Fig. 8.1
Left pneumothorax. Supine chest X-ray acquired in the emergency room (a) (note the opacity of the spinal support over imposed) where is scarcely appreciable a “deep sulcus sign” at the basal left of the radiogram (arrows) suggesting a subtle layer of anterobasal left PNX, associated with a rib fracture (arrowhead). The signs are better depictable in the magnification (b). The chest CT examination in (c) confirms the diagnosis
Due to the lack of sensitivity of chest X-ray in the supine position, US has progressively been taken into account. In 2010, an evidence-based review of chest X-ray and US in post-traumatic pneumothorax, found a US sensitivity ranging from 86 to 98% in the enrolled studies, an US specificity of 97–100%, a supine chest X-ray sensitivity of 28–75%, and a chest X-ray specificity of 100%, suggesting that bedside thoracic US is a more sensitive screening test than supine chest X-ray in detecting pneumothorax in blunt chest trauma [16, 22]. In a recent study, compared to chest CT as gold standard, the sensitivity of bed US to detect pneumothorax was 88% and the specificity 99.5%; these results are very similar to those reported in the previous review [21].
To detect a pneumothorax, the area of interest corresponds to the anterior part of the chest on both sides of the thoracic wall, approximately the third to eighth intercostal space between the parasternal and the midclavicular lines. It is essential that the results obtained have to be compared with the contralateral site. The probe is moved longitudinally in order to study the intercostal tissues on the real-time image. The hyperechogenic pleural line between the shadows of two ribs must be detected. The necessary criteria to diagnose a pneumothorax sonographically have been assessed in the “International evidence-based recommendations for point-of-care lung ultrasound” [17] and consist in absence of respiratory lung movement during dynamic examination (the so-called lung sliding sign), absence of lung pulse at power color Doppler imaging, absence of B-lines (vertical narrow based lines arising from the pleural line to the edge of the ultrasound screen, the so-called comet-tail image), detection of a “lung point” that reflects the border between the movement of the lung during respiration, and the area of absence of the sliding sign due to the pneumothorax [17] (Fig. 8.2).
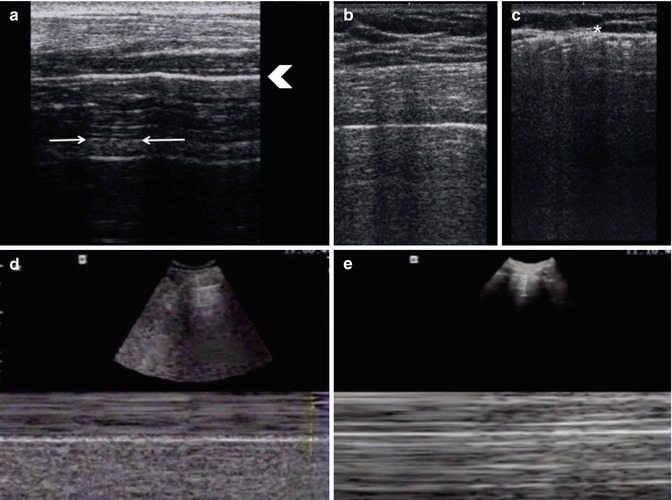
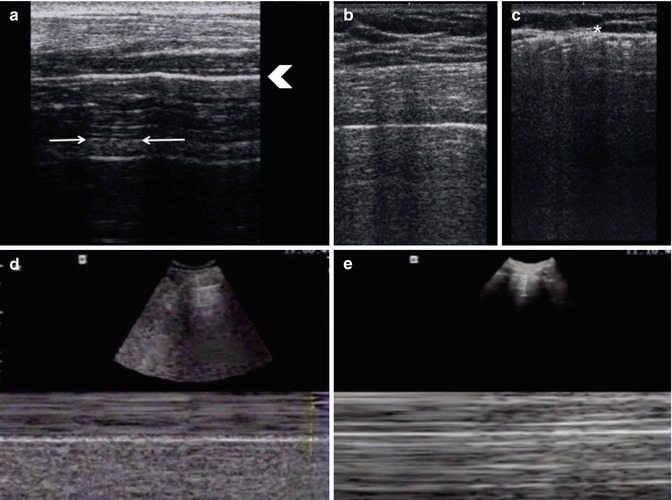
Fig. 8.2
US images to detect a pneumothorax. Despite the fact that diagnosis is purely dynamic, we have to find some basic features: first of all, the hyperechogenic pleural line between the shadows of two ribs must be detected (arrowhead in a). It is crucial to find the “lung point sign” (arrows in a) that reflects the border between the movement of the lung during respiration and the area of absence of the sliding sign due to the pneumothorax. It is essential that the results obtained have to be compared with the contralateral site: in (b) it’s possible to detect the hyperechogenic pleural line in the left lung, instead in the right one (c) there is subcutaneous emphysema that creates an acoustic barrier (asterisk). M-mode on a physiological pleura (d), and on a pathological one (e). (Courtesy of Dr. G. Volpicelli, Department of Emergency Medicine, San Luigi Gonzaga University Hospital, Turin)
While the absence of lung sliding can be seen in conditions in which there is no lung movement against the thoracic wall, for example, after pneumonectomy, pleuroparenchymal adhesion, or subpleural bullae [23], the “lung point” sign has been shown to be 100% specific for pneumothorax and should routinely be sought in all the patients with loss of lung sliding [24].
In spite of its high sensibility and specificity, we must take into account US limitations. In addition to the above-mentioned referring to the patient constitution, the presence of anterior subcutaneous emphysema and the operator skill, we must not forget that small, apical, and dependent pneumothoraces are poorly detected using thoracic US. A perspective study in 2012 correlated the site of pneumothoraces detected with chest CT with the capability of the previously acquired chest US to detect them. They found that the frequency and the extent of pneumothoraces both increments moving towards the medial and inferior thoracic regions which should always, in the authors opinion, be the first to be scanned [25]. Another limitation of chest US examination is that quantification of the pneumothorax is not easy [10] even if Volpicelli et al. in 2014 demonstrated that in supine patients, the more posterior the lung point is located, the greater the pneumothorax is, as compared to CT volume measurement [26].
MDCT offers confident diagnosis of the pneumothorax itself plus a panoramic view of all thoracic structures allowing the detection of associated injuries and accompanying pathological processes. In particular, it allows to detect the exact number and site of rib fractures, the associated lung injuries and the definition of presence and extent of an eventual subcutaneous emphysema, the latter frequently associated with pneumothorax when rib fractures are involved [11, 19, 27] (Fig. 8.3).
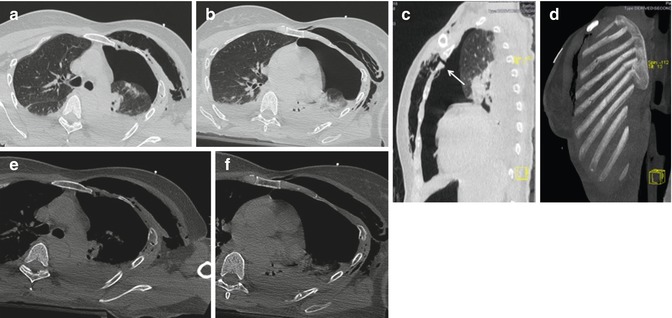
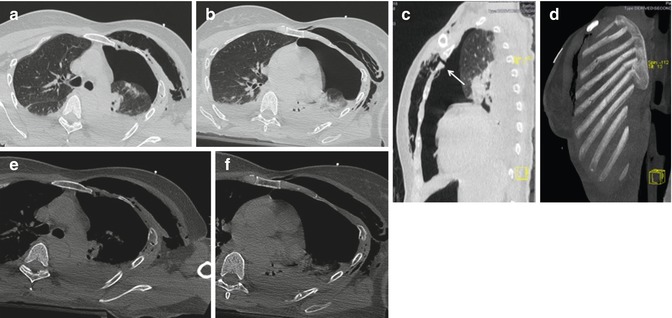
Fig. 8.3
Left pneumothorax. Axial CT-scans in (a–f) shows large pneumothorax located in the anteromedial regions with extensive chest wall emphysema. Lung parenchyma window (a–c) best helps to detect the intrapleural and intramural air collections while bone-window (e–f) permits to determine site and number of rib fractures. Note the panoramic views, often very useful for surgeons or clinicians, offered by sagittal (c), and maximum intensity projection (d) images. In the sagittal CT-image, you can also depict the mural disruption which permits the passage of the air from the interpleural to the intramural space (arrow)
Intrapleural air usually collects in the nondependent regions of the thorax and, in particular, as the patients during CT scan is in a supine position, in the anteromedial basal pleural recesses [27] (Fig. 8.4). Sometimes however, especially in little pneumothorax, air can be detected nearby the rib fracture, wherever the fracture is (also if in a dependent region). This fact may be due to preexisting or trauma-related pleural adhesions which can delimitate small air collections (Fig. 8.5).
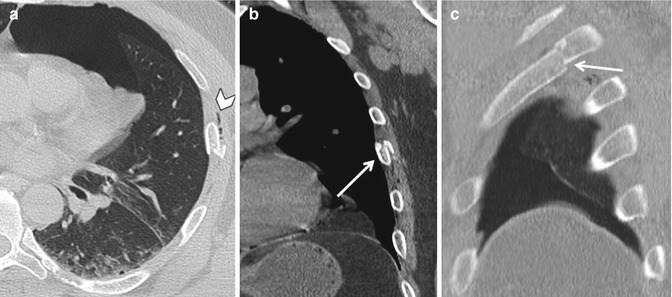
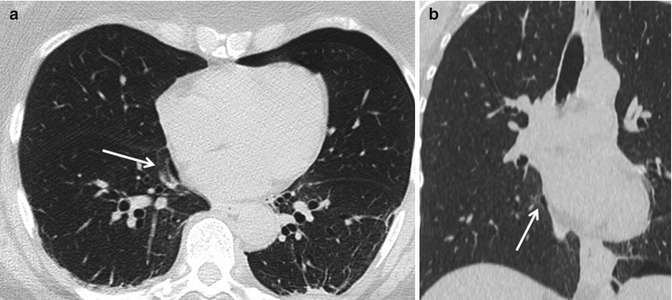
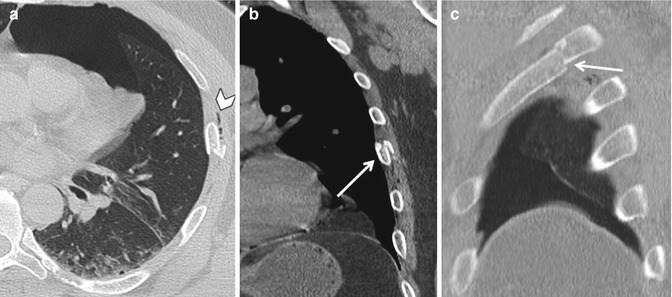
Fig. 8.4
Left pneumothorax. Axial CT-scan (a) shows a pneumothorax localized in the anterior-basal site, associated with rib fracture (arrow) and subcutaneous emphysema (arrowhead), as it is shown in coronal (b) and sagittal (c) reconstruction
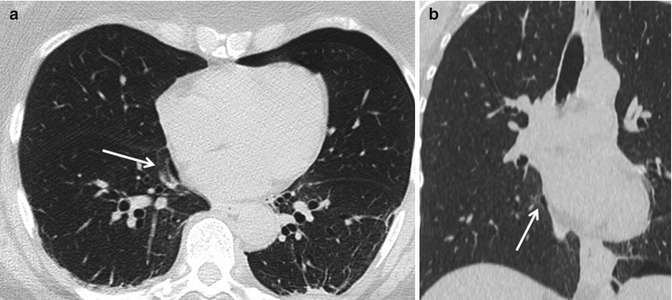
Fig. 8.5
Bilateral pneumothorax. Left pneumothorax located anteromedially and a very small air collection in the right para-mediastinal space (arrows): axial (a) and coronal (b) CT-scan. Note the absence of rib fractures in the right hemithorax: here, the main mechanism involved in the intrapleural air collection may have been parenchymal disruption due to the high increase in intrapulmonary pressure
A tension pneumothorax is a less common and more dangerous variant of the simple pneumothorax and occurs when air continues to enter the interpleural space with each breath, either via laceration in the chest wall or via an injured lung, but cannot escape [20]. The resultant progressive increase in volume of trapped air will compress the adjacent lung and diminish its expansion leading initially to respiratory distress and hypoxemia. As the interpleural volume of air continues to increase, the mediastinal structures, including the vena cava, are also displaced and compressed, producing an eventual drop in cardiac preload due to diminished venous cardiac filling pressures. The result is compromised cardiac output which usually rapidly progresses to cardiogenic shock and eventual cardiac arrest [20].
In this life-threatening situation, chest X-ray permits to depict in a few minutes the hyperlucency of a hemithorax plus the opacification of the contralateral (occupied by the mediastinal structures and by the contralateral collapsed lung) thus permitting immediate pleural drainage which is necessary as promptly as possible in order to save the patient’s life [7] (Fig. 8.6). We must remember that only a mediastinal shift from its median localization is not a definite sign of a tension pneumothorax as it can also occur in a massive lung athelectasia. So it is important to look for hyperlucency of a hemithorax associated with the ancillary signs of overinflation: flattened or inverted diaphragm profile and enlarged intercostal spaces [7]. Sudden evacuation of a large pneumothorax with tube drainage can be complicated by re-expansion pulmonary edema [28, 29] which is clearly visible in a chest radiogram, being characterized mainly by immediate appearance of subtle opacifications in the ipsilateral lung that can rapidly progress to the total opacification of the entire hemithorax. The complication is more common in younger patients (20–50 years of age), occurs more often than was previously believed, and it has a reported variable mortality rate reaching 20% [29]. So, a control chest X-ray should always be acquired after positioning a drainage tube in a hypertension pneumothorax as it exactly localizes the tube apex and helps us to rapidly diagnose acute complications of the invasive maneuver [7].
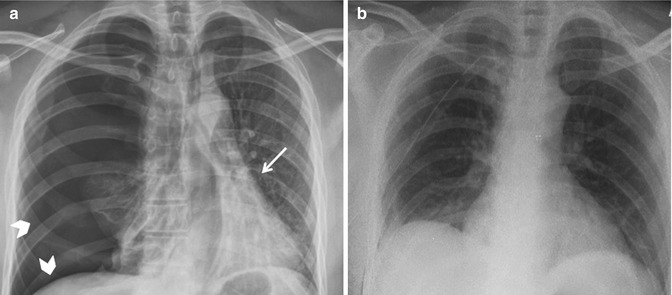
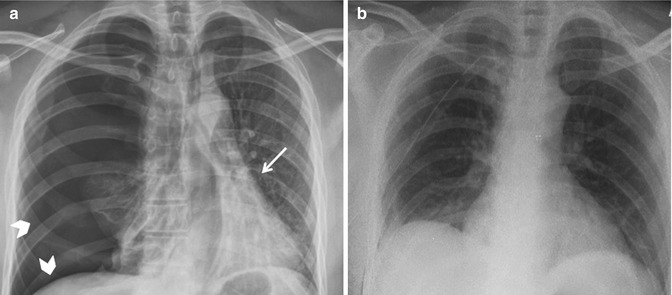
Fig. 8.6
Tension pneumothorax. Chest X-ray (CXR) in (a) shows the radiological signs of massive tension pneumothorax: contralateral dislocation of the mediastinum (arrow), hyperlucency of the ipsilateral hemithorax associated with flattening of the diaphragm and increment of the intercostal spaces (arrowheads). (b) CXR control after drainage positioning. Note the complete re-expansion of right lung without any sign of edema
MDCT signs are much the same as in conventional radiography, namely contralateral mediastinal shift and collapsed ipsilateral and contralateral lung in a hyperexpanded ipsilateral hemithorax. Also in MDCT imagines, we can find a flattened or inverted ipsilateral diaphragm [19] (Fig. 8.7). Sometimes, with MDCT we are able to find the exact site of the laceration responsible for the intrapleural air accumulation.
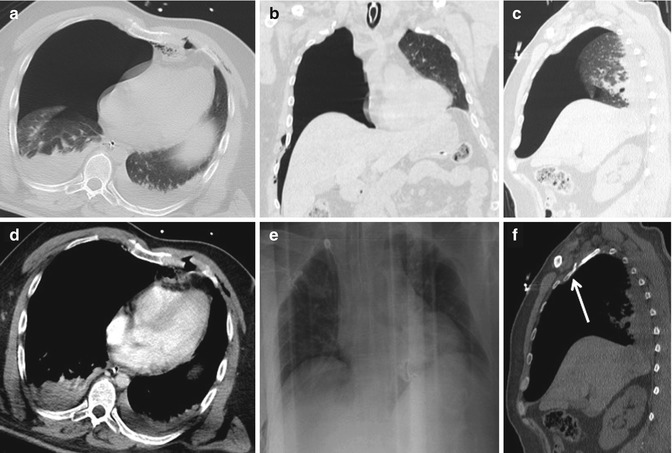
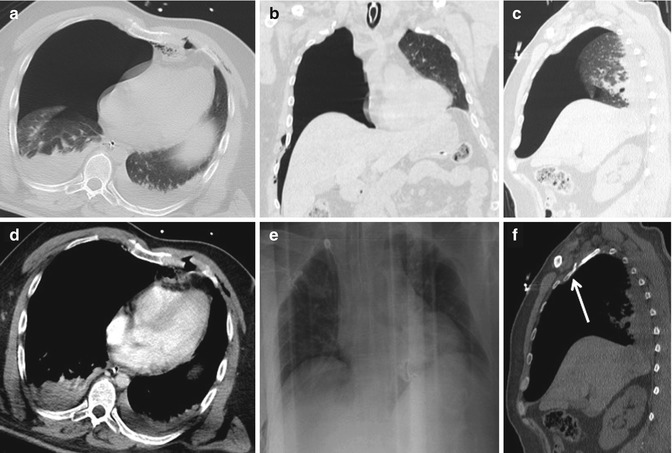
Fig. 8.7
Tension pneumothorax: axial (a–d), coronal (b), and sagittal (c) CT-scans show a massive-right pneumothorax associated with contralateral mediastinal shift collapsed ipsilateral and contralateral lung and hyperexpanded ipsilateral hemithorax. It is also visible a rib fracture in (f) (arrow), sagittal bone-window. Chest X-ray control after drainage positioning (e)
8.3.2 Hemothorax
Hemothorax represents blood in the pleural space. It is seen in 50% of blunt thoracic trauma [19] with many possible bleeding sources including pleura, chest wall, lung, mediastinal structures, and diaphragm [20]. The main reported sources of bleeding are intercostal vessels (in rib fractures) and lung parenchyma which, respectively, represent the 50 and 30% of sources [20]. In the left hemothorax, we must also be aware that the 15% of blood is derived from the aortic isthmus [20].
Chest X-ray is scarcely sensible in the depiction of pleural effusion in the supine position, as it has been demonstrated that between 50 and 100 cm3 of pleural fluid can be detected on upright chest radiograph while about 170–200 cm3 are usually necessary for detection when the radiograph is taken with the patient supine [30]. Chest X-ray, however, can diagnose complete opacification of a hemithorax that, in unstable post-traumatic patients, is highly suggestive of massive hemothorax, actually defined as a pleural collection of blood exceeding 1 L with clinical signs of shock [31] and so a life-threatening condition [31] (Fig. 8.8).
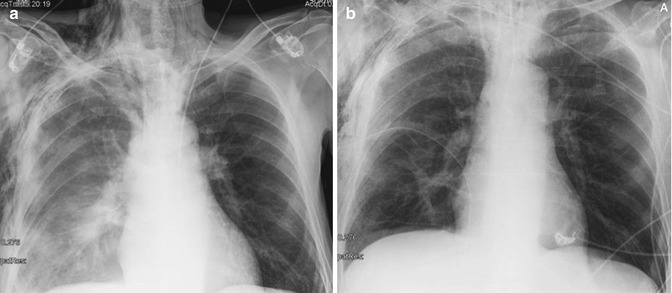
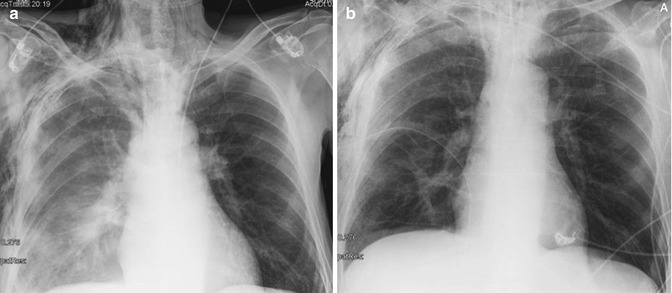
Fig. 8.8
Chest X-ray (CXR) in (a) shows an opacification of the right hemithorax caused by a massive hemothorax; subcutaneous emphysema is associated. CXR control after drainage positioning (b)
Following the actual international evidence-based recommendations for point-of-care lung ultrasound [17], the optimal site to detect a free pleural effusion is at the posterior axillary line above the diaphragm and the main standardized US signs to look for are a space (usually anechoic) between the parietal and visceral pleura and respiratory movements of the lung within the anechoic space. It has been demonstrated that US is an accurate methodic not only to detect even small pleural effusions but also to differentiate its nature [32]. In fact, visualization of internal echoes, mobile particles, or septa is highly suggestive of hemothorax. Unfortunately, if depicting anechoic fluid, US alone can’t differentiate between pleural effusion and hemothorax (Fig. 8.9) [33].
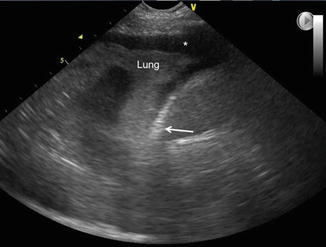
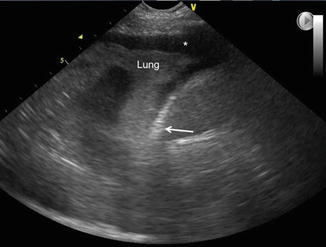
Fig. 8.9
US hemothorax. The optimal site to detect a free pleural effusion is at the posterior axillary line above the diaphragm (arrow) and the main standardized US signs to look for are: a space (usually anechoic) between the parietal-visceral pleura and respiratory movements of the lung within the anechoic space (asterisk). It has been demonstrated that US is an accurate method not only to detect even small pleural effusions but also to differentiate its nature. In fact, visualization of internal echoes, mobile particles or septa, is highly suggestive of hemothorax. Unfortunately, if depicting anechoic fluid, US alone can’t differentiate between pleural effusion and hemothorax
Although it has been stated that even very small quantities of fluid can be detected with ultrasound, with one study estimating that as little as 20 cm3 of pleural fluid can be visualized [30], a recent meta-analysis pursued in 2016 [34] showed that the sensitivity of US in detection of hemothorax is higher than radiography but it is still at a moderate level (67%), while the specificity of both imaging modalities was found to be very high. In this review, the performance of US was influenced, in the different studies, mainly by the operator skill and by the transducer frequency [34].
MDCT readily characterizes pleural fluid in the setting of trauma and can show even subtle fluid collections [8]. As blood may not be the only fluid encountered in the injured pleural space, with serous effusion and chyle being other possibilities, attenuation measurement may be employed to try to differentiate the various entities although this is not a simple effort due to the frequent coexistence of various fluids in the pleural space with different proportion [2]. However, all the authors recommend that measurement of attenuation of pleural space fluid should be routinely performed in the appropriate setting [2]. Usually, a mean density <15 HU is indicative of serous effusion, liquid blood attenuation typically measures 30–45 HU, and clotted blood is 50–90 HU. A mixture of serous and blood fluid can present as a homogeneous fluid with an intermediate density or as a very inhomogeneous one where hyperdense areas or stripes can be seen in the contest of a more hypodense fluid collection, usually the first located in the depending regions of the pleural space [2].
It is mandatory to perform an angio CT of thoracic aorta in order to identify active intrapleural bleeding and to differentiate arterial bleeding from venous one. Arterial hemorrhage is characterized by rapidly increasing hemothorax volume, mainly with hyperdense fluid, with depiction of active arterial bleeding by extravasation of contrast material into the pleural space in the arterial phase, having density similar to that of the contrast-enhanced arterial vessels [3] (Fig. 8.10). In active venous hemorrhage, you can find the presence of contrast media into the pleural space in the venous or delayed phase of the contrast-enhanced examination with the extravasated contrast media showing density values similar or inferior to venous vessels. When an active bleeding is suspected, a delayed acquisition at 5 min is highly recommended, provided that the patient’s hemodynamic stability allows for it [3] (Fig. 8.11).
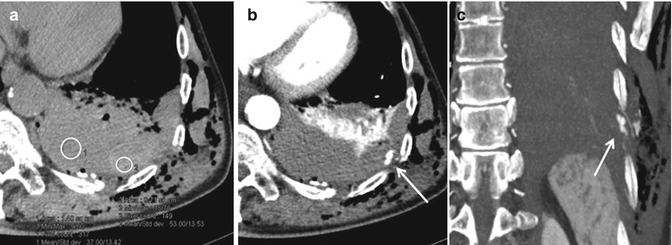
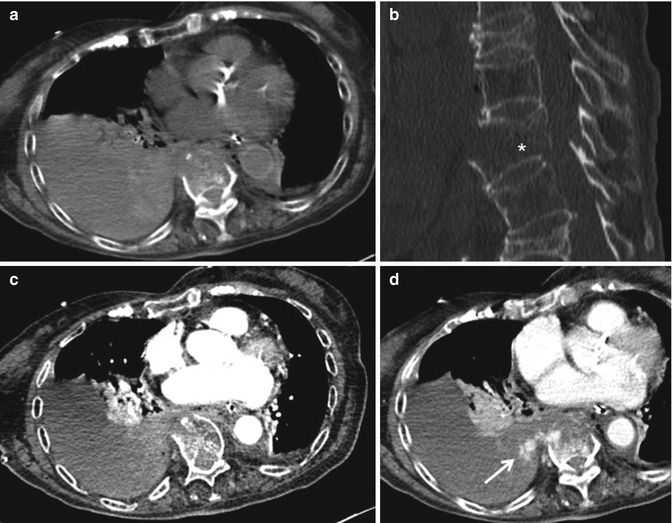
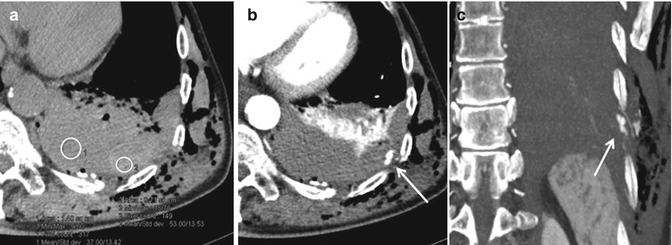
Fig. 8.10
Hemothorax. Axial CT-scan at baseline (a) shows a posterior pleural fluid collection. As blood may not be the only fluid encountered in the injured pleural space, measurement of the attenuation of pleural space fluid collection should be performed. In (a), the pleural fluid is homogeneous with a marginal hyperdense area: one roi (red circle) shows an attenuation of 37 HU (liquid blood attenuation measures 30–45 HU) and another roi (yellow circle) has 53 HU (clotted blood is 50–90 HU). Angio-CT is performed (b, c) and shows an extravasation of contrast material, proving the active arterial bleeding from an intercostal artery (arrows)
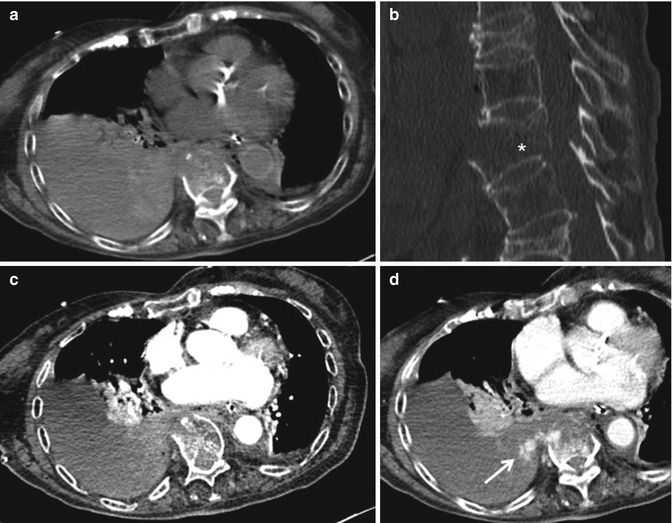
Fig. 8.11
Right hemothorax with active venous bleeding: axial CT-scan at baseline (a) shows an inhomogeneous fluid collection with iso- and slightly hyperdense coexisting areas. No extravasation of contrast media can be seen in arterial phase (c). The venous phase in (d) proves the active blood supply from a lumbar artery (arrow), in a vertebral fracture, (b) (asterisk)
8.4 Lung Injuries
The spectrum of pulmonary injuries consequent to thoracic blunt or open trauma consists in pulmonary contusion, pulmonary laceration, and pulmonary extrathoracic herniation [2].
8.4.1 Lung Contusion
It is the most common type of lung injury in blunt chest trauma with a reported prevalence of 17–70% [35].
Although the pathophysiology of pulmonary contusion is complex and poorly understood, three possible mechanisms have been hypothesized [36, 37]:
- 1.
Inertial effect: the lighter alveolar tissue is sheared from the heavier hilar structures due to different tissue densities and therefore different rates of acceleration or deceleration.
- 2.
Spalling effect: lung tissue bursts or is microsheared where a shock wave meets the lung tissue at interfaces between gas and liquid.
- 3.
Implosion effect: it occurs when a pressure wave passes through a tissue containing bubbles of gas; the overexpansion of gas bubbles stretches and tears alveoli walls [36] with no macroscopic disruption of pulmonary parenchyma.
These injuries damage alveolar capillaries, so blood and other fluids accumulate in the alveolar spaces. Then, the different blood components entering the lung tissue most likely cause the release of multiple inflammatory factors which act as starter for many processes as damage to type II pneumocytes and enrollment of many flogistic cells and molecules into pulmonary parenchyma [36].
Recently, studies on rats demonstrated a rising level in bronchoalveolar lavage (BAL) of inflammatory cellules and molecules. They all decline to baseline in about 1 week [38]. This inflammatory response probably leads to multiple events including endoalveolar and endobronchial mucus production. Aufmkolk et al. in 1999 and Raghavendran et al. in the first 2000s [39, 40] reported some abnormalities in surfactant lipid composition in BAL from trauma patients which reflect type II cell injury or dysfunction. Such surfactant activity deficits were most severe 24 h after contusion and returned to normal state over 48–96 h in parallel with improving lung parameters. Furthermore, tracheal instillations of bovine surfactant in rats with trauma-induced pulmonary contusion improve pulmonary function at 24 h post-injury [40] and surfactant therapy was to be found beneficial in suine with unilateral induced lung contusion [41].
So, contusion has been proved to be sustained by multiple, non-completely known, mechanisms, including alveolar and interstitium filling with hematic and non-hematic fluids, flogistic activation (which can be responsible also for systemic effects as fever or contralateral pulmonary edema), and surfactant abnormalities. All these mechanisms lead to a precocious lung dysfunction which rapidly tends to resolve, usually with complete restitution [36, 37].
The principal complications of lung contusion are ARDS and pneumonia [37]. ARDS develops in 17% of patients with isolated pulmonary contusion, while 78% of contusions with additional injuries develop ARDS [42]. Globally, significant pulmonary contusion leads to ARDS in 50 to 60% of cases while pneumonia develops in about 20% of cases [43].
Systemic activation of neutrophils after trauma is likely to be the leading cause of acute respiratory distress syndrome and multiple organ failure in post-contusion hospitalization [44, 45].
Lung contusion is also a well-known risk factor for development of pneumonia. However, the reason for this increased susceptibility is not clear. It has been supposed that acute lung injuries of lung contusion create in the local pulmonary immune system a sort of vulnerability from a “second” hit bacterial infection [46].
The respiratory impairment of lung contusion includes ventilation/perfusion mismatching, increased intrapulmonary shunting, increased lung water, segmental lung damage, and loss of compliance [36, 37]. Clinically, patients with pulmonary contusion present very unspecific signs as hypoxemia, hypercapnia, and dyspnea [36, 37].
In lung contusion, findings on chest radiography are nonspecific, varying from irregular, patchy areas of opacification to diffuse and extensive homogeneous hypolucency. These changes are usually evident within 6 h after trauma and resolve rapidly, typically within 3–10 days [2, 7, 37]. The scarce specificity of pulmonary post-contusion changes on chest X-ray suggest clinicians to refer as pulmonary contusion every opacification seen on radiograms in post-traumatic patients with the reported time evolution, until proven otherwise [36, 37].
As already stated, lung pulmonary changes in lung contusion consist mainly in three phases characterized, respectively, by: a precocious hematic or serous flooding of alveolar cavities, an intermediate (1–2 h after the traumatic event) edematous infiltration of lung interstitium and a successive flooding of air spaces with inflammatory cells associated with local surfactant deficiency [47]. This last phase, occurring from 6 to 24 h after the trauma and developing in consolidation, is the one visible on chest X-ray [7]. As it has been demonstrated the US accuracy in diagnosing pulmonary interstitial edema [48], we can assume that US is able to depict pulmonary contusion changes in the interstitial phase, earlier than chest X-ray. A study by Soldati et al. [47] confirmed this statement as demonstrated a sensibility of an admission ultrasound examination of 94.6% and a specificity of 100% (in the selected trauma population) compared to CT as gold standard. This specificity cannot be reached in a clinical population, since there are other lung diseases that show the same US patterns, and although US is able to recognize different alterations in lung parenchyma, its specificity in a general population is not so high [47].
As a bedside technique, lung US is performed focusing on the anterior and lateral walls and on the most posterior accessible region beyond the posterior axillary line, not compromising patient immobilization in supine position [18]. The US signs for lung contusion [17] are: the presence of the gliding sign (that is generated by the lung parenchyma movement under the parietal pleural and that indicates the absence of pneumothorax or pleural effusion) associated with the presence of an alveolo-interstitial syndrome defined as the presence of multiple B-lines, in a patient with no clinical suspicion of cardiogenic pulmonary edema, or peripheral parenchymal lesions defined as subpleural echo-poor (or with tissue-like echotexture) regions, allowing ultrasound transmission, from which B-line-like artifacts arise [17, 47]. Lower frequency ultrasound scanning may allow for better evaluation of the extent of a consolidation [17] (Fig. 8.12).
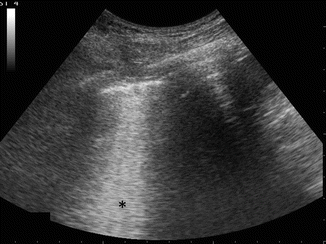
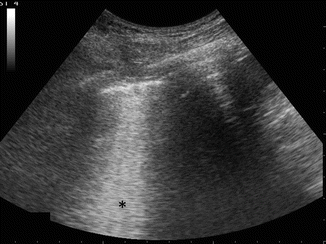
Fig. 8.12
Lung contusion: US image shows an early pulmonary contusion, which was not yet detectable with a standard chest X-ray. The interstitial involvement is shown by the vertical hyperechoic lines, the so-called B-lines (asterisk)
In a recent article [49], the diagnostic accuracy of US was compared to that of combined clinical examination and chest radiography for pneumothorax, lung contusion, and hemothorax, with thoracic CT-scan as reference. It tested also the ability of an US score, measuring the extent of lung contusions, to predict ARDS. It confirmed the major accuracy of US than that of combined clinical examination and chest radiography for all the three injuries, and it demonstrated that the suggested US score can identify patients at risk of developing ARDS after blunt trauma [49].
The more frequent MDCT findings of contusion consist of poorly defined, generally non-segmental areas of consolidation and ground glass alterations [2] sometimes (especially in children) sparing 1–2 mm of subpleural lung parenchyma adjacent to the injured chest wall [43]. Contusion areas generally do not show air bronchograms as the bronchioles are often filled with fluid. These signs are well visible with MDCT, unlike chest X-ray, immediately after the traumatic event though it reaches the major increment within the first 6–24 h [19, 37, 50] (Fig. 8.13). As in chest X-ray, clearance of an uncomplicated contusion in MDCT begins at 24–48 h with complete resolution after 3–14 days [2] (Fig. 8.14).
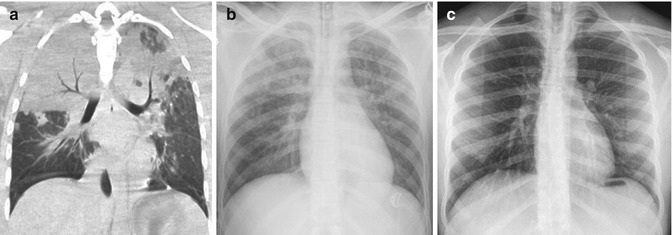
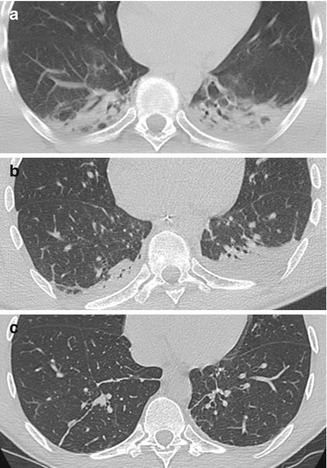
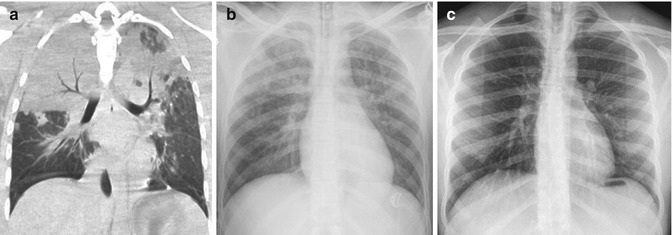
Fig. 8.13
Bilateral parenchymal contusion. Coronal CT-reconstruction immediately after trauma (a) shows large parenchymal consolidations. Chest X-ray (CXR) acquired 2 h after the trauma (b) shows patchy areas of opacification in the upper lobes. CXR after 14 days shows a complete resolution (c)
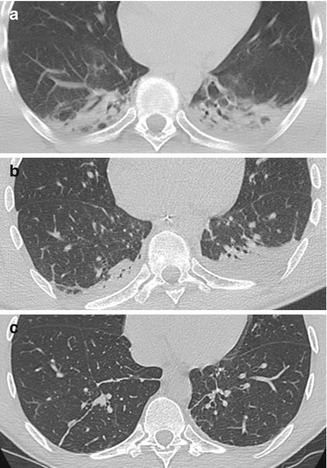
Fig. 8.14
Contusion of the posterior-lower lobes (a), that improves in the controls after 5 (b), and 10 days (c)
Despite the high sensibility, MDCT is poorly specific so in clinical practice, any kind of densitometric increment in lung parenchyma that shows the same temporal pattern is related to contusion damage (Figs. 8.15 and 8.16). At the same time, any kind of parenchymal increase in density arising after 24 h from the traumatic event should be referred to other lung injuries rather than contusion (the more frequents, in a post-traumatic setting, being pneumonia or aspiration) (Fig. 8.17). On the other hand, any kind of lung alterations that do not resolve within a week in a traumatic patient should be regarded as contusion complications, in particular as infection or ARDS [2, 27] (Fig. 8.18).
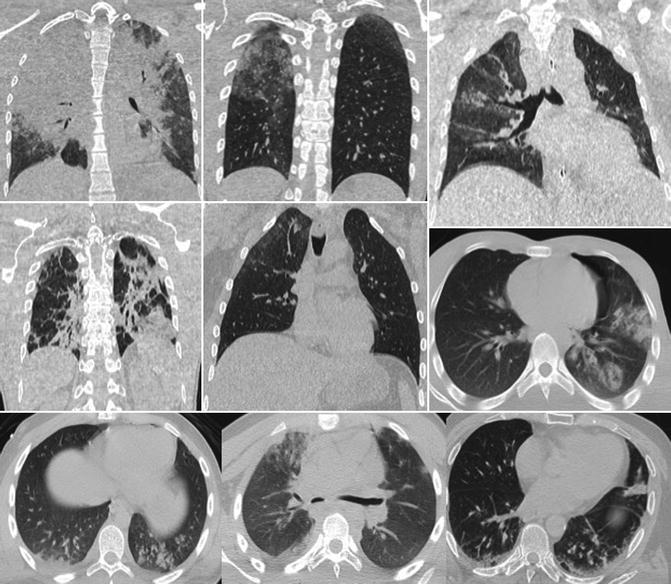
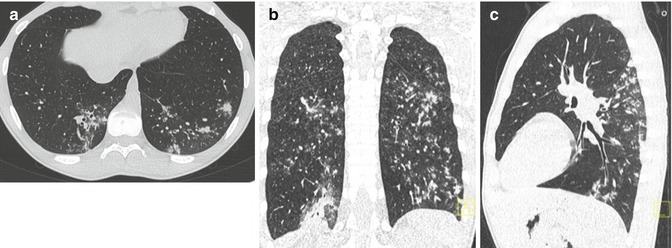
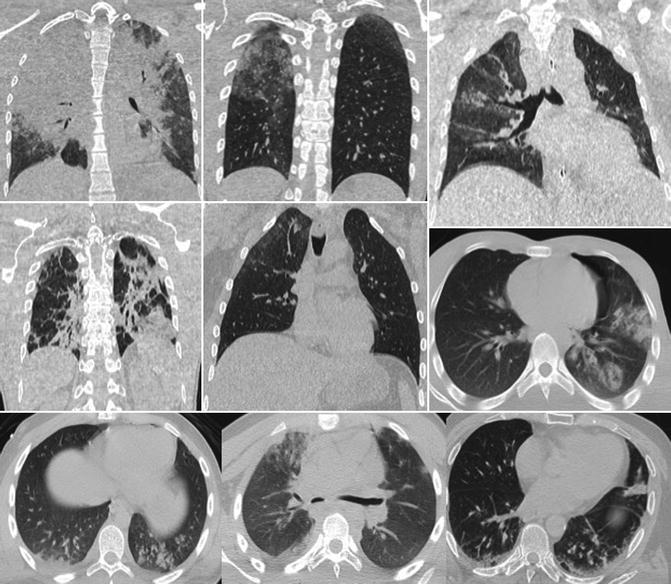
Fig. 8.15
Several examples of pulmonary contusion: it generally consists of poorly defined and non-segmental areas of consolidation and/or ground glass alteration adjacent to the injured chest wall. As we can see, the heterogeneity of lung contusion doesn’t permit a differential diagnosis based only upon CT-scan aspect. Thus, every consolidation or ground glass alteration seen on CT-scan after a thoracic trauma should be considered pulmonary contusion until proven otherwise
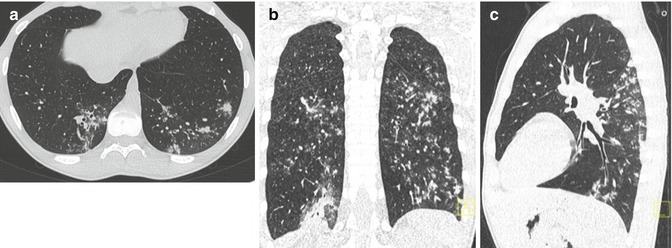
Fig. 8.16
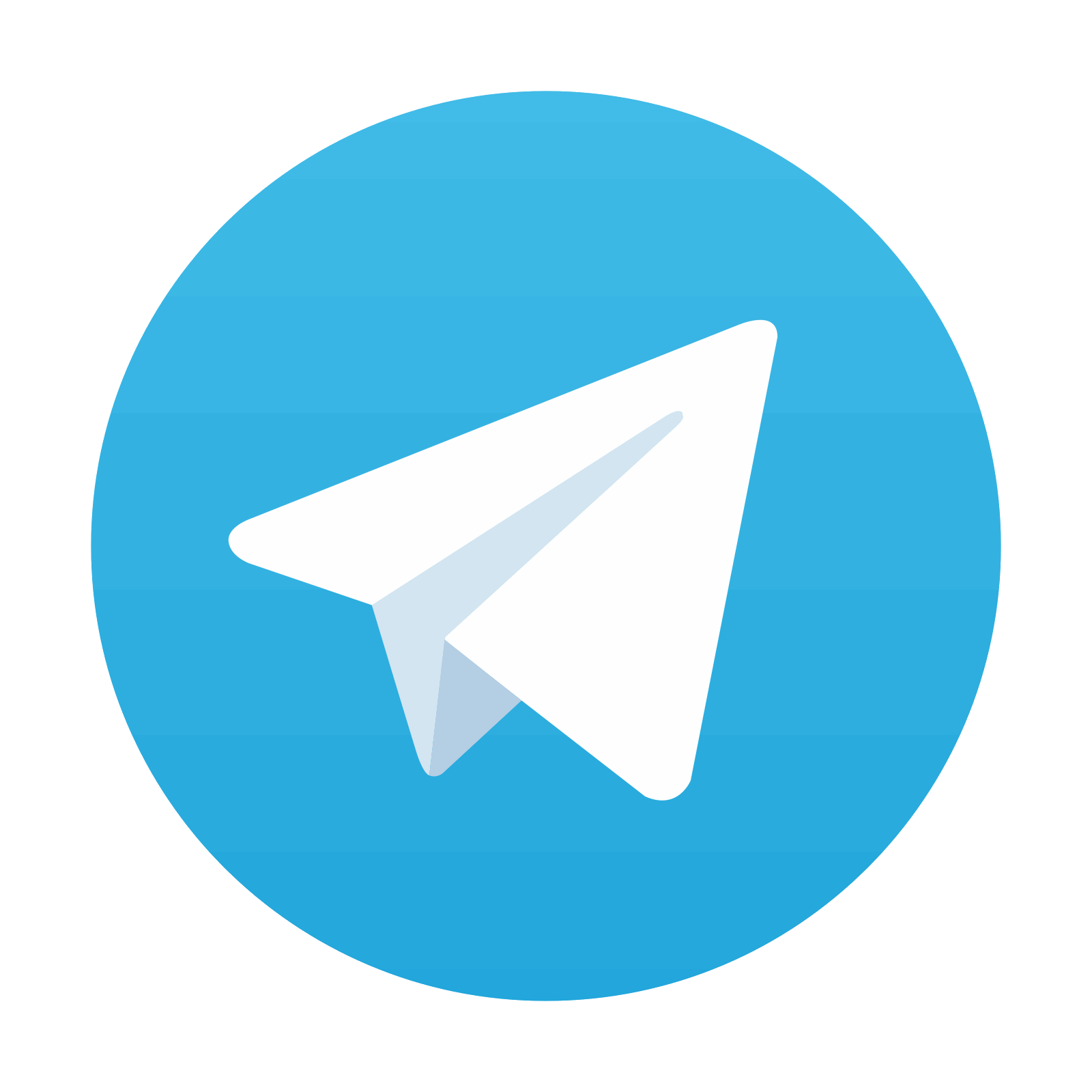
Atypical contusion areas in the posterior segments of the inferior lobes. Post-traumatic CT-scan in axial (a), coronal (b), and sagittal (c) reconstructions. Their clarification in less than 10 days from the traumatic event suggested the hypothesis of pulmonary contusions unless their atypical aspect
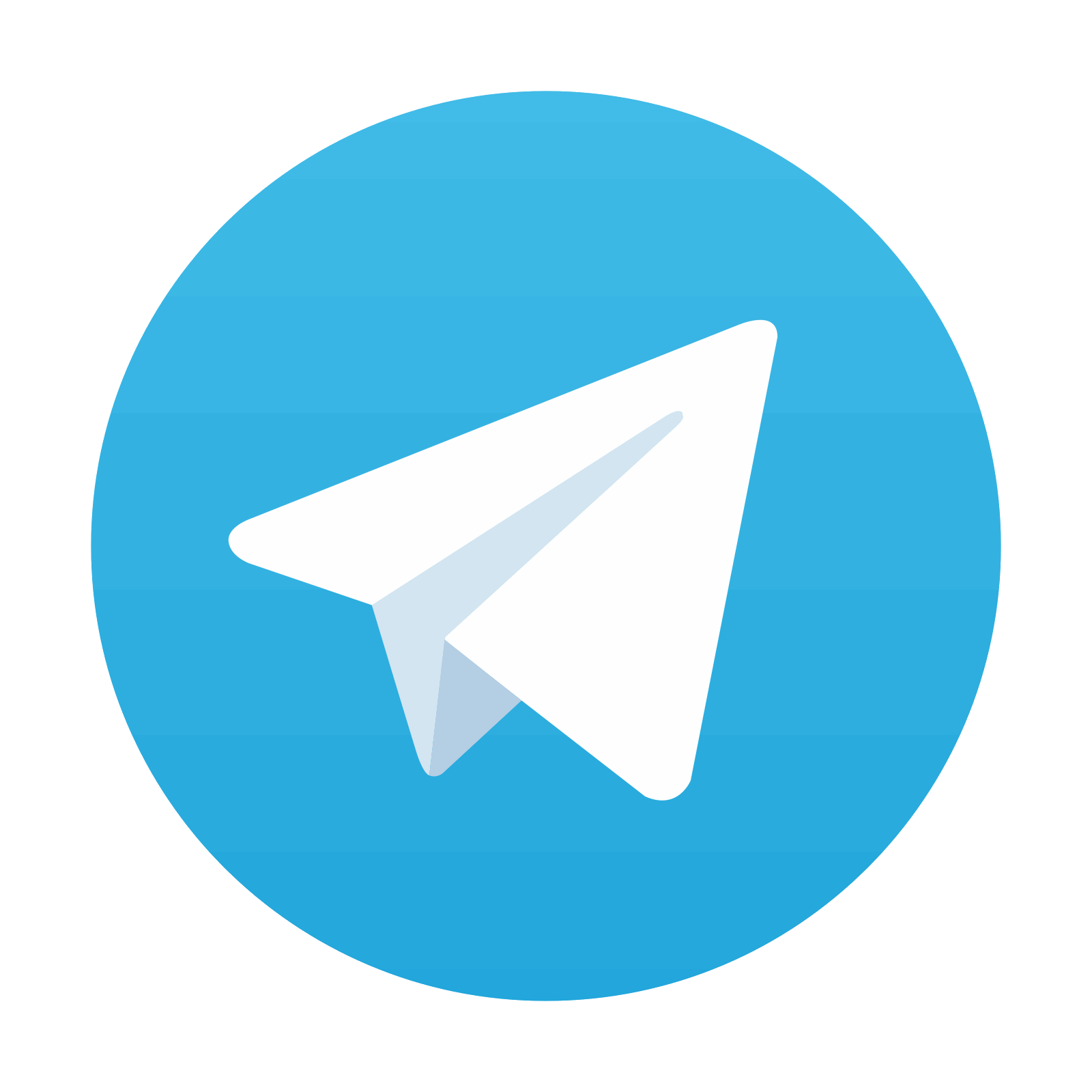
Stay updated, free articles. Join our Telegram channel

Full access? Get Clinical Tree
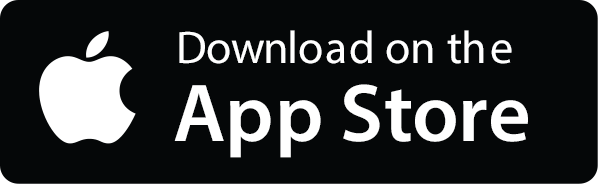
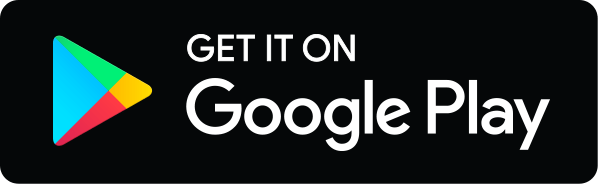
