Introduction
The demand for pediatric magnetic resonance imaging (MRI) is steadily increasing because MRI is radiation free, has exquisite soft tissue contrast, and provides quantitative structural, functional, and vascular information for diagnosing a wide spectrum of diseases. Furthermore, the advent of genomics has led to improvements in understanding the genetic basis of a variety of heritable disorders associated with childhood neoplasms, so serial laboratory and radiologic screening protocols in patients with cancer predispositions (e.g., Beckwith-Wiedemann syndrome, von Hippel-Lindau disease, Li-Fraumeni syndrome) are established. Computed tomography (CT) and whole-body F-fluorodeoxyglucose positron emission tomography/CT (FDG-PET/CT) are common surveillance modalities. However, these modalities expose children to ionizing radiation, and cumulative radiation doses from these relatively low-dose individual imaging studies may increase their lifetime cancer risk. A retrospective cohort analysis focusing on radiation exposure from CT scans in 178,604 pediatric patients found children with cumulative doses of over 50 mGy to the red bone marrow and 60 mGy to the brain (≈2-3 head CT scans) to be about three times more susceptible to developing radiation-induced leukemia and brain cancer, respectively. In addition, given the possible radiation sensitivity of patients with cancer syndromes such as Li-Fraumeni syndrome or ataxia-telangiectasia, localized and whole-body MRI may be a promising alternative for cancer prescreening and surveillance in children, thereby making every reasonable effort to avoid or limit ionizing radiation as far below dose limits as practical (ALARA [“as low as reasonably achievable”]).
Although MRI has been extensively used in adult imaging for decades, MR knowledge and methodology are not directly transferable to pediatric imaging, because “children are not just small adults.” There are both practical and technical challenges when imaging infants and children. These include anatomic differences, developmental changes, differences in physiologic parameters, different spectrum of diseases, challenges in holding still for long periods of time, patient cooperation, availability of pediatric equipment (e.g., smaller-sized radiofrequency [RF] coils, MR-compatible incubators), and specific absorption rate (SAR) issues. Also, MRI requests may be nonspecific because children are poor reporters and often describe their symptoms very vaguely. All these factors could render pediatric MRI exams potentially complicated, costly, and lengthy. Nonetheless these problems can be overcome by proper patient preparation and optimization of techniques to obtain diagnostic image quality.
This chapter gives an overview of pediatric MRI focused on techniques and parameters that differ from adult imaging. Topics include preparing and coaching a pediatric patient, special equipment, addressing safety concerns, optimizing signal-to-noise ratio (SNR) and contrast, techniques to minimize motion artifacts, standard imaging protocols, and emerging techniques in pediatric MRI.
Patient Preparation
Adequate patient preparation before the procedure is very important in reducing a child’s anxiety and promoting his/her cooperation during the MR examination. Developing a trusting relationship with the parent or guardian is key to successful pediatric imaging. Preprocedural preparation for the pediatric patient begins with a brief medical history describing the problems that warrant the MR examination. The patient or parent of a minor patient fills out a screening form indicating whether the patient has had any surgical interventions, including implanted prostheses, or if the child has experienced any accidents that would have resulted in embedded metal. If the patient has an implant, its MR compatibility must be determined before the MR examination; “the list” ( www.mrisafety.com ) is a convenient resource for this purpose. Furthermore, the staff member conducting the screening should actively gather information from both parent and child, because the child could have items stuck in the ear or nose, unknown to the parent.
For patients who may need sedation, the parent is also asked whether the child has ever experienced any symptoms that relate to certain conditions that may increase the risks of sedation. A history of upper airway obstruction, chronic liver disease, mental retardation, and congenital heart disease are some clinical conditions that may indicate an increased risk of sedation or risk of sedation failure. If a patient has an acute illness (e.g., unexplained fever, upper respiratory tract infection), an elective or nonurgent MR examination should be temporarily postponed. To minimize the risks of aspiration, pediatric patients should abstain from eating and drinking for a designated time period prior to sedation. According to NPO (nothing by mouth) guidelines for intravenous (IV) sedation, patients can have clear liquids up until 2 hours, breast milk up until 4 hours, infant formula up until 6 hours, and solid food and any other liquids up until 8 hours before the procedure. For oral sedation, patients typically can have solid food up until 4 hours and clear liquids up until 2 hours before the procedure.
Sedation
Children referred for MRI often feel anxious or distressed about the unknown, unfamiliar surroundings and long examination times (on average, ≈ 45 min), and they may also be biased by their parents’ anxiety. Children who have had an MRI before may still be afraid of the large and noisy machine, confined space, the need to lie still, discomfort from surface coils, and the possibility of IV contrast injection. Given these factors, many children are unable to cooperate and require sedation. To successfully complete the study, sedation is generally recommended for children younger than age 6 to 8 years and for older children with behavioral problems. However, establishing definitive age limits for sedation is not recommended; the need for sedation should be decided on an individual basis.
Sedation Agents.
The American Academy of Pediatrics (AAP) has published guidelines for sedation and monitoring of children undergoing imaging studies. The most common drugs used for sedation in pediatric MRI are propofol, dexmedetomidine, chloral hydrate, and pentobarbital. Propofol is often preferred for pediatric sedation because it is effective, has short induction (2 minutes) and recovery times (8 minutes), can easily be titrated to the required level of sedation, and has low incidence of complications. Typical propofol doses for sedation are 2 to 5 mg/kg/h IV. Dexmedetomidine is an alternative sedative for MRI with less respiratory side effects compared to propofol. It is administered at a loading dose of 2 to 3 µg/kg over 10 minutes, followed by a 1 to 2 µg/kg/h maintenance infusion for sedation. However, dexmedetomidine may have side effects such as bradycardia and hypotension and is therefore unsuitable for patients with compromised cardiac function. Chloral hydrate is another widely used sedative drug with barbiturate-like features. The dosing is 25 to 100 mg/kg, the onset time is 15 to 30 minutes, and duration of effect is 60 to 120 minutes. However, this drug has side effects such as nausea, vomiting, long recovery times, and postoperative agitation that lead to high failure rates for its use in MRI. Pentobarbital is another short-acting barbiturate and is given either orally or rectally at 3 to 6 mg/kg, with an onset time of 15 to 60 minutes; the duration of effect is 60 to 240 minutes. However, its use is restricted because it may be associated with respiratory and cardiovascular depression.
Although a variety of sedative drugs are available, one should have a clear understanding of the pharmocokinetic effects of each agent when choosing the most appropriate drug for a particular patient and scenario. Institutional guidelines are usually in place for the selection and administration of effective and safe sedation in children during radiologic procedures. In pediatric MRI, establishing high sedation success rates largely depends on (1) filtering out children in whom sedation is unsafe or will likely be unsuccessful, (2) administering appropriate drugs and dosages, (3) continuously monitoring vital parameters, and (4) having experienced staff on site for immediate resuscitation.
Monitoring During Sedation.
Every child undergoing sedation during an MR examination should be continuously monitored with MR-safe or MR-conditional equipment. Blood pressure, pulse rate, oxygen saturation, respiratory rate, electrocardiogram (ECG), and temperature are the parameters commonly monitored. Of these, the most reliable and immediate indicators of patient distress are increase in pulse rate and decrease in transcutaneous oxygen saturation, because the most frequent complications of sedation are related to respiratory depression or airway obstruction. All patients should be monitored not only during the procedure but also following the procedure until postsedation recovery is complete.
Imaging Without Sedation
Sedation is frequently needed in pediatrics because some children are unable to lie immobile for the period of time needed to complete an MR study. Though often necessary, sedation is not without risk. Longer sedation is needed for MRI, owing to longer scan times that can put the child at risk for respiratory depression with oversedation. Patients on a ventilator or those who hypoventilate are at risk for atelectasis. Figure 6-1 shows images of a sedated patient who developed partial atelectasis in the left lung within 20 minutes. A potential issue with opacities caused by atelectasis is that they may obscure underlying pathology such as metastatic disease or sites of infection. Sedation services are also costly, requiring a team of pediatric anesthesiologists, intensivists, emergency department pediatricians, and pediatric nurses who work to ensure a safe procedure. Hence imaging without sedation could improve patient safety and reduce associated costs. Some techniques that have been shown to be effective in improving patient compliance and hence image quality without the need for sedation follow.
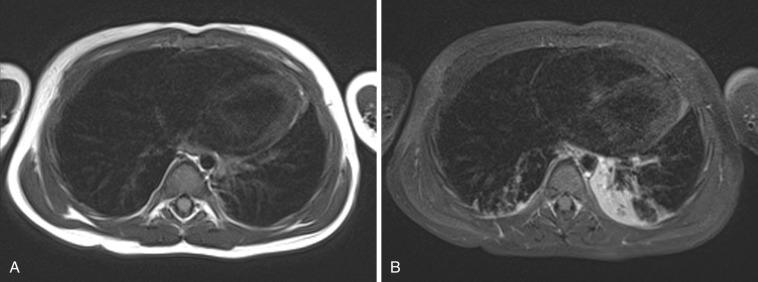
Feed and Sleep.
Feed and sleep is a technique in which sleep is induced in very young children through proper feeding and by providing warmth and comfort. This technique is routinely used in infants younger than 3 months of age undergoing MR examination ( Fig. 6-2 ). The infant is made to fast for 4 hours and then fed until satisfied at least 30 minutes before the scan time. After the child goes to sleep or settles down, he/she is swaddled using infant sheets and secured in an immobilization device, sound protection is applied, and then the child is taken into the scan room escorted by a parent or a guardian. In one study the feed-and-sleep approach with immobilization devices enabled 32 of 36 infants to successfully complete MRI scans with good image quality and without any sedation. Another study by Nordahl et al. showed good success rates in acquiring diagnostic-quality MRIs without the use of sedation in autistic children aged 2.5 to 4.5 years by scanning them in the evening during their normal bedtime.
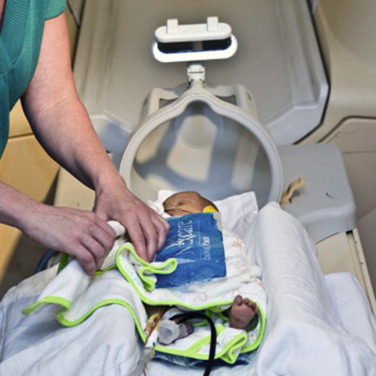
Communication and Positive Reinforcement.
Professionals who work with children can give reassurance and raise confidence in young patients to undergo the procedure without the need for sedation through verbal communication and body language. Positive reinforcement is also an effective way to keep children motivated to complete the scan successfully; reinforcement can be through verbal praise, certificates, trophies, and other prizes appropriate to the child’s age. Rewarding or praising pediatric patients for their efforts at each step is very helpful in gaining and maintaining cooperation and keeping them immobilized during the procedure.
Child Life Specialist.
In recent years, child life specialists (CLSs) have become an integral part of the imaging team. Their knowledge and ability to relate to parents and to children of different ages and levels of maturity can mean the difference between successful and unsuccessful imaging, and the difference between the need for sedation and completing a study without sedation. CLSs are positioned to educate patients and parents about an imaging study prior to the child presenting for the examination, thereby allaying anxiety and enhancing patient cooperation. The CLS may intervene prior to the study by discussing the procedure with the patient and parent, introducing the family to the imaging team, building rapport, presenting coping skills, and using strategies such as play therapy, mock MRI, and distraction techniques in an attempt to increase the likelihood the child will be able to cooperate during the examination. During the procedure the CLS can serve as liaison between technologists, patient, and parents, engage in nonstudy talking, distract the patient, provide emotional support, and so forth.
Play Therapy and Mock MRI.
Play therapy is used to prepare children older than about age 4 years for MRI scans by explaining the procedure in a play-based manner with the help of medical toys, tape recordings, mockup scanners, or using real equipment. Showing pictures of children undergoing or having their favorite soft toys undergo an MR scan to explain what the examination entails makes a positive impact on how children will perceive the whole experience. Playing with a toy scanner model with sliding table and listening to an audio recording of the MR scanner noise ( Fig. 6-3 ) help children become familiar with the procedure and develop coping strategies, allowing them to lie still and complete the scan.
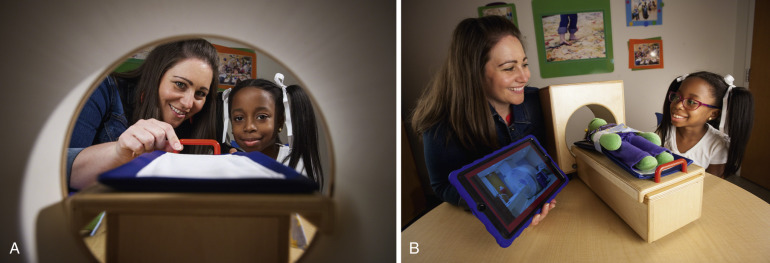
Recent studies have shown that sedation rates were considerably decreased in children exposed to a mock MRI scanner. The mock scanner room is well decorated and made appealing to children; it is usually in close proximity to the clinical MRI room ( Fig. 6-4 ). In the mock scanner the child experiences the same sounds and visuals he/she will experience during the real examination, such as lying on the table within the bore, having coils placed, hearing the sounds when sequences are played, and sometimes even rehearsing breath holds and developing coping strategies for IV contrast administration. This helps children cope with stressful conditions and makes the real experience less intimidating and frightening. A recent study showed that adequate play therapy in combination with a mock MRI session greatly improved patient cooperation and facilitated clinical MRI scans without sedation in 110 (84%) of 131 patients.
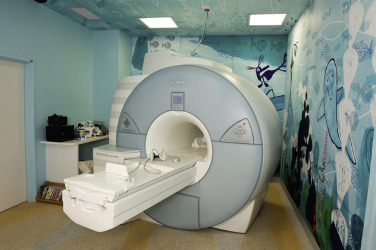
Distraction.
Stress during MR procedures can be effectively reduced in pediatric patients by using distraction strategies. Simple physical contact between a parent and child (e.g., parent placing a hand on the child’s hand or foot) is very useful in allaying fear. Parents can bring pacifiers, MR-safe toys, books, or other comfort items that will distract the child. Making the physical environment more appealing by having a bright, colorful, and lively room with pictures of animals or characters can also distract older children and help them handle the procedure. However, young infants may prefer a warm and darkened environment that facilitates sleep.
Auditory distraction techniques like playing music and storytelling are effective ways to calm and distract pediatric patients. Watching a video during the scan is an extremely valuable distraction tool. Older video system designs projected a video onto a screen in the scanner room, which was reflected by a mirror attached to the head coil ( Fig. 6-5A ). This setup worked well for brain scans but not for body scans, because as the table moved, the screen went out of focus. Also, this setup did not allay claustrophobic feelings some children experience within small-bore magnets. Modern commercial entertainment systems use MR-compatible video goggles that do not interfere with table movement and completely enclose the patient’s eyes, thereby minimizing claustrophobia (see Fig. 6-5B ). It has been shown that the use of MR-compatible video goggles greatly reduces the need for sedation and provides a smoother workflow while obtaining good-quality images.
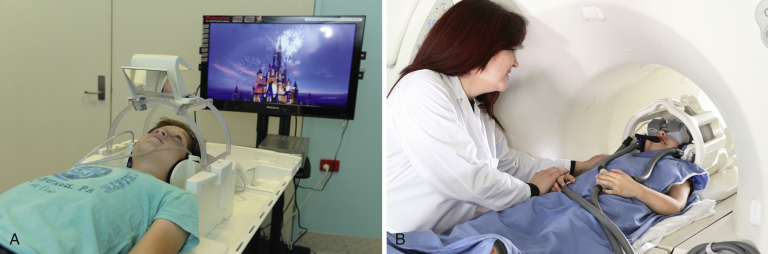
Open MRI.
Traditional MRI scanners have long, narrow horizontal tubes into which the patient slides until the targeted body part is positioned in the center of the magnet. Such a closed environment can be daunting for pediatric patients and add to their anxieties and fears. Open MRI scanners have been designed to mitigate claustrophobic feelings. Several open magnet types are on the market. In the “pancake” design, the poles of the magnet are arranged horizontally, making the magnet three times larger and open on all sides. This allows parents to lie next to their child in the magnet, hold their child’s hand, and/or maintain eye contact during the scan, which can reduce anxiety for both child and parent and ultimately eliminate the need for sedation. Other designs include traditional magnets with shorter bore length and larger diameter, a so-called double-doughnut design with an open space in the middle, and a standup MRI configuration that allows patients to stand or sit. Historically, image quality was a problem with open MRIs because of their low magnetic field strength (0.2-0.35 tesla [T]). New open MRI technology has both open design and field strength of 1 T or higher, with images of the same quality as traditional scanners.
Safety
MR Screening
Compared to adult scans, where typically only a technologist and the patient enter the magnet room, a relatively large number of people (e.g., parents/guardians, child life personnel, sedation/anesthesia team) may enter for pediatric scans. If someone accidentally carries any ferrous object into the magnet room, it may become a projectile owing to the strong forces from the static magnetic field. Although nonferrous metals are not attracted by the magnetic field, they may experience Lenz’s forces either due to rapid switching of gradients or when moved in the static magnetic field. Moreover the strong static field or the high RF power may cause electronic equipment to fail. RF fields inside the magnet bore can induce currents in conducting structures such as wires, electrode leads, or even sweaty skin, which in turn can induce eddy currents in the body that may cause burns in the patient. Hence everybody needs to be screened for medical conditions or for metal items inside or on the body, especially for pacemakers and implants, before entering the magnet room. Generally each individual entering the MR room must complete a screening form as part of the screening process, as previously mentioned. The American College of Radiology (ACR) has published extensive guidelines on MR safety, and all MR personnel should be trained and follow MR safety guidelines to avoid any MR-specific risks. These risk-management and training requirements are now also demanded by The Joint Commission.
Equipment
All equipment used in the MR suite needs to be labeled according to the scheme proposed by the U.S. Food and Drug Administration (FDA) :
- 1.
MR safe: A square green label indicates that the item is “MR safe” and can be used in the MR environment and poses no known hazard. Items include nonmetallic, nonmagnetic, and nonconducting objects such as plastic or wood.
- 2.
MR conditional: A triangular yellow label represents that the item is “MR conditional” and has been tested and demonstrated to pose no known hazards in a specific MR environment (e.g., maximum static magnetic field, gradients, SAR) under specified conditions of use. The conditions of use need to be known by the person introducing the item into the scanner room and handling it.
- 3.
MR unsafe: A round red label indicates that the items (i.e., ferromagnetic objects) are “MR unsafe” and known to pose a direct threat to persons and equipment in the scanner room and must not be brought into the scanner room.
Required non–MR-safe equipment like ventilators and monitoring devices can be used by placing them outside the magnet room and running nonmetallic connectors to the patient. Nowadays many MRI suites are provided with wave guides (wall openings) between the scan room and the control room, where fiber optic leads, plastic tubes, and other nonconducting leads or hoses can be fed through. Electrically conductive leads cannot be run through because they will act as antennas, compromise the RF shielding of the magnet room, lead to MRI artifacts, and also may be dangerous to the patient because of RF heating.
Typical equipment necessary for neonates or sick children follows.
Incubator.
MRI of critically ill preterm and term neonates is made feasible with the availability of MR-compatible incubators with integrated RF coils. In the neonatal intensive care unit (NICU), infants are transferred from their incubator to the MRI-compatible incubator ( Fig. 6-6 ) with all necessary equipment (IV pumps, ventilators, monitors) connected; earmuffs and RF coils are also attached. They are then transported to the MR room and scanned within the incubator. The MR-compatible incubator not only offers a safe humidity- and temperature-controlled environment to the infant at all times, but also optimizes the workflow because there is no additional transfer of the infant necessary when entering and leaving the magnet room. If an MR-compatible incubator is not available, a standard incubator can be used to transport the infant from NICU to the room adjacent to the scanner room. However, the infant will need to be taken out of the incubator for scanning. Most recently, Cincinnati Children’s Hospital pioneered an infant-sized MRI scanner ( Fig. 6-7 ) installed in the NICU ; by avoiding transportation to the radiology department, a safer way of performing MRI in critically ill and premature newborns is provided.
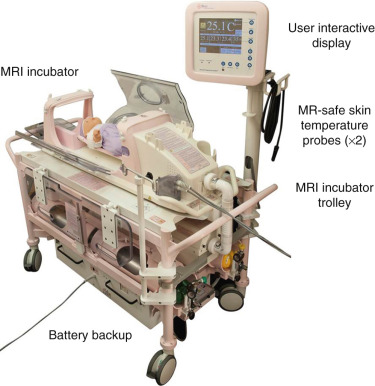
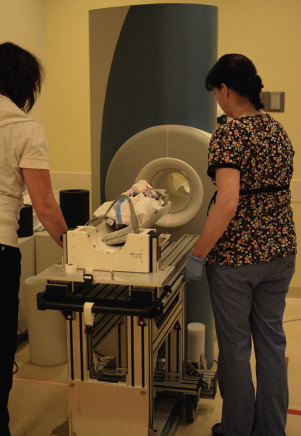
Ventilator.
Patients being ventilated should be transferred to an MR-compatible ventilator with MR-conditional gas cylinders before undergoing the examination. In situations where MR-compatible ventilators are not available, standard ventilators can be used. However, they need to remain outside the magnet room and the extended hoses are then guided into the scanner room; personnel must ensure that the ventilator is not accidentally transferred into the room.
Intravenous Pumps.
Only absolutely necessary IV solutions should be administered during MRI. The IV pumps should be replaced with MR-compatible pumps if available. Otherwise, regular IV pumps can be used by placing them outside the magnet room and running them through extended lines, as with non–MR-compatible ventilators, following the same precautions.
Resuscitation Equipment.
An emergency cart should always be available and readily accessible when pediatric patients, especially sedated ones, are undergoing MR examination. The cart must contain necessary age- and size-appropriate drugs and equipment that can resuscitate an unconscious or a nonbreathing child. This includes catheters, laryngoscopes, oral airways, positive pressure oxygen delivery system, wall suction and oxygen, portable oxygen cylinders, and suction equipment. Because most of these items are not MR-compatible, the resuscitation cart should be kept outside the magnet room but in a room adjacent to the scanner for easy access. In an emergency, the patient should be taken into the adjacent room for resuscitation in accordance with ACR recommendations for MR safety.
Ear Protection
During MRI examination, switching of the gradient fields creates loud acoustic noise, typically above the safe level of 85 dB. Prolonged exposure to such loud noise can potentially cause hearing loss, especially in children, owing to their sensitive ears. Hence noise reduction is crucial when imaging pediatric patients. There are some active and passive measures that can be implemented to reduce the acoustic noise during the MRI scan. Active measures include modification of the imaging pulse sequences to reduce imaging gradient rise times and slew rates, and to soften sharp changes in the gradient pulses. Passive measures include using a removable sound-insulating foam insert and electrodynamic headphones with embedded MiniMuff ear pads. Ear protection is not only important for patients but also for parents or staff members accompanying the patient in the magnet room. In recent years the availability of MR-compatible active noise-cancelling headphones that introduce an out-of-phase secondary acoustic wave form to cancel the sound produced by the gradients has become an effective approach for improving the safety of both patient and accompanying person.
RF Exposure
During MRI scanning, the patient is exposed to RF fields. The amount of RF energy deposited into the patient tissue during imaging is known as the specific absorption rate (SAR), expressed in watts per kilogram (W/kg). SAR depends on several factors, such as the strength of the static magnetic field, type of RF coil used, exact pulse sequence (number and type of pulses, including flip angle and waveform), patient size, and body part being imaged. According to the FDA, the maximum allowable SAR is 3 W/kg (averaged over 10 minutes) for head imaging and 4 W/kg (averaged over 15 minutes) for whole-body imaging.
Higher SAR levels increase the body temperature, causing patient heating. However, the actual increase in temperature due to RF exposure is dependent on the patient’s thermoregulatory system. Children are particularly sensitive to RF-induced heating because they have relatively immature systems for controlling body temperature, especially infants and premature neonates. Hence monitoring SAR is very important for pediatric MRI. MR manufacturers use built-in SAR models to estimate the predicted SAR for a given patient size and pulse sequence. However, because MRI scanners are primarily built for adults, adult SAR models may not be reliable for use in pediatric patients, especially infants and young children with their relatively small size and different morphology compared to adults. Kussman et al. reported that an infant developed fever (38°C) during cardiac MRI even though the predicted SAR value did not reach the SAR limit; this suggested that SAR limits may need to be revised for infants and young children.
SAR increases with the square of the magnetic field strength secondary to an increase in the RF frequency applied. For instance, there is a fourfold increase in SAR at 3 T compared to 1.5 T using the same scanning parameters, which consequently may lead to further rise in body temperatures at higher field strengths. Hence implementing measures to reduce SAR is of utmost importance in pediatric MRI. Ways to reduce SAR are to increase repetition time (TR), decrease flip angle, reduce the number of slices, use parallel imaging techniques to decrease the number of RF pulses applied, intersperse gradient echo and spin echo sequences, minimize the use of saturation bands, and use special transmit coil designs that are restricted to the body part of interest, because SAR increases with coil size. Furthermore the room should be cool and the patient should be dressed in a loose hospital gown for better heat dissipation. Infants are usually wrapped or swaddled to keep them warm and comfortable in the scanner; however, this may further impair heat dissipation. Thus body temperature should be constantly monitored in infants younger than age 1 year (e.g., via MR-compatible fluoro-optic temperature probes attached to the abdomen).
Techniques and Applications
Strategies to overcome practical difficulties in performing pediatric MRI have been addressed in this chapter, but there are also technical challenges when imaging pediatric patients: poor signal-to-noise ratio (SNR), contrast optimization, and motion artifacts. Described in the following sections are some techniques used to overcome these technical problems.
Optimizing SNR
The four major factors that significantly contribute to the SNR in MRI are field strength, voxel size, acquisition time, and coil selection (see Chapter 3 for a detailed discussion).
- 1.
Field strength: The higher the field strength, the more surplus spins align with the main magnetic field, participate in image formation, and contribute to the SNR. 3-T magnets have an approximately twofold higher SNR compared to 1.5-T magnets. However, scanning at higher field strength imposes challenges such as altered T1 contrast due to longer T1 times, shorter T2* decay due to magnetic field inhomogeneities, increased chemical shift artifacts, and higher SAR.
- 2.
Voxel size: SNR is directly proportional to voxel volume (i.e., SNR decreases with voxel size). In children, anatomic structures are significantly smaller than in adults; the size of the brain of an average-weight term newborn is approximately 25% that of an average adult. Therefore scans with higher spatial resolution (i.e., smaller voxel size) are needed to depict anatomic features in the same detail. Increasing the resolution decreases the voxel volume and hence the SNR. For example, if the resolution is doubled in all three spatial dimensions, an eightfold reduction in the voxel volume and SNR results.
- 3.
Acquisition time: SNR is proportional to the square root of image acquisition time for a given pulse sequence, coil selection, and voxel size; if the voxel volume is halved, the acquisition time must be quadrupled to maintain the same SNR. Higher SNR can be achieved by increasing the number of averages, decreasing receiver bandwidth, reducing the parallel imaging factor, and oversampling the k-space; all these measures lead to longer acquisition times. In pediatric imaging, longer acquisition times may, however, not always be feasible, owing to limited patient cooperation or time constraints due to sedation.
- 4.
RF coils : Appropriate coil selection is another means to optimize the SNR in pediatric MRI. There are two important factors for boosting the SNR: first, selecting a coil that is appropriate in size for the anatomy being scanned ( Fig. 6-8 ), and second, using multichannel coils with smaller elements. The size of the RF coil should be approximately 1.5 times larger than the area of interest. At many sites, infants are imaged using adult head or knee coils because of the lack of dedicated neonatal head or body coils. Often the size of adult volume coils is too big for infants and young children, which results in a suboptimal filling factor (i.e., the ratio of tissue volume to the volume of the coil). A coil that tightly fits the anatomy of interest has a higher filling factor and produces better SNR than one with a lower filling factor when scanning under identical conditions ( Fig. 6-9 ). State-of-the-art array coil systems consist of up to 128 coils with separate receiver chains. Higher SNR can be achieved with such arrays because each coil element detects magnetization and noise from its immediate vicinity only (i.e., coil sensitivity area; see Chapter 3 ). Coils that are not contributing signal to the imaging volume should be turned off to reduce image noise. Overall, phased array coils allow acquisition of fewer signal averages, which shortens the scan time while maintaining high SNR and resolution.
FIG 6-8
Selected commercially available infant array coils. A, A neonate head birdcage coil designed to cover 95th percentile of newborns weighing up to 4.5 kg and 55 cm whole-body length. The coil is designed to accommodate intubated patients as well (a notch is present on the anterior section of head coil for tube clearance). B, A 16-channel integrated infant head/spine array (IHSA) coil suited for high-resolution head/spine imaging in infants. C, A 20-channel infant cocoon suited for brain, spine, cardiac, and torso imaging of 95th percentile of 6-month-old infants.
(Courtesy SREE Medical Systems, Cleveland, OH [ www.sreemedical.com ].)
FIG 6-9
Size-optimized 32-channel receiver array coils for pediatric brain imaging. A, Posterior coil segments of five constructed pediatric array coils. The coil formers are based on the 95th percentile MRI contours of corresponding-aged children and have been dilated to accommodate foam padding. The coil formers were 3D printed and enclosed in a plastic box. Mounted mirrors are used to project visual stimulus for research studies. B, SNR comparisons between sagittal images obtained from the sized-matched head phantoms using the pediatric brain array coils (first row), the 32-channel adult brain array coil (second row), and a CP birdcage coil (third row). Images show that the highest SNR gain occurs closest to the surface of the constructed array. In the “brain” center of the phantoms the SNR is only slightly improved. The superimposed ROI on the birdcage coil SNR maps correspond to the regions used in the average brain SNR measurement. The 7-year-old phantom was too big to fit into the pediatric birdcage coil.
(From Keil B, et al: Size-optimized 32-channel brain arrays for 3T pediatric imaging. Magn Reson Med 66:1777–1787, 2011, Figs. 2 and 5. ©Wiley-Liss, Inc. Reprinted with permission of publisher.)
Optimization of Contrast
Optimal image contrast can partly compensate for low SNR in pediatric MRI by using a combination of different image weightings and by using contrast agents. The spectrum of disease, the T1s, and the T2s for tissues and lesions are very different in children compared to adults, and thus parameters used for T1 and T2 weighting in adult imaging cannot be automatically replicated for pediatric imaging. In addition, changing one sequence parameter may necessitate changes in multiple other parameters. Hence familiarity with the basic sequence properties and their tradeoffs is necessary for the technologist performing MRI in the pediatric population.
Changing Contrast Due to Maturation.
In children the anatomy is not only smaller than in adults, but there are normal changes in the appearance of many structures as children grow, develop, and mature. For instance, the water content in the body is 75% at birth and decreases to only 55% to 65% for an average adult. The developmental changes in children are more apparent in the brain, bone, and cartilage. In the brain, sulcation and myelination of white matter tracts occur rapidly during the first 2 to 3 years of life ( Fig. 6-10 ). In the skeleton, with maturation there is conversion of the epiphyseal and apophyseal cartilage to bone, as well as conversion of hematopoietic marrow to fatty marrow. Knowledge of these developmental changes is imperative for accurate interpretation of studies. These developmental changes also cause different tissue contrast in children compared to adults when the same imaging parameters (TR, echo time [TE]) are used, and hence create challenges in optimization of the parameters for pediatric imaging. In general, age-adapted protocols should be used.
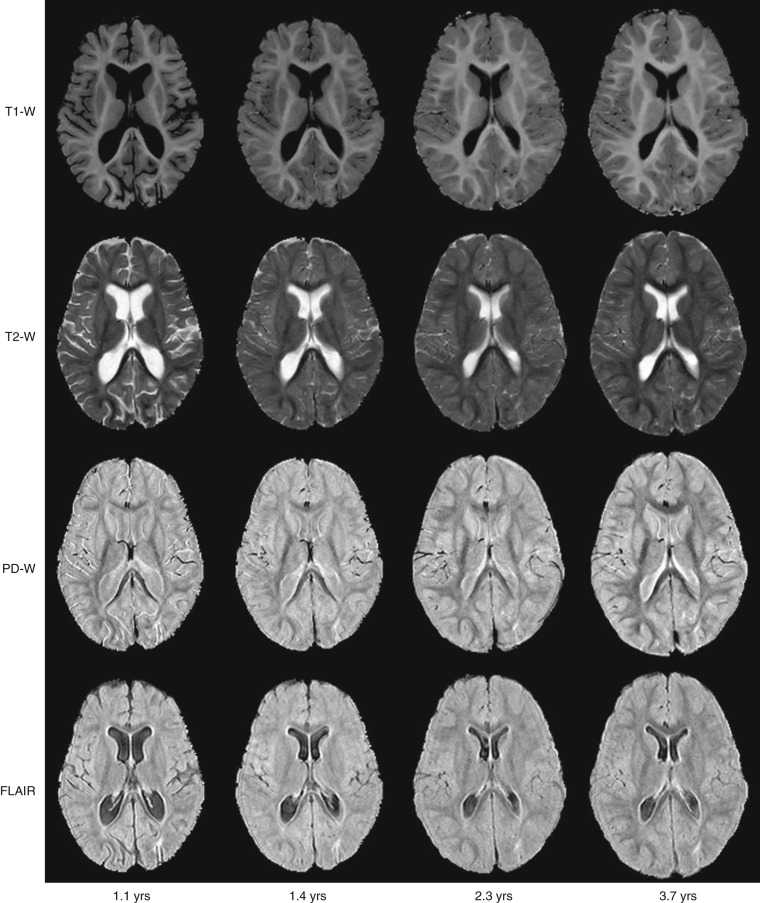
Native Contrast.
One of the main advantages of MRI over other imaging modalities is the ability to produce different tissue contrasts (principally T1-, T2-, proton density [PD], and diffusion-weighted) by exploiting the inherent properties of different tissue types. Optimal weighting in MRI is based on the combination of sequence parameters (TR, TE, inversion time [TI], flip angle, b value) chosen to provide good contrast that can differentiate a lesion from its surrounding tissue. Selection of the parameters would be easy if the exact values of T1 (for TR and flip angle), T2 (for TE), and apparent diffusion coefficient (for b value) for the tissue of interest are known. Unfortunately these values change because of the pathology, interindividual differences, and even intraindividual change over time (e.g., change in tumor characteristics over the course of treatment). One solution is to use a wider spectrum of weightings to obtain weakly and heavily weighted images, and another solution is to fuse images with different weightings (e.g., a combination of T1, T2, and diffusion) using postprocessing software.
Intravenous Contrast Agents.
Gadolinium (Gd)-based contrast agents greatly improve diagnostic image quality and provide clinically relevant information in addition to native MRI, as for adults. Table 6-1 gives an overview of the main applications for which contrast enhancement in pediatric MRI is indicated. Dynamic contrast-enhanced (DCE)-MRI assists in measuring important physiologic parameters such as perfusion, capillary permeability, or glomerular filtration rate (GFR) (see Chapter 5 for further reading). DCE-MRI is more challenging in children because the smaller vessels limit both the pressure with which a contrast bolus may be administered (i.e., slower injection rates, smaller-gauge catheters) and the volume of contrast used. Furthermore, the faster circulatory dynamics and smaller anatomy typically found in young children may pose an additional challenge in acquiring high spatiotemporal images for first-pass studies. However, recent studies demonstrated the use of combined parallel imaging and compressed sensing techniques to resolve the challenges of high spatiotemporal resolution and respiratory motion in pediatric body DCE-MRI.
