Fig. 9.1
(a) Coronal SSFSE T2w image of a fetal chest at 24 weeks gestation with intermediate signal lung parenchyma. Note the fluid-filled trachea and bronchi (b). Coronal SSFSE T2w image of a fetal chest at 35 weeks gestation. Note the increase in signal of the parenchyma as compared to 24 weeks GA
The airways, including the trachea, carina, and bronchi, are typically filled with fluid and thus appear bright on T2w (Fig. 9.1a). When needed, repeating sequences with thinner slices may be beneficial for further evaluation.
The thymus is a homogeneous anterior mediastinal structure which should not exert any mass effect on surrounding structures. It is homogeneously intermediate in signal best seen in the third trimester as it increases in size.
The heart is low signal on T2w SSFSE due to flowing blood. SSFP sequences are more useful in delineating the myocardium, septum, and valves (Fig. 9.2). Dynamic SSFP sequences can demonstrate cardiac motion.
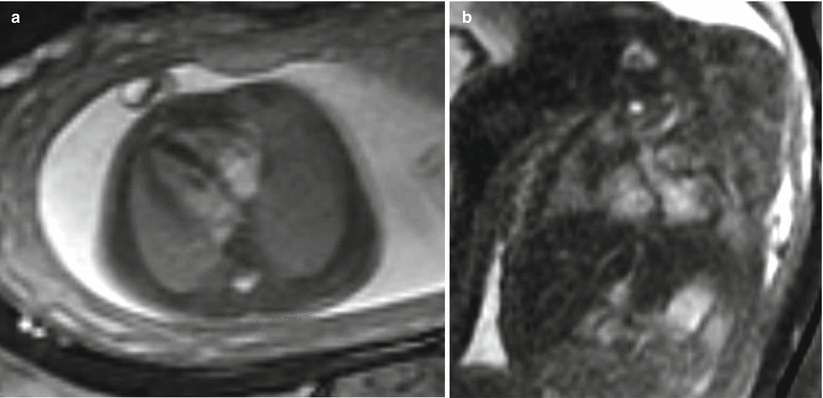
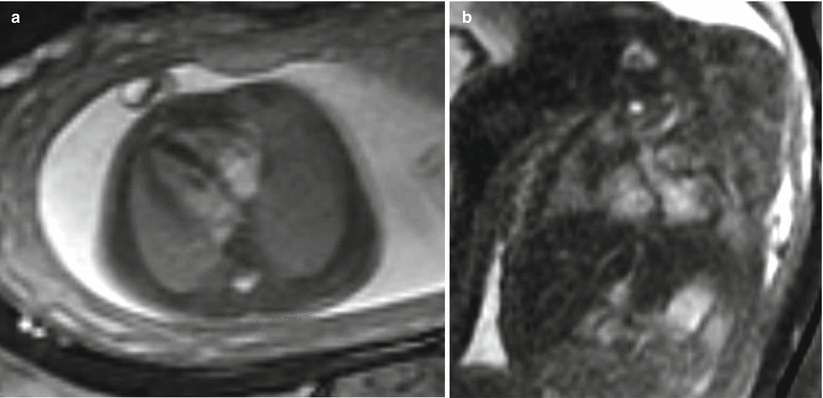
Fig. 9.2
(a) Axial SSFP image at 27 weeks gestation demonstrates normal axis of the four-chamber view of the heart. The blood is bright on this sequence allowing visualization of the ventricular septum. (b) Coronal SSFP image demonstrates the left ventricular outflow track
The diaphragms are dome-shaped bands between the lungs and the abdomen. They have low signal on T2w, slightly lower than the adjacent liver. Breathing may be noted on dynamic SSFP sequences.
9.3 Lung Development
Different but overlapping stages of maturation are seen in the lungs throughout gestation. Stages include embryonic (26 days to 6 weeks), pseudoglandular (6–16 weeks), canalicular (16–28 weeks), saccular (28–36 weeks), and alveolar (36 weeks to childhood). There is an overlap between these stages due to differing speeds of maturation between central and peripheral lung regions and cephalad and caudal segments [8, 9].
During the embryonic stage, the laryngotracheal tube originates as a ventral diverticulum from the primitive pharynx. With time, it elongates to form the larynx and trachea from its proximal end and the lung buds distally.
By days 26–28, the lung buds enlarge and divide. A right and left bud will continue to branch during the fifth week of gestation, forming three secondary branches on the right and two on the left. Further dichotomous branching continues to occur until the 20th week when the terminal bronchioles are formed. Several more divisions occur to form the respiratory bronchioles. Major structures are formed by 28th week but the lungs continue to mature until early childhood.
The diaphragm has four different embryonic origins: the septum transversum, the pleuroperitoneal membranes, the paraxial mesoderm of the body wall, and the esophageal mesenchyme.
9.4 Abnormalities of the Fetal Thorax
9.4.1 Congenital Diaphragmatic Hernia (CDH)
9.4.1.1 Definition
CDH is a congenital anomaly that affects the development of the diaphragm and allows for intra-abdominal organs to move into the thoracic cavity. It results in lung hypoplasia from lung compression and mechanical complications from mediastinal and cardiac deviation.
9.4.1.2 Classification
CDH can be classified based on location: posterolateral Bochdalek hernia (70–75 %), anterior Morgagni hernia (23–28 %), and central defects (2–7 %) [10]. Most CDHs occur on the left side (85–90 %), some on the right (10–15 %), and only rarely bilaterally (2 %) [11].
CDH can also be differentiated as isolated or complex. CDH is often isolated. However, in 23–43 % of cases, an associated anomaly can be present [12], most likely a cardiac defect (20 %) [11, 13, 14]. When multiple abnormalities are present, genetic anomalies should be considered with a higher mortality [14]. More than 50 genetic causes have been described and can vary from chromosomal abnormalities such as trisomy 18, microdeletions, or microduplications to syndromes with unknown genetic mutations (Fryns syndrome).
9.4.1.3 Imaging Findings
US findings seen in patients with CDH include presence of stomach bubble, gallbladder or bowel within the thoracic cavity, deviation of the heart and mediastinum, herniated liver, abnormal position of the umbilical and hepatic veins, pleural effusion, and polyhydramnios [11, 13, 15]. MRI is an adjuvant study helping to confirm the diagnosis, aiding in the search for additional abnormalities, and providing additional data such as lung volumes that may help predict outcome.
MRI better demonstrates the position of the liver and the amount of herniation due to superior differentiation of the hepatic lobe (bright on T1) from the compressed lung or a solid type of CPAM [16–18]. MRI can also help by providing better evaluation of the bowel with bright T1 signal of meconium and allowing visualization of the normal ipsilateral and contralateral lung [19] which is useful in the assessment of pulmonary hypoplasia [7] (Fig. 9.3). Suggestion of a hernia sac by a rounded appearance of the most superior aspect of the hernia has been associated with a better prognosis [7, 16] (Fig. 9.4).
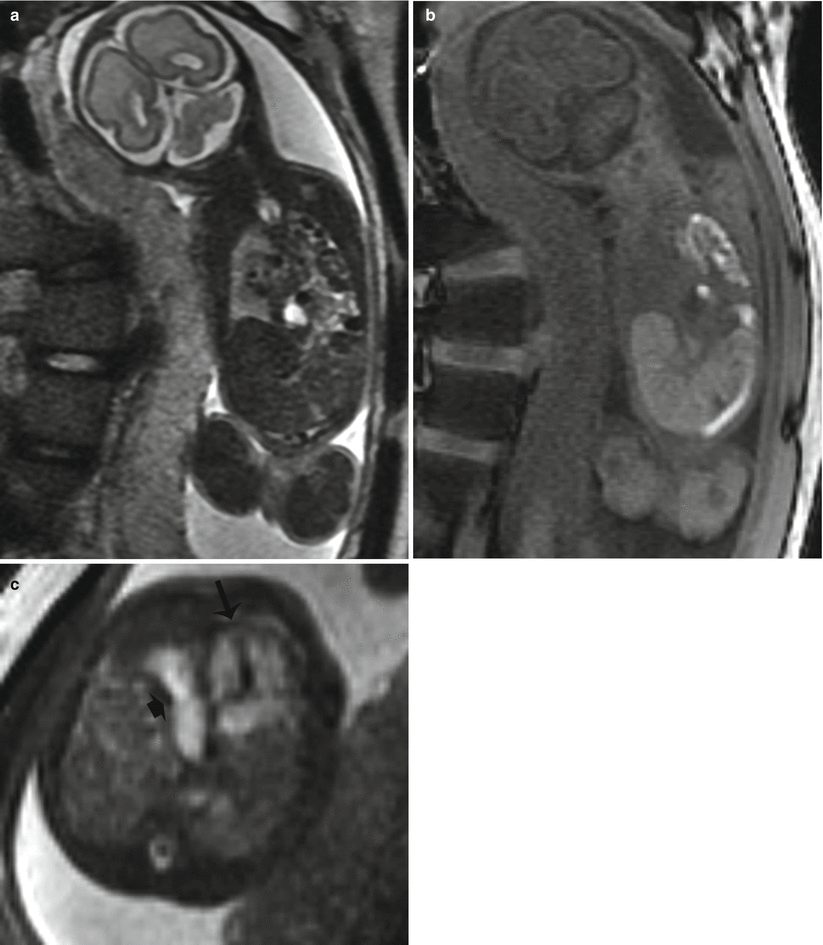
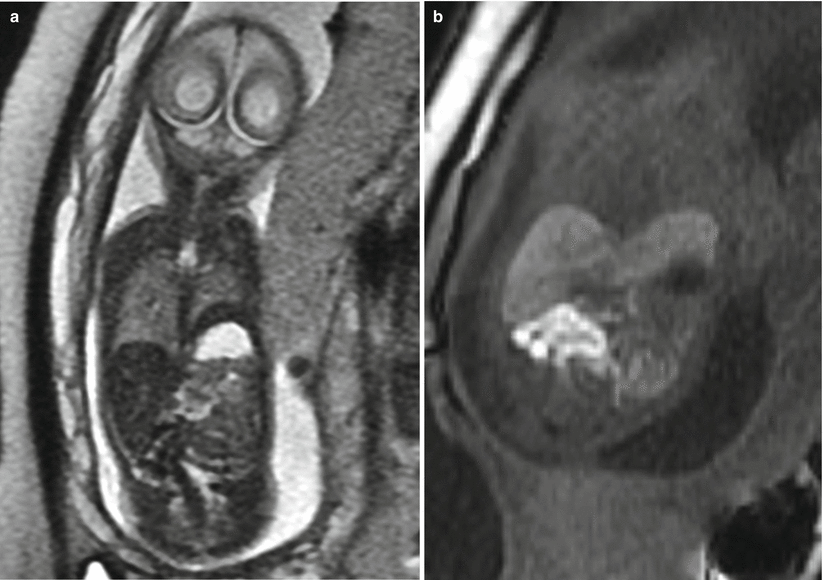
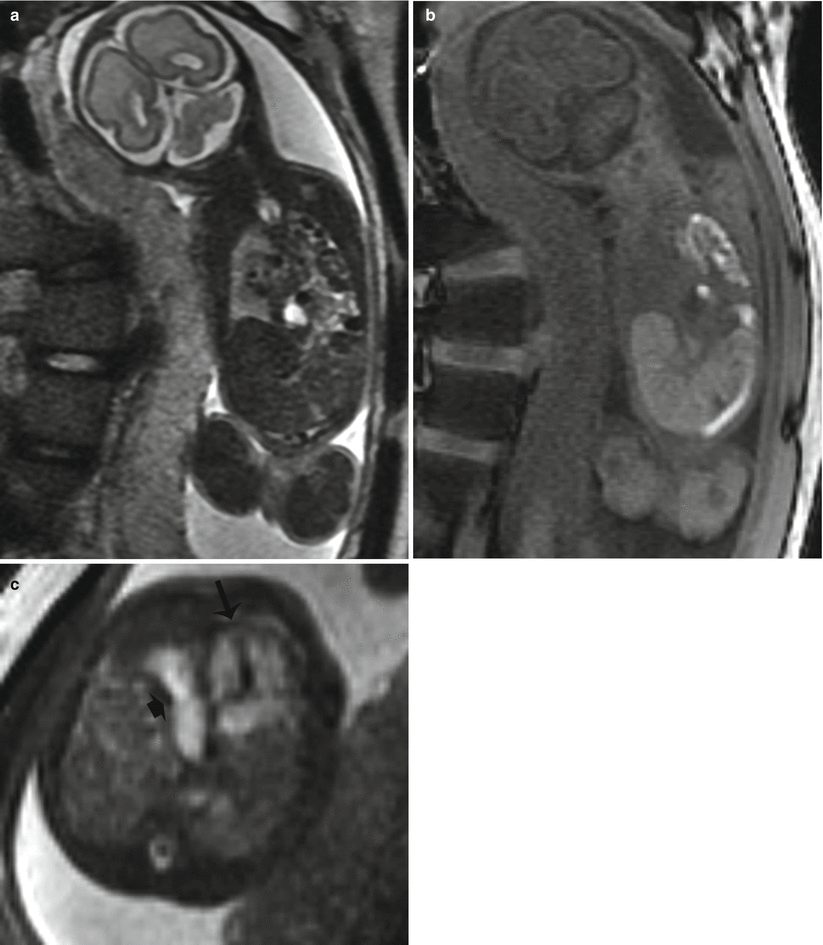
Fig. 9.3
Left CDH at 26 weeks gestation. (a) Coronal SSFSE image demonstrates bowel filling of the left hemithorax. Residual lung is noted on the right. (b) Coronal T1w image demonstrates meconium-filled bowel herniated into the left hemithorax. (c) Axial SSFP image of the chest demonstrate the heart (long arrow) to be shifted moderately to the right. The stomach (short arrow) is herniated into the chest with heterogenous collapsed bowel posterior to the stomach. A small amount of herniated left lobe of the liver is noted anterior to the stomach
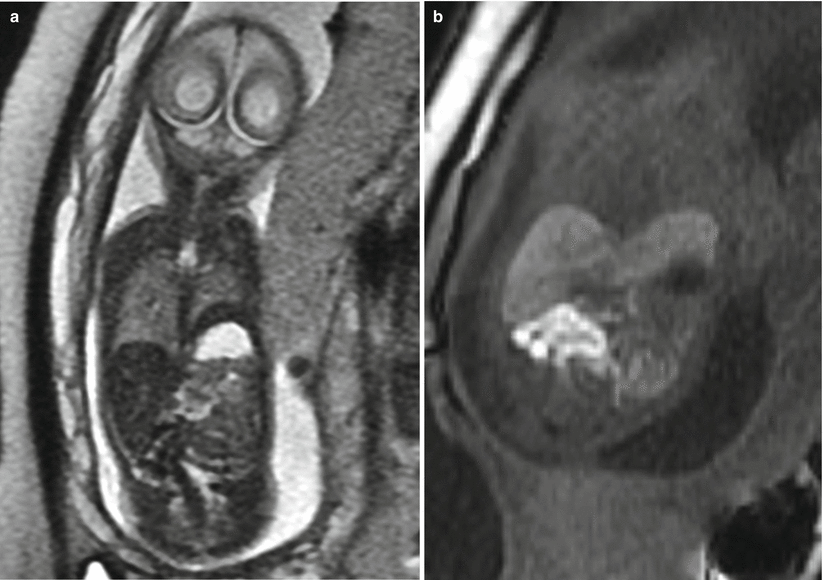
Fig. 9.4
Membrane-covered CDH at 22 weeks gestation. (a) Coronal SSFSE image demonstrates elevated left hemidiaphragm with residual left upper lung. (b) Coronal T1w image demonstrates herniation of the liver into the left hemithorax. The infant did well after delivery without requiring ECMO
Different volumetric measurements can be obtained with MRI and used as indicators of outcome. MR measurements allow assessment of both lungs and can be more reliably obtained than US [17]. MRI measurements include total lung volume, observed to expected total lung volume (o/e TLV), percent predicted lung volume (PPLV), fetal lung volume to fetal body volume ratio (FLV/FBV), percent liver herniation (%LH), and herniated liver to fetal thoracic volume ratio (LiTR).
MRI can measure the diameter of the aorta and pulmonary arteries used to obtain the modified McGoon index as a prognostic indicator of pulmonary hypertension [19].
Imaging with diffusion-weighted sequences to assess growth and maturity has been attempted though no correlation has been seen with lung volume or fetal survival [20].
9.4.1.4 Prognosis and Fetal Management
Prognosis varies depending on the side of the herniation, the position of the liver, associated abnormalities, and age at diagnosis [12].
The presence of herniated liver decreases survival from 74 to 45 % [21], and the presence of associated anomalies also decreases the survival [12]. The severity of lung hypoplasia and resulting lung hypertension are major determinants in the mortality and morbidity of the patients [22–24], and a larger herniation diagnosed earlier results in worse pulmonary hypoplasia with a resulting higher mortality.
Bilateral hernias are usually fatal. Familial and syndromic hernias usually have a worse prognosis compared to isolated hernias [25].
Different ultrasound and MRI measurements have been used to estimate the severity of lung hypoplasia and probable outcome of the fetus:
(a)
Lung to head circumference ratio (LHR), obtained by ultrasound, has been used as a predictor of outcome and as indicator of the need of more aggressive treatment such as ECMO. LHR less than 1 is seen in patients with poor outcome, with a survival rate of 45 % [15, 26]. LHR’s prognostic value is variable due to differences in methodology, compression of normal lung, and dependence on gestational age [25], resulting in a controversial association with mortality [22].
(b)
(c)
Total lung volumes have been used to quantify degree of lung hypoplasia. A better prognosis is expected in fetuses with a total lung volume of 25 cm3 or more, compared to fetuses with a lung volume of 18 cm3 or less.
(d)
o/e TLV has a good correlation with ultrasound obtained LHR and has better predictive value compared to total lung volumes but is operator dependent and affected by motion [30]. Mortality rate of 87 % was seen in fetuses with o/e TLV of less than 25 %, and it decreased to 31 % in those fetuses with an o/e TLV of 25–35 % and further decreased to 17 % in those with an o/e TLV of more than 35 % [25].
(e)
Percent predicted lung volume (PPLV) is obtained by dividing the volume of the CDH by the volume of the expected lung volume; the expected lung volume is obtained by measuring the total lung volume and subtracting the mediastinal volume [31]. If PPLV is greater than 15 %, a good outcome with 100 % survival can be expected; if PPLV is less than 15 %, bad outcome is likely, with only 40 % survival [31].
(f)
%LH is the ratio obtained between the herniated liver and the total volume of the liver. It shows an accuracy of 87 % in predicting mortality [32].
(g)
LiTR is the ratio of herniated liver volume to total thoracic volume without the spine and has 85 % accuracy in predicting mortality [33].
(h)
FLV/FBV ratio. This measurement is equivalent to the postmortem lung weight to body weight ratio used to evaluate pulmonary hypoplasia. A lower ratio is associated with increased mortality [34].
In a study by Ruano et al., the best combination of measurements to assess mortality is o/e TLV and %LH [24].
Ultrasound methods exist to predict pulmonary hypertension. By MRI, the modified McGoon index can be obtained by adding the right pulmonary artery diameter plus left pulmonary artery diameter and dividing the sum by the diameter of the descending aorta measured at the level of the diaphragm [19].
Complete anatomical examination should be performed to assess for presence of associated anomalies (25–75 %) [35]. Echocardiography is indicated as congenital cardiac defects occur in 10–35 % of cases and decrease survival from 73 to 67 % if it is a minor defect or 36 % if major complex cardiac defect [25].
A prenatal karyotype with microarray is indicated to assess for chromosomal anomalies, present in 10–20 % of cases [14, 35].
Follow-up ultrasound examinations should be performed to assess fetal well-being, lung volume, and changes in mediastinal shift that could result in hemodynamic changes [36]. Third trimester follow-up MRI can be performed to assess lung volume near time of maximal intrauterine growth and plan delivery; this late MRI can also be used for postnatal surgical planning decreasing the need for postnatal cross-sectional imaging [19].
Different fetal surgical techniques have been attempted, such as in utero repair and fetal tracheal occlusion via open surgery or endoscopy, with clip or balloon occlusion. EXIT to ECMO is also offered in some centers but has not demonstrated significant survival benefits [21]. New nonsurgical prenatal options are being evaluated, including pharmacologic treatments and stem-cell-based strategies, and could be promising in treating pulmonary hypertension and hypoplasia [23].
9.4.2 Congenital Pulmonary Airway Malformation (CPAM)
9.4.2.1 Definition
CPAM is a congenital anomaly of the lung resulting in a cystic, hamartomatous mass of lung tissue that communicates with the bronchial tree through an abnormal communication and has normal pulmonary blood supply and venous drainage into the normal pulmonary veins [37].
9.4.2.2 Classification
CPAM has been classically classified based on pathology by Stocker et al. into type I (large cysts, more than 2 cm), type II (multiple small cysts), and type III (microcystic). Adler et al. described a simplified classification based on gross appearance, and its use is recommended in fetal medicine:
(a)
Macrocystic: One or more cysts measuring more than 5 mm
(b)
Microcystic: Multiple cysts smaller than 5 mm
It usually affects one lung (95 %) but can be seen bilaterally, and both lungs can be affected. It is usually unilobar (85–95 %) and usually occurs in the lower lobes [38].
9.4.2.3 Imaging Findings
MRI findings are characteristic of fluid-filled structures, demonstrating bright T2 signal. A solid appearing lesion or a multicystic mass lesion with small or large cysts surrounded by abnormal hyperintense parenchyma is appreciated in the thoracic cavity, depending on the type of CPAM (Fig. 9.5). The lesion exerts mass effect on the heart and other mediastinal structures causing heart rotation and shift to the contralateral side with different degrees of compression of venous structures and results in impaired venous return (Fig. 9.6). As a complication from mass effect and compression, pleural effusion, pericardial effusion, skin thickening, and/or ascites can develop (Fig. 9.7). Dilatation of the proximal esophagus can also be seen due to esophageal compression. As the fetus grows, the lesion becomes less conspicuous by ultrasound and can be better seen by MRI [3].
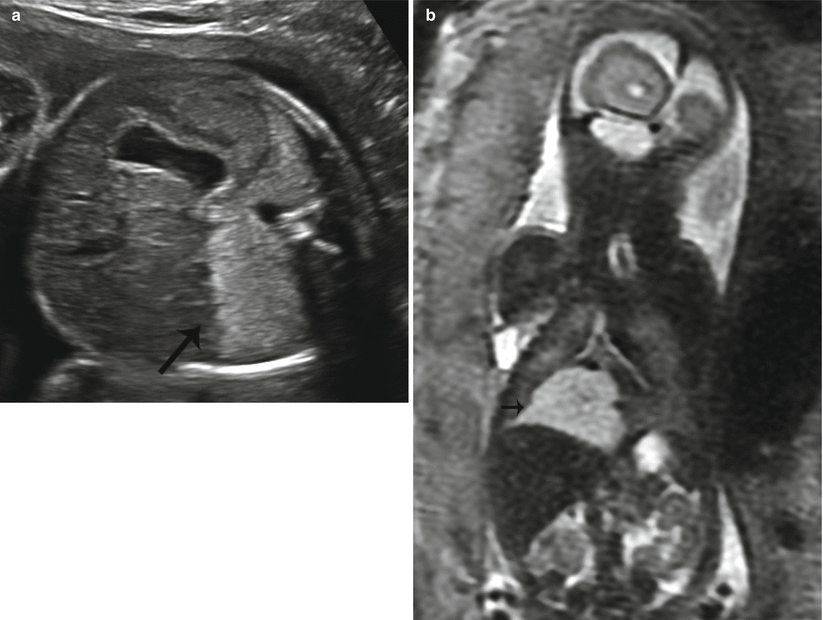
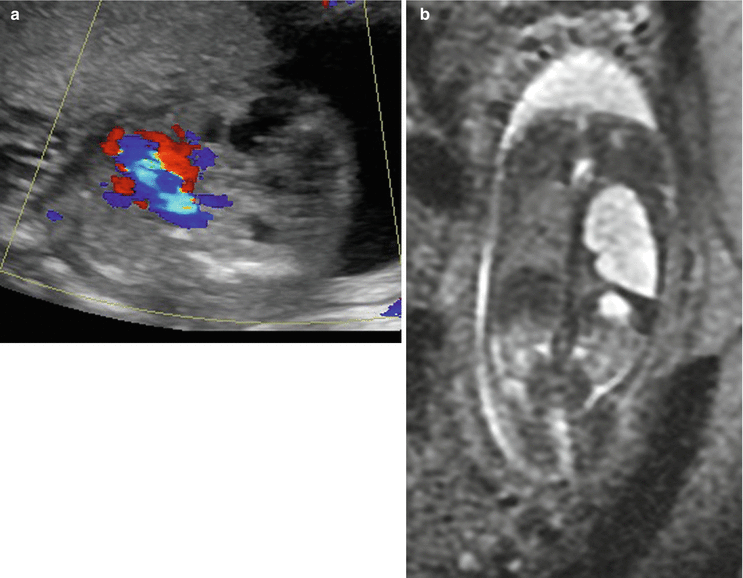
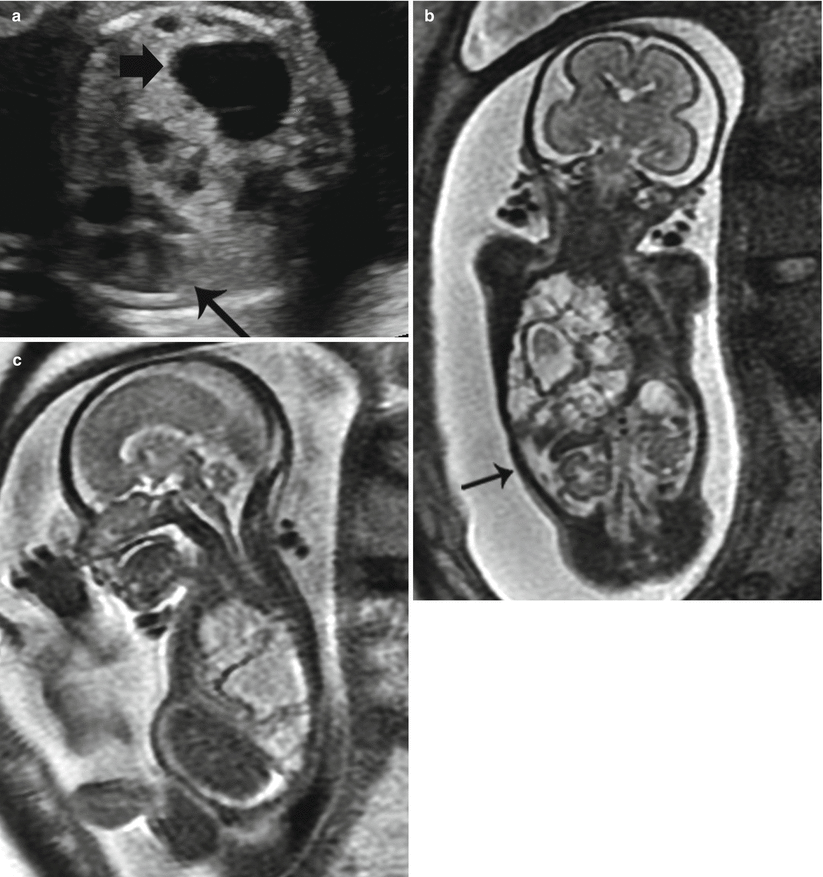
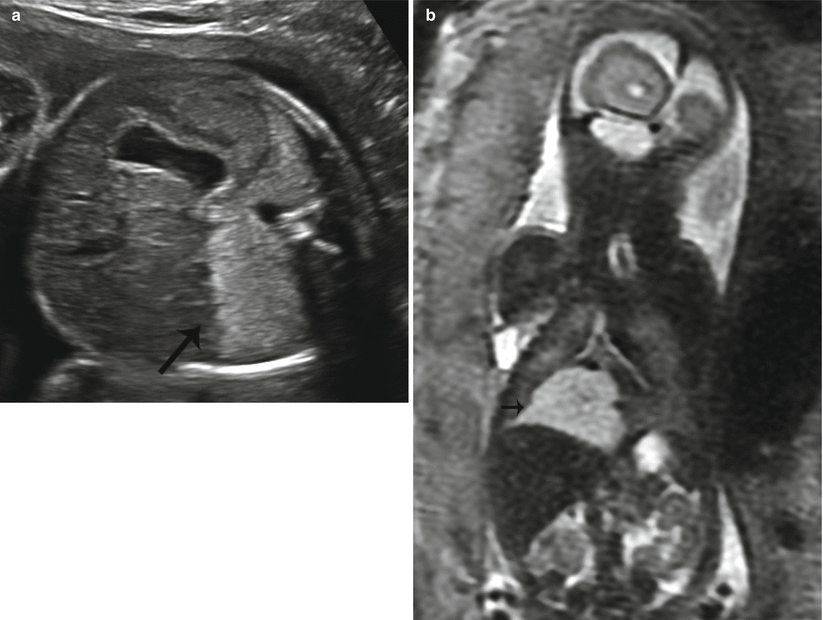
Fig. 9.5
Type III CPAM at 25 weeks gestation. (a) Axial US demonstrates an echogenic homogeneous mass in the right lower lobe (arrow). (b) Coronal SSFSE T2w image of the chest demonstrates a homogeneously high-signal mass in the right lower lobe (arrow). There was minimal mediastinal shift with a CVR of .4
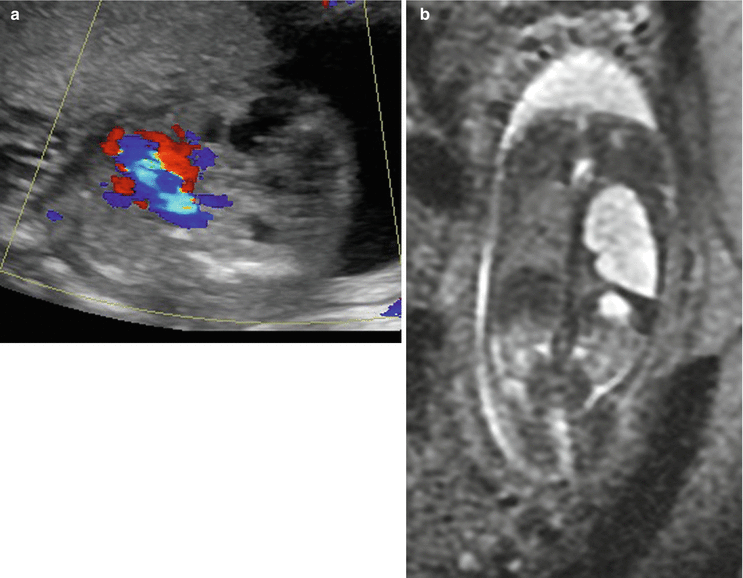
Fig. 9.6
Type 1 CPAM at 21 weeks gestation. (a) Axial US demonstrates macrocysts in the left lung deviating the heart (color) to the right. (b) Coronal SSFSE T2w image demonstrates that the cystic mass is in the left upper lobe
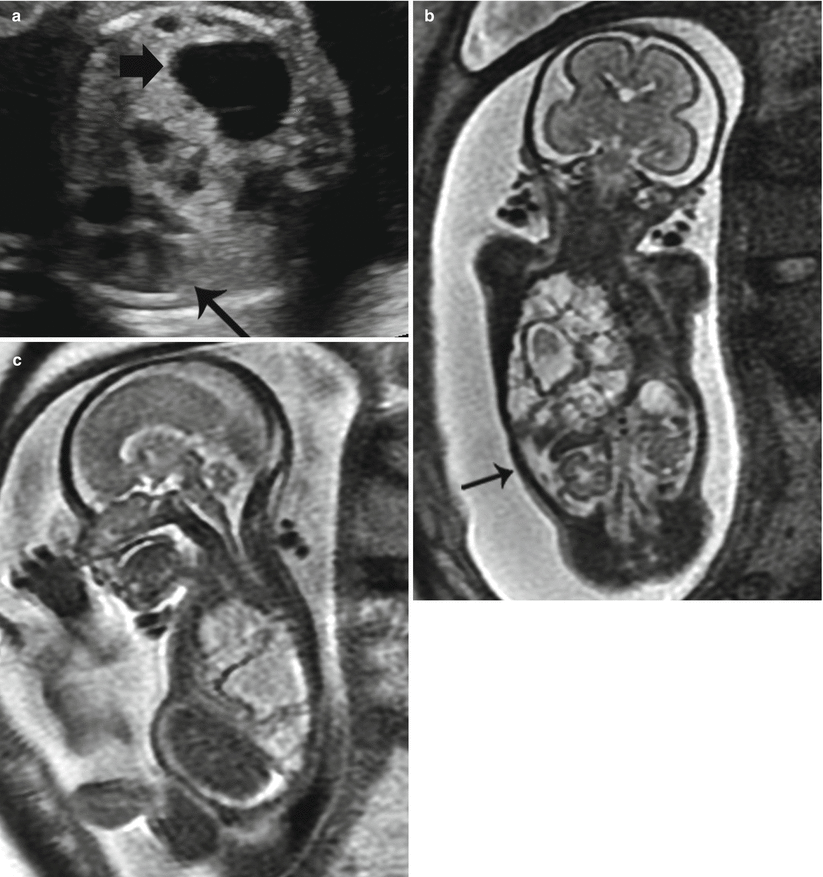
Fig. 9.7
Type 1 CPAM at 23 weeks gestation with ascites. (a) Axial US image demonstrates a right lung mass with scattered macrocysts (thick arrow) and surrounding echogenic tissue in the right lung deviating the heart (thin arrow) to the left. (b) Coronal and (c) Sagittal SSFSE MR images of the chest demonstrate macrocysts deviating the diaphragm inferiorly. Ascites is present (arrow). CVR measured was 2.3. The fetus survived following intrauterine drainage of several cysts
MRI allows evaluation of the residual normal lung and can sometimes be beneficial differentiating CPAM from other lung anomalies and identifying associated congenital anomalies [4, 39–41]. It can also help in differentiating between complete resolution and the presence of residual lesions, important for postnatal follow-up planning [7].
9.4.2.4 Prognosis and Fetal Management
Prognostic indicators include the size of the lesion, amount of mediastinal shift, and presence of polyhydramnios and/ or hydrops. Macrocystic lesions usually have a favorable prognosis, since most cases have a slow growth. When large, either macro- or microcystic, lesions can cause mediastinal shift and result in hemodynamical changes including polyhydramnios and nonimmune hydrops, which may require in utero intervention.
The CPAM volume ratio (CVR) [42], a measurement described by ultrasound, should be measured to assess prognosis and risk of hydrops. It is obtained by calculating the volume of the lung mass (height × anteroposterior diameter × transverse diameter × 0.52) and dividing it by the head circumference. A CVR <1.6 indicates a 14 % risk of developing hydrops for macrocystic lesions and 3 % for microcystic lesions. If CVR is >1.6, the risk increased to 75 % [42] (Fig. 9.8).
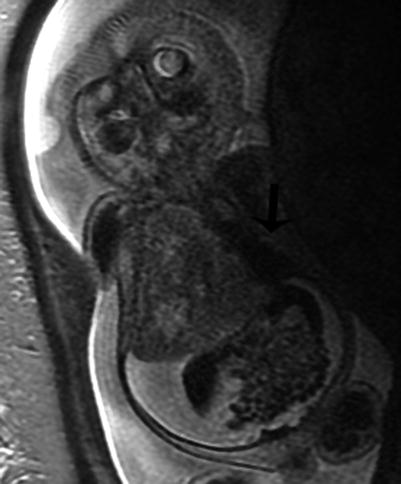
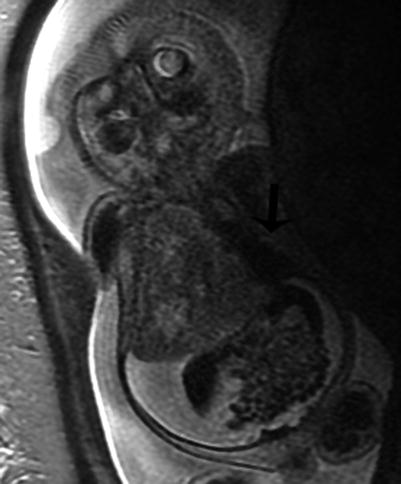
Fig. 9.8
Type III CPAM with hydrops at 28 weeks gestation. Coronal SSFSE MR image demonstrates a large heterogeneous mass in the right thorax deviating the heart to the left (arrow). CVR was 5. There is diffuse skin edema and ascites. The fetus died despite steroid therapy
CPAM typically demonstrates an increase in size between 20 and 26 weeks of gestation and then plateaus [43]. It can later decrease in size [43] or seem to disappear due to similar signal intensity compared to normal lung parenchyma [44]. A residual mass can be seen in postnatal images in 40 % of cases when resolution by prenatal images was suspected.
Differential diagnosis includes BPS, CLO, bronchogenic cyst, CDH, laryngeal or tracheal occlusion, neurenteric/enteric cysts, and mediastinal teratomas.
9.4.3 Bronchopulmonary Sequestration (BPS)
9.4.3.1 Definition
BPS is a congenital anomaly of the lung characterized by a mass of nonfunctional lung tissue, usually affecting the lower lobes, without communication with the bronchial tree. Arterial supply to the mass is systemic and usually originates from the aorta, either lower thoracic or upper abdominal aorta. Other origins such as from the gastric artery and splenic artery can also occur [47].
9.4.3.2 Classification
BPS can be classified based on anatomical characteristics either intralobar (75 %) or extralobar (25 %) [48]. An intralobar lesion shares the visceral pleura of the rest of the normal lung, and venous blood drains into the pulmonary venous system; extralobar sequestration has its own visceral pleura, drains into the systemic veins, and is most commonly diagnosed in the prenatal or perinatal period [37, 48].
Based on location, the majority of BPS are supradiaphragmatic (85 %), and the remaining cases are either diaphragmatic or infradiaphragmatic (10 %) [37, 49]. Description of the location is important in order to provide a better differential diagnosis and follow-up evaluation; subdiaphragmatic lesions must be differentiated from neuroblastomas and adrenal hemorrhage [37, 48].
When a BPS coexists with a CPAM, it is called a hybrid lesion, and they can have both systemic and pulmonary arterial feeders (Fig. 9.9). 25–50 % of extralobar sequestrations are hybrid lesions [36].
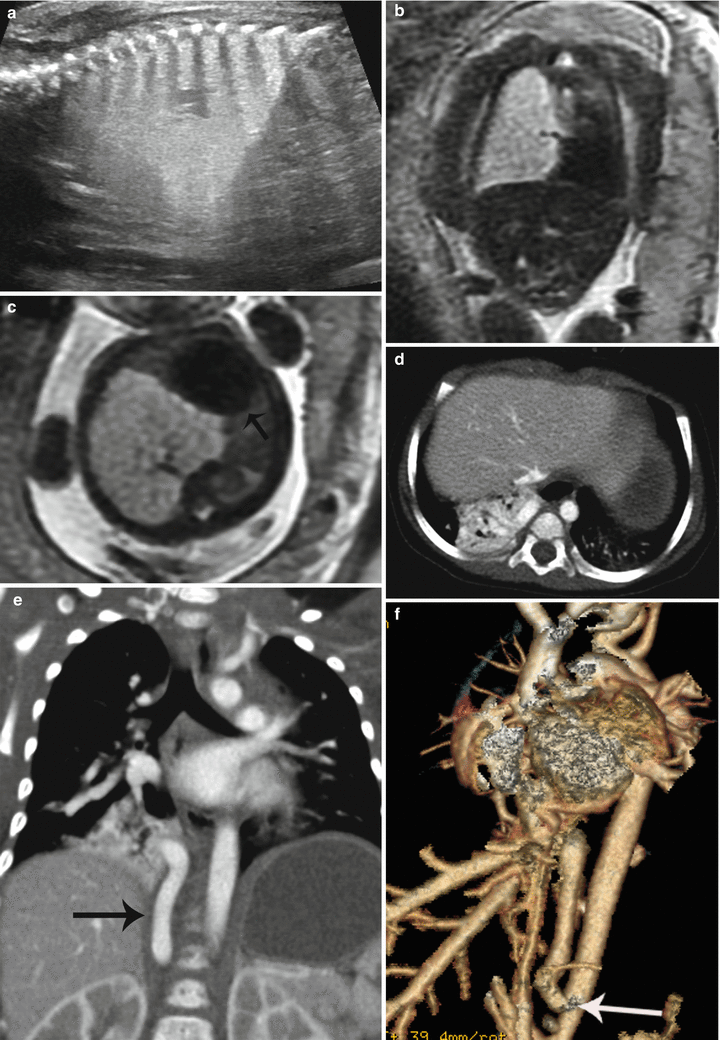
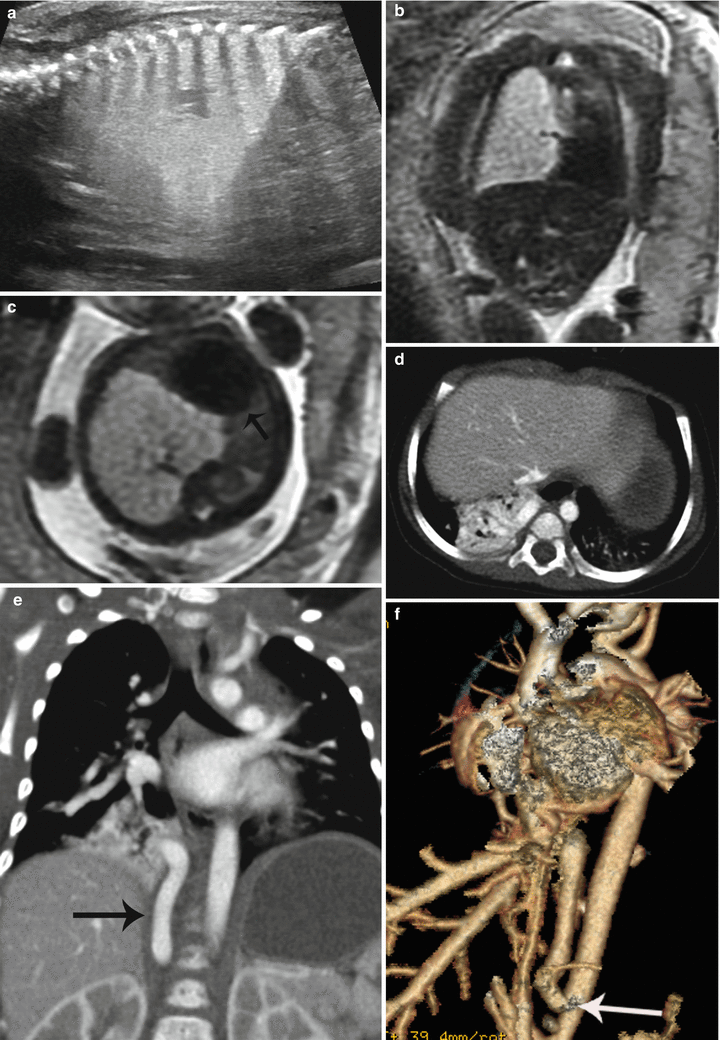
Fig. 9.9
Hybrid CPAM BPS at 23 weeks gestation. (a) Sagittal US image demonstrates a large echogenic mass inverting the diaphragm. A few macrocysts are noted centrally. (b) Coronal and (c) axial SSFSE MR images demonstrate a high-signal mass deviating the heart to the right (arrow). CVR was 1.7. No definite systemic feeding vessel was identified. Patient was asymptomatic at delivery. (d) Axial CT at 6 months of age demonstrates a vascular mass in the right lower lobe. (e) Coronal reconstruction reveals a large infradiaphragmatic systemic feeding vessel (arrow) coursing into the mass. (f) 3D reconstruction demonstrates a large feeding vessel (arrow) branching off the celiac axis
9.4.3.3 Imaging Findings
MRI demonstrates a well-defined, wedged-shaped lung mass that is hyperintense on T2-weighted sequences. A low-signal linear structure representing the lung mass feeder can sometimes be seen by MRI but is usually better seen by ultrasound, by power and color Doppler (Fig. 9.10). MRI is beneficial in the delineation and better localization of the mass. MRI also allows for better evaluation of the contralateral lung and in the assessment of associated congenital anomalies.
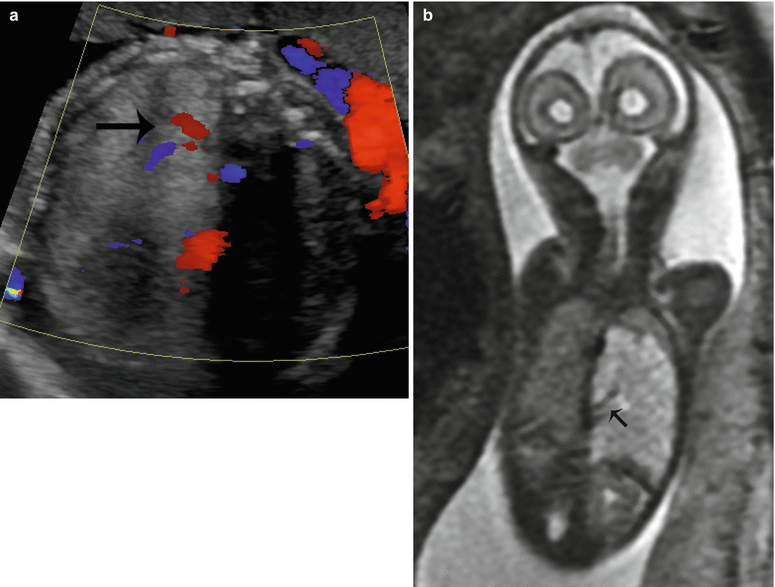
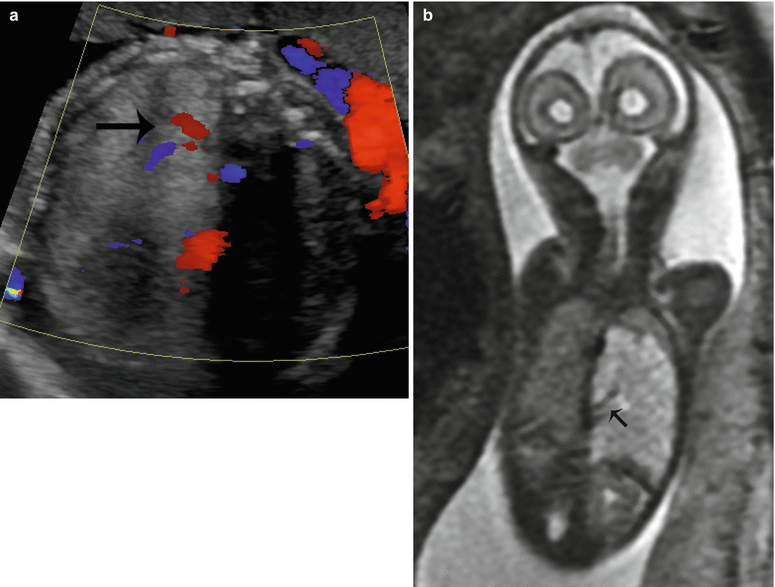
Fig. 9.10
Bronchopulmonary sequestration at 27 weeks gestation. (a) Axial US demonstrates an echogenic solid mass in the left lower lobe with a systemic feeding vessel (arrow) branching from the descending aorta. (b) Coronal SSFSE T2w confirms the left lower lobe mass with systemic feeding vessels (arrow) coursing into the mass
Follow-up MRI may demonstrate a smaller lesion with hypointense T2 signal compared to the lung if the lesion either decompressed into the lung, outgrew the systemic circulation, or had a vascular/lymphatic obstruction due to torsion [50].
9.4.3.4 Prognosis and Fetal Management
Prognosis depends on the size of the lesion and presence of hydrops. When the mass is large, it can cause mass effect on the mediastinal structures. If significant compression is exerted on the heart and venous structures, hydrops can occur; if the esophagus is compressed, polyhydramnios can be seen [41, 50, 51]. Most have a good prognosis since the majority decreases in size in utero (75 %), and hydrops is rarely seen [4, 41, 48, 50]. If hydrops develops as a complication, the survival decreases to 20–30 % [50].
Serial follow-up examinations should be performed. The majority (75 %) of prenatally diagnosed BPS decreases in size and becomes harder to see by ultrasound, but an increase in size can also be seen. Follow-up examinations are performed with ultrasound for reevaluation of the lesion, change in size, variation in mediastinal compression, and presence of hydrops. Closer follow-up is recommended for larger lesions given with higher risk for hydrops. Late MRI can be performed to better delineate the feeding vessels, with a better correlation with postnatal imaging [52]. In some institutions, postnatal imaging is not performed if prenatal MRI properly depicts the feeding vessel for surgical planning.
Thorough examination of the entire fetus should be performed looking for associated congenital anomalies, especially in the presence of a suspected extralobar type. Fetal karyotype should be recommended if fetal intervention could be considered in the future management.
Fetal intervention is indicated in the presence of hydrops earlier in pregnancy and significantly increases survival [51, 53]. If hydrops develops after weeks 32–34, early delivery and postnatal management is an option [41].
9.4.4 Congenial Lobar Overinflation (CLO)
9.4.4.1 Definition
CLO is a rare entity characterized by overinflation of the lung. It is usually unilateral and usually affects one lobe; involvement of more pulmonary lobes [63] and bilateral lesions has been described.
In neonates who present with respiratory distress, the left upper lobe is most commonly affected followed by right middle and right upper lobes; in prenatal cases, a predisposition for lower lobe involvement and a high association with bronchial atresia have been seen [37]. It is also known as congenital lobar emphysema, though no damage to the alveolar wall is appreciated [37].
9.4.4.2 Classification
CLO can be idiopathic in about 50 % of cases [63]. The remaining 50 % can be extrinsic or intrinsic in origin. Intrinsic factors include cartilaginous deficiency, bronchial stenosis/atresia, or mucus plugs. Extrinsic causes include extrinsic compression from an extrabronchial mass or vascular structure such as dilated pulmonary arteries in tetralogy of Fallot with the absent pulmonary valve (Fig. 9.11).
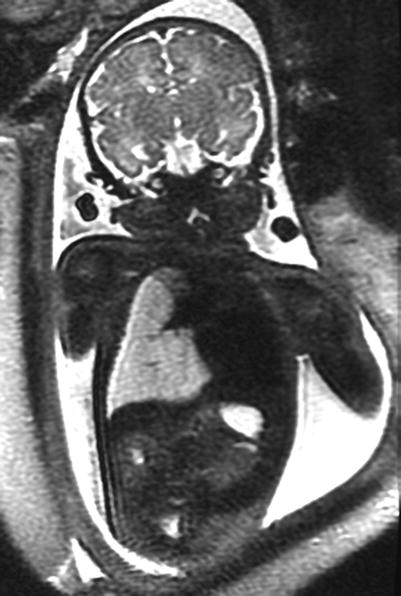
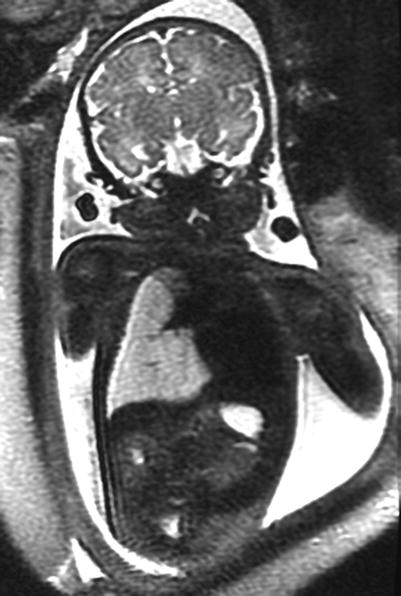
Fig. 9.11
Congenital lobar overinflation at 35 weeks gestation. Coronal SSFSE T2w image demonstrates a hyperexpanded high-signal right lower lobe deviating the heart to the left with flattening of the right hemidiaphragm. The fetus had tetralogy of Fallot with absent pulmonary valves causing trapping of fluid
9.4.4.3 Imaging Findings
CLO is characterized as an area of increased T2 signal with a lobar distribution; it is usually more homogeneous than CPAM and less hyperintense when compared to BPS [48]. Lung structure appears intact with stretched hilar vessels [64].
9.4.4.4 Prognosis and Fetal Management
Echocardiogram should be performed to exclude congenital heart disease. 14 % of patients have an associated cardiac anomaly, most likely a large left to right shunt with pulmonary hypertension [65].
Prenatal outcome varies from spontaneous resolution to progression with increase in size and increased intrathoracic pressure resulting in impaired fetal circulation and swallowing with hydrops and polyhydramnios. Occasionally, resolution is suspected prenatally, but a persistent, nonvisualized mass could result in neonatal respiratory insufficiency due to presence of overinflation and valve mechanism [63].
The main indicator of prenatal outcome is the presence of hydrops, and follow-up examinations should be performed to assess changes and presence of hydrops.
9.4.5 Congenital Hydrothorax
9.4.5.1 Definition
The presence of pleural fluid is considered abnormal in the fetus. It can be seen in isolation or in association with a lesion, such as CPAM, BPS, lymphangiectasia, cardiac anomalies, infections including TORCH, and chromosomal abnormalities like Turner syndrome and trisomy 21.
9.4.5.2 Classification
Hydrothorax can be primary or secondary. Primary hydrothorax is rare and affects males more frequently. It results from an anomaly in the development of the lymphatic system resulting in chylous leak into the pleural space and can be unilateral or bilateral [67, 68]. Primary effusions are characterized by having high pressures, resolving rapidly after thoracentesis and developing hydrops restricted to the upper fetal body [68]. Secondary hydrothorax is seen in hydrops-related pathologies or pulmonary masses that can cause mass effect and compression.
9.4.5.3 Imaging Findings
When a hydrothorax is present, MRI demonstrates an area of bright T2 signal surrounding the lung parenchyma. It can be small or large and unilateral or bilateral and can remain stable, resolve, or progress to hydrops. Depending on the size, mediastinal shift and lung compression can occur (Fig. 9.12). MRI may help identify associated abnormalities not seen by ultrasound, like an inconspicuous CPAM or BPS.
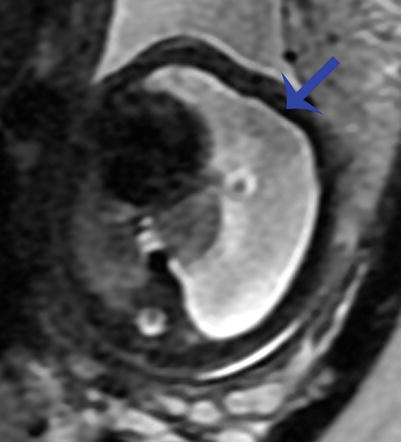
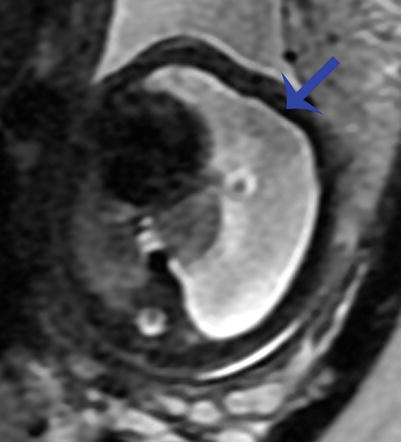
Fig. 9.12
Congenital hydrothorax at 30 weeks gestation. Axial SSFSE image of the chest demonstrates a large left pleural effusion (arrow) deviating the heart to the right and compressing the ipsilateral lung. There is compression of the contralateral right lung as well
Depending in the content of the pleural fluid (lipid, protein, or blood products), different signal intensities might be seen with MRI in both T1- and T2-weighted images that may help establish the etiological diagnosis [7].
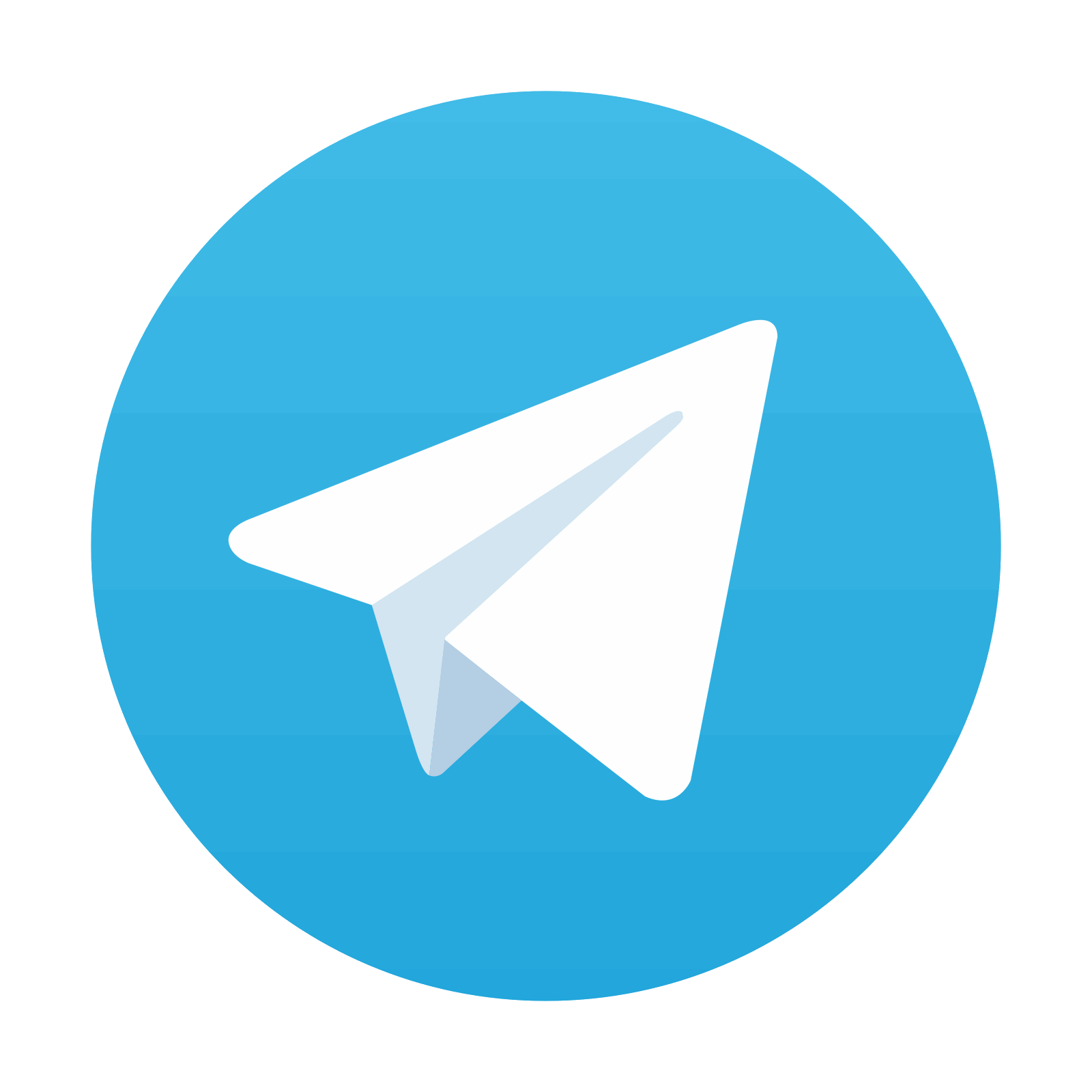
Stay updated, free articles. Join our Telegram channel

Full access? Get Clinical Tree
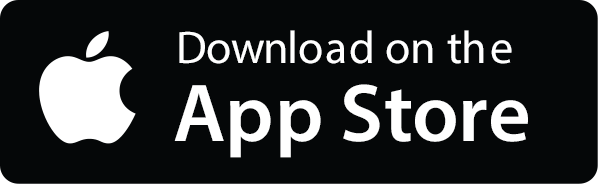
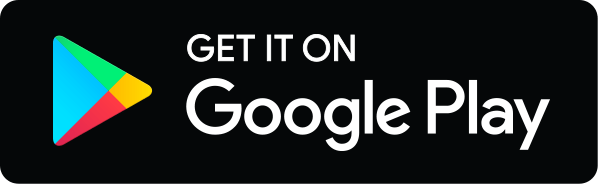