In the reparative zone, a slow healing process described by Phemister as a “creeping substitution” is observed [53]. The term describes the process in which the dead bone is substituted with new bone. In the past, the term “creeping substitution” has been used to describe the repair process in LCPD. However, the application of the term “creeping substitution” to LCPD is incorrect as the repair process in LCPD is staged with resorption occurring first followed by a delay in new bone formation. There is no immediate substitution of the dead bone with the new bone. Instead, the dead bone is substituted to a fibrovascular tissue.
Based on clinical observations, the repair process in adult osteonecrosis appears to be limited if >30 % of the femoral head is involved. Because of the inability to repair a large area of necrosis in adults, the femoral head collapses due to a mechanical failure of the necrotic bone, most often at the junction of the necrotic bone and the reparative zone. A segmental collapse of the necrotic region of the femoral head produces a disruption in the smooth articular surface which leads to the development of premature osteoarthritis.
59.4 Pathogenesis of Femoral Head Deformity
One of the most significant sequelae of LCPD is the development of the femoral head deformity. The experimental studies using the piglet model reveal that the pathogenesis of the femoral head deformity following ischemic osteonecrosis is complex with multiple contributing factors. Mechanical studies on the piglet model reveal that ischemic osteonecrosis induces a significant mechanical weakening of the immature femoral head [47, 48].
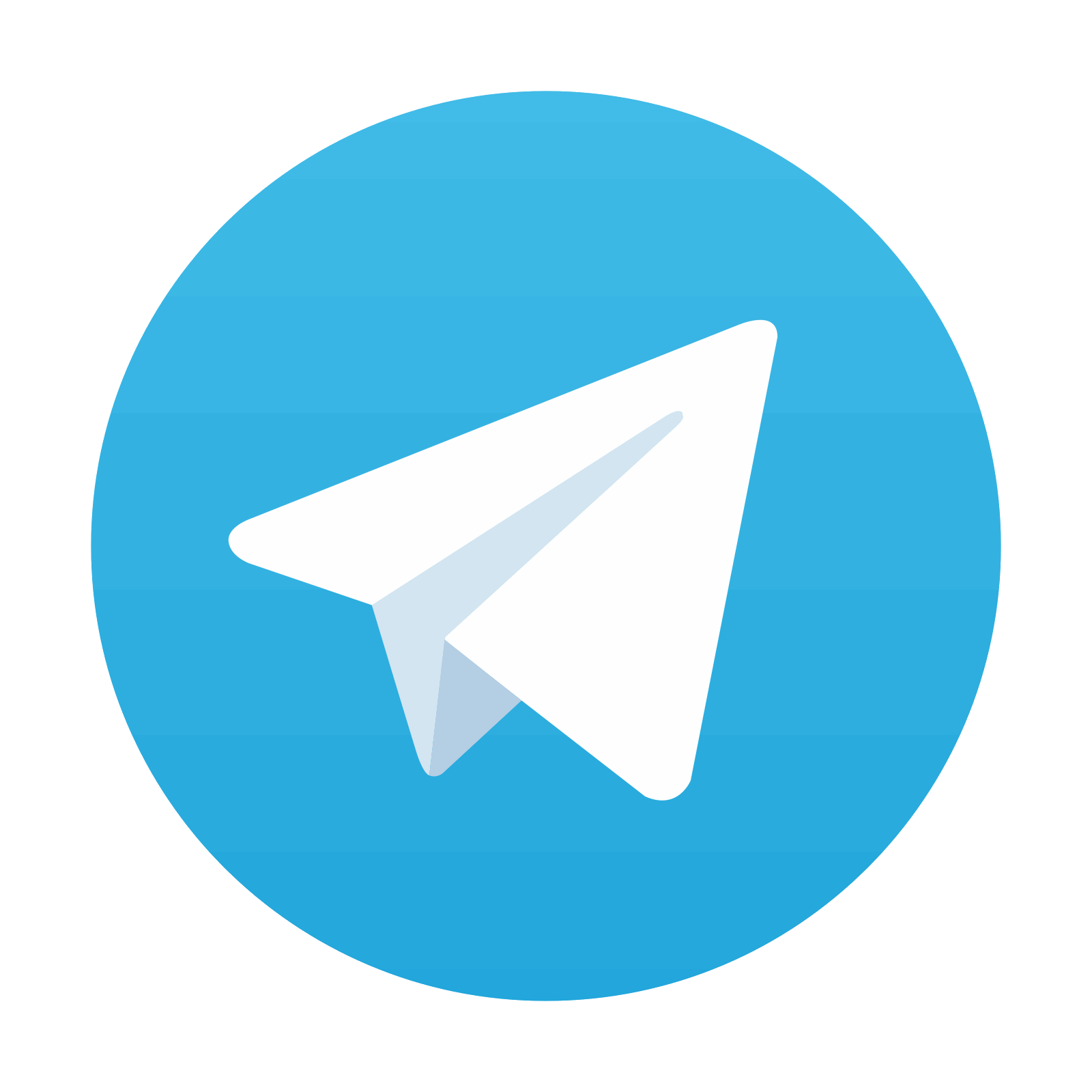
Stay updated, free articles. Join our Telegram channel

Full access? Get Clinical Tree
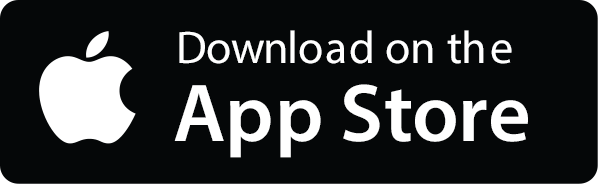
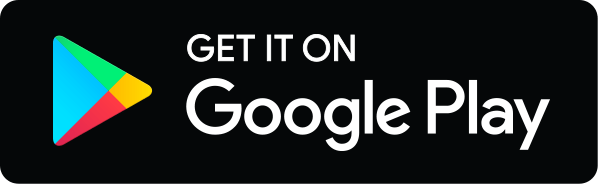