Fusion techniques are considered the gold standard for treatment of lumbar spinal instability, although there are many shortcomings and disadvantages. In the past two decades the concept of dynamic stabilization has been introduced in clinical practice. A more thorough knowledge of the spinal biomechanics has led to the ability to modify the loads within the spinal unit. Many different devices based on this concept were designed. Almost all these devices are percutaneous or minimally invasive. Thus, not only surgeons but also interventional radiologists can play a major role in the treatment of the degenerated spine.
The skull essentially protects the brain. The spine, made of a series of joints, has motion as its primary function, at the same time protecting the spinal cord. The basic spinal functional unit (SU) is the smallest physiologic motion unit of the spine and therefore is termed, a motion segment . It consists of 2 adjacent vertebrae, the disc, and all the connecting ligaments. Individual motion segments contribute to the total motion of the spine. Abnormal motion in an SU is defined as vertebral or spinal instability. Myriad biomechanical, clinical, and radiologic definitions of spinal instability are available. The definition by the American Academy of Orthopaedic Surgeons states, “Segmental instability is an abnormal response to applied loads, characterized by motion in motion segments beyond normal constraints.” Motion in degenerated joints (ie, beyond the normal limits of the joint itself) generates pain. Eliminating motion in an abnormal SU seems to eliminate pain. Most orthopedic surgery for the degenerated lumbar spine, entailing various fusion (ie, locking of 2 or more vertebrae as a single unit) techniques, with or without instrumentation, is based on this principle. Disadvantages and problems of traditional fusion methods are loss of mobility and curvature, altered sagittal balance, instrumentation failure, harvest site pain, infection, and transference of load (stress) to adjacent levels. Such surgery, moreover, is not easily reversible. The main concern of fusion surgery is the impact on the adjacent levels, in particular facet joints and discs, structures that are then overloaded. After fusion surgery, these may consequently deteriorate ( Fig. 1 ). Hilibrand and colleagues have estimated that after cervical fusion there is 25% symptomatic adjacent level disease at 10 years; Ghiselli and colleagues estimate 36% at 10 years after lumbar fusion.
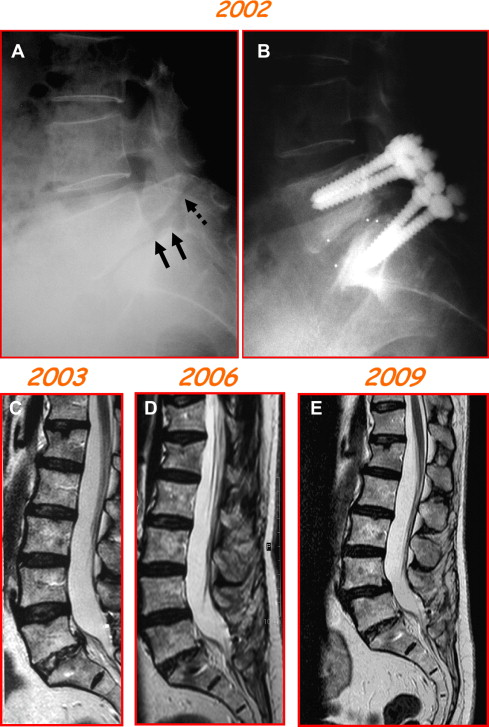
All of these issues have led to attempts to develop new motion preservation technologies for surgical treatment of spinal instability, commonly referred to as dynamic stabilization. Dynamic stabilization has been defined as “a system that would alter favorably the movement and load transmission of a spinal motion segment, without the intention of fusion of the segment.” Dynamic stabilization tries to introduce a more gradual, intermediate therapeutic step between abnormal movement of the SUs (instability) and total absence of movement (fusion). The most significant advances in this field were made in the past 10 to 15 years; during the same period a gradual shift toward a minimally invasive approach of spinal surgery was technically developed and accepted. During this period, attention of biomechanics experts and spine surgeons was mainly focused on the posterior structures of the spine, facets, and spinous processes, for 2 reasons: these structures are easily accessible by a minimally invasive approach and an action on them determined by different devices can largely modify the functional behavior of the SU. The combination of 2 concepts, preservation of motion and minimal surgical invasiveness, seems to be opening a new era in surgery for the degenerated spine. The radiologic community, which has always been in the forefront of developing and performing minimally invasive interventions for the degenerated spine (percutaneous discectomies, vertebroplasty, and other procedures) is well situated to participate in applying these innovations.
Basic biomechanics
The SU is extremely complex in its components and movements. Thus, there can be many different sources of pain in pathologic situations. Unfortunately, the biomechanics of SUs are neither fully understood nor simple to explain. This article summarizes the main concepts on which the designs of the different devices are based, simplifying the 3-D anatomy and physiology of the SU to a 2-D model ( Fig. 2 ) (ie, essentially eliminating the torque and lateral bending movements, which are not, or almost not, affected by the dynamic devices).
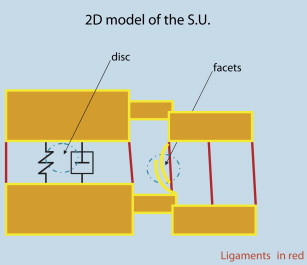
In flexion and extension, muscles apply a bending moment to the SU. A bending moment ( M ) corresponds to 2 vector forces applied in opposite directions with a distance between them different from 0 and is measured as a force ( F ) multiplied by a distance ( d ): M = Fd ( Fig. 3 ). During flexion of the lumbar spine, muscles apply a bending moment to the SUs ( Fig. 4 ).
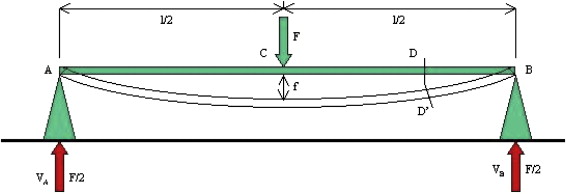
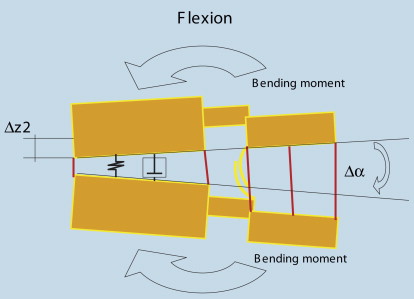
The total motion obtained (modification of posture from neutral to flexion) is the sum of the modifications obtained at the level of every single SU (ie, a decrease of the anterior disc height [Δz2] and a widening [defined by the angle between the spinous processes: αΔ] of the posterior structures, which are stretched and opened). The supraspinous ligament is the structure limiting flexion more effectively. The opposite happens in extension, with an increase in the anterior disc height and closing of the interspinous space, with the angle becoming negative ( Fig. 5 ). Two concepts to be acquainted with are that of neutral zone (NZ) and that of instantaneous center of rotation (ICR). The NZ ( Fig. 6 ) is the position of the SU in which a small bending moment can obtain a large movement (ie, a large change of the angles between the 2 vertebrae). In a normal SU, the center of the NZ corresponds to the middle position between flexion and extension; a small moment is required to start flexion (or extension), but with progressive increase of the movement it becomes harder and harder to obtain new flexion (or extension). The NZ is a measurement of the laxity of the SU, and it widens in the presence of instability. Pathologic widening of the NZ allows exaggerated movements, which in turn require a large amount of energy for return to the neutral state. Dynamic devices aim to reduce the NZ or to reposition it in the proper (not painful) place.
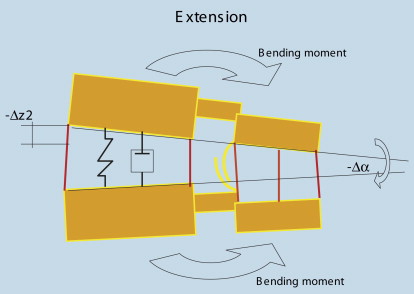
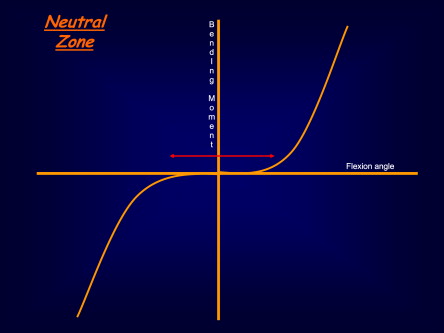
The ICR corresponds to the point at which, if a load is applied, no bending occurs. It is defined as instantaneous, because it can change at every instant, during different types of movements. As an example, think of a bike’s wheel. When the wheel turns around the central pivot not touching the ground, the ICR corresponds to the not moving center of the hub, but in a running bike the only not moving part is the one touching the ground, and it changes at every instant (imagine the wheel turning as a whole around the fixed point in contact with the ground). It is difficult to predict the ICR in structures as complex as the SU. It changes with different movements, and these changes become more unpredictable in the presence of instability. More often, in a healthy SU in the standing, inactive position, the ICR is located posterior to the center of the disc, just above the inferior end plate (approximately corresponding to the center of gravity). It moves in flexion-extension, and the variability is considerable. There are no simple rules to predict the effect of stabilization devices on the ICR, but one is notable: the ICR moves toward an increase of stiffness. What happens when an interspinous spacer is deployed? Things are not modified in flexion (if the supraspinous process is surgically preserved); in extension, the movement is not modified until the spacer goes under compression.
At that moment ( Fig. 7 ), if the spacer is rigid and provided that the bone does not fail (break) (see Fig. 7 for the weakest points of the posterior arch, which are possible sites of failure), an additional increase in the anterior height of the disc is obtained (−Δz3), with stretching of the anterior annulus. To allow and compensate for this, the facets move opposite to the normal direction, opening instead of closing. In other words, the movement, which is no longer obtainable at the expense of the interspinous space (decrease of the angle in extension [see Fig. 5 ]) is now obtained at the level of different, elastic structures. An immediate consequence is that back pain induced in extension by pressure originating in the facets or posterior annulus of the lumbar spine may be relieved by unloading of facets or posterior annulus generated by the interspinous decompression. In a similar way, is it possible to unload the whole disc, when it is surmised as the source of pain? The disc is extremely stiff, and only minimal increase-decrease in disc height con be obtained under axial compression-distraction (Δz1 in Fig. 8 ). The disc can be unloaded only if the load is transferred to a device/instrumentation, but implants are not stiff enough to accomplish that task. For this reason, stand-alone instrumented fusions fail in the long term if bony fusion does not take place. This is a general rule of fusion surgery: a fusion cannot be expected to be obtained simply by hardware, because the action of the hardware is temporary. Its action is to allow for actual bone fusion to take place. Otherwise, instrumentation (even metallic ones) and contact surfaces with bone fail in the mid or long term. The disc, however, is compliant in flexion-extension, because of posterior and anterior shifting of the nucleus pulposus in flexion-extension. Thus, if posterior stabilization devices cannot have a primary role in unloading the disc, they can have a profound effect on the loads of specific regions of the disc. Fig. 9 depicts the effects of a tension band (tying the upper and lower spinous processes to the interspinous device), which give an additional moment to resist bending. In a normal/uninstrumented condition, the ICR in flexion moves anteriorly, whereas the action of the tension bend keeps it more posterior, limiting its shifting. The consequence is a reduction of compression forces on the anterior annulus and reduction of tension on posterior annulus and facets. Alternatively ( Fig. 10 ), the rigid interspinous spacer in extension moves the ICR posteriorly behind the facets (ie, toward the increase of stiffness determined by the device), modifying the loads on the different parts of the SU: the load on facets and posterior annulus is reduced instead of increased (tension instead of usual compression). Thus, with wise use of the correct device, the loads can be moved away from painful areas, giving relief to patients and at the same time preserving motion in the SUs.
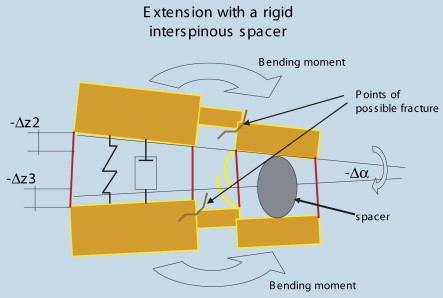
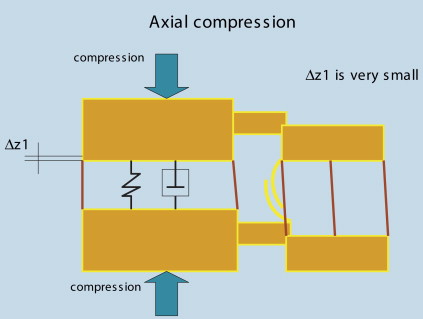
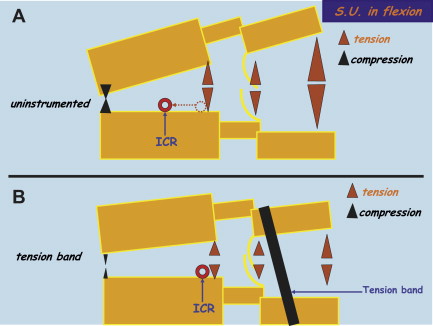
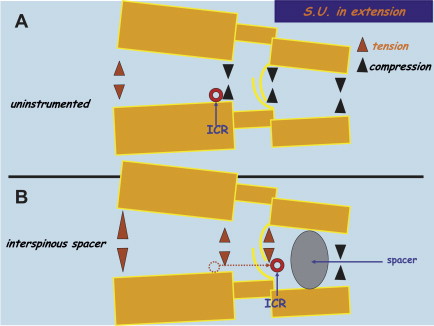
Design rationale of different devices and general surgical principles
Regarding dynamic stabilization devices, the only surgical approaches to date that can be considered minimally invasive, and widely available to clinical practice, are the posterior ones, for anatomic reasons. Anterior approaches for nonfusion procedures, such as the ones for total disc replacements (prostheses), still require open procedures. Nucleus replacements (replacing the nucleus only, with the advantage of cartilage and annulus preservation) are under ongoing development and evaluation. Many different materials are proposed, the most promising among them represented by injectable polymers. No single system is widely available in clinical practice or truly minimally invasive.
Posterior stabilization devices fall into 2 main categories of design: interspinous spacers (with or without tension bands) and pedicle screw-based systems. The bands are passed around the upper and lower spinous processes and then tied to the interspinous component, with the double purpose of securing the device and (in some devices) limiting flexion/rotation. Rigid, nondeformable interspinous spacers have a constant effect on the distraction of the spinous processes, whereas low-rigidity, deformable spacers act more as shock absorbers, with a consequent, more physiologic action on range of motion of the SU together with an increase of bone compliance.
The interspinous process decompression system X-STOP ( Fig. 11 ) (St. Francis Medical Technologies, Alameda, CA, USA, now owned by Medtronic) was proposed by Zucherman and colleagues in the late 1990s, for treatment of symptoms of neurogenic intermittent claudication (INC) due to segmental spinal stenosis. The X-STOP consists of an oval spacer that is positioned between the 2 symptomatic spinous processes. The lateral wing is then attached to prevent the implant from migrating anteriorly or laterally out of position. Anterior migration and posterior migration are also limited, respectively, by the lamina and the supraspinous ligament (the latter not being violated). The central pivot was rigid, made of titanium, in the first version. Now it is semirigid thanks to a layer of polyetheretherketone (PEEK) (discussed later) external to the metal. It is deployed through a small posterior surgical approach. It is intended to prevent extension of the stenotic levels, yet allowing flexion, axial rotation, and lateral bending. The intervention can be performed under local anesthesia and mild sedation, without removing any bone or soft tissues, with preservation of the supraspinous ligament. Leaving in place and intact the supraspinous ligament has the double of effect not only preventing posterior migration of the device but also not modifying the behavior of the SU in flexion. The procedure is typically done with a 24-hour hospitalization.
