Multislice CT and MRI of the Thoracic Aorta
Gautham P. Reddy
Martin Gunn
Lee M. Mitsumori
Michael B. Gotway
Charles B. Higgins
INTRODUCTION
The aorta can be imaged with a number of modalities: x-ray angiography, transesophageal echocardiography (TEE), multislice computed tomography (MSCT), and magnetic resonance imaging (MRI). Because some diseases of the thoracic aorta are life threatening, the aorta must be fully evaluated when pathology is suspected. For comprehensive diagnostic assessment of the thoracic aorta, the lumen, aortic wall, and periaortic region must be depicted to assess intraluminal, mural, and extramural disease. Aortic pathology can involve any or all of these three locations. Imaging techniques must have the capability of evaluating the entire thoracic aorta as well as the origins of the arch vessels to define the extent of involvement. In some patients the status of the aortic valve and annulus must also be delineated. Imaging studies are used both for the initial diagnosis and for the surveillance of disease progression over time.
MSCT and MRI are the mainstays of evaluation of the thoracic aorta. The use of MSCT or MRI is dependent upon the information required, the clinical status of the patient, history of contrast reaction, and institutional practice.
The advantages of MSCT are its speed, ease of use, and lack of operator dependence. At many institutions, MSCT is the examination of choice for evaluation of acute aortic pathology, including dissection, intramural hematoma, aneurysm rupture, penetrating ulcer, and acute traumatic injury. MSCT can be used to evaluate the lungs and pleura in patients with suspected aortic disease, a capability that can be especially important in the acute setting because vascular and nonvascular diseases can have similar clinical presentations and may be difficult to differentiate on the basis of signs and symptoms. At many centers, MSCT is preferred for acutely ill patients and MRI for patients who are stable.
MRI is a valuable technique for comprehensive noninvasive morphologic and functional evaluation of the thoracic aorta. MRI has several advantages, including high intrinsic contrast between the blood pool and vessel wall, a wide range of soft tissue contrast that allows the delineation of the vascular and perivascular structures, the ability to evaluate both morphology and function of the vascular system, multiplanar imaging, avoidance of iodinated contrast agent, and absence of ionizing radiation. Because of its robustness, MRI remains the optimal method of evaluating aortic disease in patients who are not acutely ill. MRI can serve as a complementary imaging modality in acute aortic syndromes when CT is contraindicated (such as in patients with renal failure) or when CT does not provide complete evaluation of the disease process.
TECHNIQUES
MSCT
MSCT techniques have evolved rapidly, as scanners with additional detector rows have become available. Nonetheless, certain variables must be optimized to yield the highest image quality: collimation, pitch, field-of-view, reconstruction increment, amount, rate and timing of contrast administration, distance to be scanned, mA, and tube rotation time. Occasionally, a compromise among these parameters is necessary to optimize the scan.
MSCT of the thoracic aorta usually begins with a noncontrast series. Slice thickness of 5 mm is sufficient for the unenhanced scan, which is followed by the contrast-enhanced scan.
Optimal postcontrast imaging requires a slice thickness of 0.6 to 2.5 mm, which provides excellent spatial resolution and allows for high quality reconstructed images. A pitch of 1.375 to 2 allows a large volume of coverage to be obtained rapidly. The field of view should include at least the outer rib to outer rib at the widest portion of the thorax.
There is no consensus regarding the iodine concentration that should be used for MSCT. Undiluted (300 or 360 mg I/mL) contrast agent is usually appropriate. Approximately 80 to 140 mL of contrast agent is injected at a rate of 3 to 5 mL/s. These rates can be achieved using a 20-gauge intravenous catheter. A standard scan delay of 25 seconds after start of injection will usually suffice for MSCT. However, patients with diminished cardiac function or an unsuspected stenosis of the injected vein may require longer delays to achieve optimal opacification of the vessel. Therefore, either a test
bolus to determine contrast transit time, or bolus triggering software allow for synchronization of CT acquisition with optimal contrast opacification in nearly all patients.
bolus to determine contrast transit time, or bolus triggering software allow for synchronization of CT acquisition with optimal contrast opacification in nearly all patients.
The contrast-enhanced MSCT should extend from the thoracic inlet to below the aortic bifurcation in the pelvis, to assess the possibility of pathology extending into the arch vessels and iliac arteries.
The mA used is critical for obtaining high quality studies. Values of 120 to 150 mA per tube rotation will usually suffice for most patients, but heavier individuals may need an upward adjustment in the mA. Automated exposure algorithms that permit axial and longitudinal (XYZ) dose modulation exist for most scanners with nonelectrocardiographic (ECG) synchronized helical acquisitions. The use of prospective ECG gating will reduce motion artifact and should be used when available. MSCT of the aorta is best achieved with the shortest available tube rotation time, as low as 330 to 350 ms with currently available machines.
MRI
Several MRI techniques are useful for imaging the thoracic aorta. The most important sequences are ECG-gated black blood double inversion recovery and white blood steadystate free precession (SSFP) cine sequences as well as contrast-enhanced three-dimensional (3D) MR angiography (MRA). With respect to the long axis of the aorta, images can be acquired parallel (sagittal oblique or sagittal) or perpendicular (transverse). Use of orthogonal planes helps to distinguish among intraluminal, mural, and extramural pathologies and reduces partial volume averaging. ECG gating is used for black blood and SSFP cine acquisitions but not for MRA.
A typical protocol for imaging of the thoracic aorta consists of black blood, SSFP cine, and MRA sequences. Transverse images are acquired from a level several centimeters above the aortic arch to the level of the diaphragm, using a slice thickness of approximately 5 mm. Transverse breath hold SSFP cine MRI is performed in the same anatomic region. A slice thickness of 4 to 6 mm is used for the cine sequence. A black blood inversion recovery sequence is acquired in the oblique sagittal or sagittal plane (slice thickness 3 to 5 mm) to display the entire length of the aortic arch, along with the ascending aorta and proximal descending aorta, on the same image. This sequence may be useful to visualize the origins of the arch vessels, which is important when aortic dissection is suspected. The oblique sagittal imaging sequence is prescribed from the transverse image at the level of the aortic arch. Contrast-enhanced 3D MRA is performed in the sagittal or oblique sagittal plane to reduce the number of phase encodes required, resulting in shorter acquisition times and/or better spatial resolution.
For black blood imaging, double inversion recovery technique is used to suppress the signal of the intraluminal blood. The aortic lumen is therefore homogeneously black. Although this technique can be valuable, the luminal signal is not completely black when flow is inordinately slow.
SSFP sequences render the blood pool homogeneously bright. These sequences are especially useful for aortic evaluation because they demonstrate intraluminal pathology as a “filling defect” in the bright blood pool. The bright signal of the blood on SSFP images is secondary to “flow-related” enhancement. With SSFP imaging radiofrequency (RF) pulses are applied to saturate a volume of tissue. Because the TR is short, there is little time for signal recovery from stationary tissue. Therefore the maximum signal is emitted by blood flowing into the volume of tissue, because this blood contains the only protons that have not been saturated by the RF pulses. Slow blood flow, which results in decreased flow enhancement, is the primary factor that causes signal depression on these images. Signal loss also can occur as a consequence of disturbed flow caused by intravoxel phase dispersion, such as occurs with turbulence from a flow jet.
In the thorax, SSFP sequences are frequently performed as breath hold cine acquisitions. SSFP cine images are acquired at multiple contiguous slice locations. An ECG signal is acquired along with the imaging data so that images can be reconstructed at multiple phases (frames) of the cardiac cycle. Generally at least 16 to 32 frames are acquired at each anatomic level.
Three-dimensional MRA with intravenous gadolinium chelate contrast agent relies on the T1-shortening effect of gadolinium to produce high-signal intensity in blood vessels, thereby avoiding flow-related artifacts. The protocol for 3D MRA varies from institution to institution. One of the most effective prescribes MRA in the sagittal or oblique sagittal planes. ECG gating is not required to perform this sequence. Thirty to sixty images are typically obtained using a slice thickness of 1 to 2 mm.
The MRA sequence is performed during a breath hold of approximately 15 to 25 seconds. The patient can be provided with oxygen by nasal cannula, at a rate of 2 L/min, to aid in breath holding. The contrast agent is administered via an antecubital vein with use of a power injector (2 mL/s, 0.1 mmol/kg total). Timing of the acquisition can be optimized by using real-time imaging for bolus detection or by performing a preliminary acquisition employing a test bolus to estimate circulation time.
Image postprocessing of the CT and MRI data sets can include:
maximum intensity projection; uses include calcifications and small vessels (such as collaterals)
multiplanar reformations and curved planar reformation; uses include measurement of perpendicular aortic dimensions and centerline lengths for graft planning
volume rendering; uses include 3D display of aortic course, and extent of disease involvement
NORMAL RANGE OF THORACIC AORTIC DIMENSIONS
The sizes of the thoracic aorta (mean and standard deviation) as measured on axial MRI or CT images are:
Sinus of Valsalva 3.3 ± 0.4 cm
Mid-ascending aorta 3.0 ± 0.4 cm
Proximal descending aorta 2.4 ± 0.4 cm
These normal measurements were determined in a population of young adults.
THORACIC AORTIC ANEURYSM
Aortic diameter exceeding 4 cm is called dilatation, and a diameter over 5 cm is termed an aneurysm. The maximum diameter of the aorta is an important determinant of the risk of rupture. When the aortic diameter is more than 6 cm, the risk of rupture in the next 5 years is greater than 30%.
Thoracic aortic aneurysms vary in shape and size. Saccular aneurysms are localized and do not involve the entire circumference of the aorta (Fig. 29-1). Fusiform aneurysms involve the entire aortic circumference and may extend over a great length of the vessel (Fig. 29-2). Annuloaortic ectasia results from cystic medial necrosis, which is associated with Marfan syndrome; although it can be idiopathic. In annuloaortic ectasia the aortic root, and often the entire ascending aorta, is enlarged (Figs. 29-3 and 29-4). Many aneurysms involving the thoracic aorta also involve the abdominal aorta.
In a true aneurysm, all three layers of the aortic wall are intact, whereas a false aneurysm results from a focal disruption of the aortic wall contained by the adventitia and surrounding fibrous tissue. Common causes of false aneurysm are trauma (Fig. 29-5), infection, and surgery (Fig. 29-6). True aneurysms most frequently are secondary to atherosclerosis. Other etiologies of true aneurysms include connective tissue disorders such as Marfan syndrome, arteritis (Takayasu or giant cell arteritis), idiopathic cystic medial necrosis, relapsing polychondritis, complications of aortic valve disease, and aneurysm of the ductal remnant.
Mycotic aneurysms result from infection of the aortic wall and occlusion of the vasa vasorum by septic emboli; this causes weakening of the wall resulting in aneurysm formation. Etiologies of mycotic aneurysm include septic emboli from intravenous drug use and indwelling catheters, infection of prosthetic valves, or infection of atherosclerotic plaque. Common infectious agents include Salmonella, Staphylococcus aureus, streptococci, tuberculosis, and fungi, such as Candida and Aspergillus. Mycotic aneurysms are usually saccular, and they may grow quickly. Mycotic aneurysms can be associated with periaortic fat infiltration and inflammation or gas formation. Mycotic aneurysms are at high risk for rupture.
Imaging Evaluation of Aneurysm
In the imaging evaluation of thoracic aortic aneurysm, the primary goals are measurement of the maximum diameter of the aorta, demonstration of the extent of the aneurysm, identification of branch vessel involvement, compression of adjacent structures, and detection of periaortic hematoma. The demonstration of mural thrombus is important in patients who present with peripheral embolization.
MSCT findings of aortic aneurysm rupture include high-density fluid in the wall of the aorta, pleural space, or pericardium
(Fig. 29-7). Mediastinal hematoma also suggests the possibility of aneurysm rupture. The “draped aorta” sign may indicate an early, contained rupture. This sign manifests as indistinctness of the posterior wall or close alignment of the posterior wall with the contour of the adjacent vertebral bodies.
(Fig. 29-7). Mediastinal hematoma also suggests the possibility of aneurysm rupture. The “draped aorta” sign may indicate an early, contained rupture. This sign manifests as indistinctness of the posterior wall or close alignment of the posterior wall with the contour of the adjacent vertebral bodies.
MRI also readily depicts the size and extent of an aneurysm of the ascending or descending thoracic aorta. The outer dimension of the aneurysm and the size of the patent lumen can be seen. Because it can demonstrate the outer wall of the aorta, unlike angiography, MRI allows accurate measurement of the aneurysm diameter even when there is wall thickening or mural thrombus. Mural thrombus and atherosclerotic plaque are depicted as eccentric or concentric thickening of the aortic wall. The patent lumen can usually be determined
by the flow void on black blood MRI. However, slow flow can produce intraluminal signal on black blood images. Slow flow can be differentiated from thrombus with SSFP cine MRI or contrast-enhanced MRA. MRA is especially effective for demonstrating the extent of thoracic aortic aneurysm, the relationship to the aortic branches, and the precise dimensions—especially in the aortic arch.
by the flow void on black blood MRI. However, slow flow can produce intraluminal signal on black blood images. Slow flow can be differentiated from thrombus with SSFP cine MRI or contrast-enhanced MRA. MRA is especially effective for demonstrating the extent of thoracic aortic aneurysm, the relationship to the aortic branches, and the precise dimensions—especially in the aortic arch.
![]() FIG. 29.4. Annuloaortic ectasia in a patient with Marfan syndrome. Note the marked, diffuse enlargement of the aortic root and ascending aorta (A). |
Identification of periaortic or mediastinal hematoma is vital in the evaluation of aneurysm rupture. On black blood images hematoma appears as an area of high-signal intensity, although this appearance may not be seen early. It may produce intermediate signal intensity during the first few hours after bleeding. MRI can delineate the extent of the hemorrhage, which may spread through the mediastinum or be localized to the periaortic region, pleural space, subpleural space, or pericardium.
MRI is an effective noninvasive means of monitoring the progression of thoracic aortic aneurysms. If the aortic diameter is greater than 6.0 cm or if there is a rapid increase in diameter, surgical repair is generally necessary. Because it is noninvasive and accurate, MRI is also the optimal method for sequential follow-up of the aorta in patients with a high risk for developing dissection, such as those with Marfan or Ehlers-Danlos syndromes (see Fig. 29-3).
False aneurysms can be revealed by MSCT, contrast-enhanced MRA, or a combination of transverse and sagittal or sagittal oblique MR images. MRI is useful for depiction of
the longitudinal extent of the false aneurysm. Post-traumatic false aneurysms seen on MSCT and MRI are most frequently located at the site of ligamentum arteriosum and involve either the entire aortic circumference or only the anterior wall (see Fig. 29-5).
the longitudinal extent of the false aneurysm. Post-traumatic false aneurysms seen on MSCT and MRI are most frequently located at the site of ligamentum arteriosum and involve either the entire aortic circumference or only the anterior wall (see Fig. 29-5).
ACUTE AORTIC SYNDROMES
Aortic Dissection and Intramural Hematoma
Aortic dissection is a separation of the aortic wall that results from a tear in the intima. Blood can enter the aortic wall through an intimal disruption and extend proximally and distally in the media, displacing the intima inward. The dissection may reenter the lumen at one or more sites of fenestration. Typically blood flows in both the true and false channels, although the false channel is sometimes thrombosed. The dissection may disrupt the adventitia and cause pericardial tamponade, exsanguination, pleural hemorrhage, or periaortic hematoma. If the dissection involves or occludes branches of the aorta, cerebral ischemia, myocardial infarction, renal insufficiency, or mesenteric infarction can ensue.
Aortic dissection can result from an intramural hematoma, which is caused by rupture of the vasa vasorum, the arteries that supply the aortic wall. The ruptured vasa vasorum bleed
into the aortic wall, which can result in an intimal tear and separation of the wall. If the intima does not rupture, causing a frank dissection, then an intramural hematoma remains. The intramural hematoma may be localized, or it can extend along the wall in antegrade or retrograde direction or sometimes rupture through the adventitia. An intramural hematoma can be considered to be a form of dissection, and management is similar to that of a frank dissection.
into the aortic wall, which can result in an intimal tear and separation of the wall. If the intima does not rupture, causing a frank dissection, then an intramural hematoma remains. The intramural hematoma may be localized, or it can extend along the wall in antegrade or retrograde direction or sometimes rupture through the adventitia. An intramural hematoma can be considered to be a form of dissection, and management is similar to that of a frank dissection.
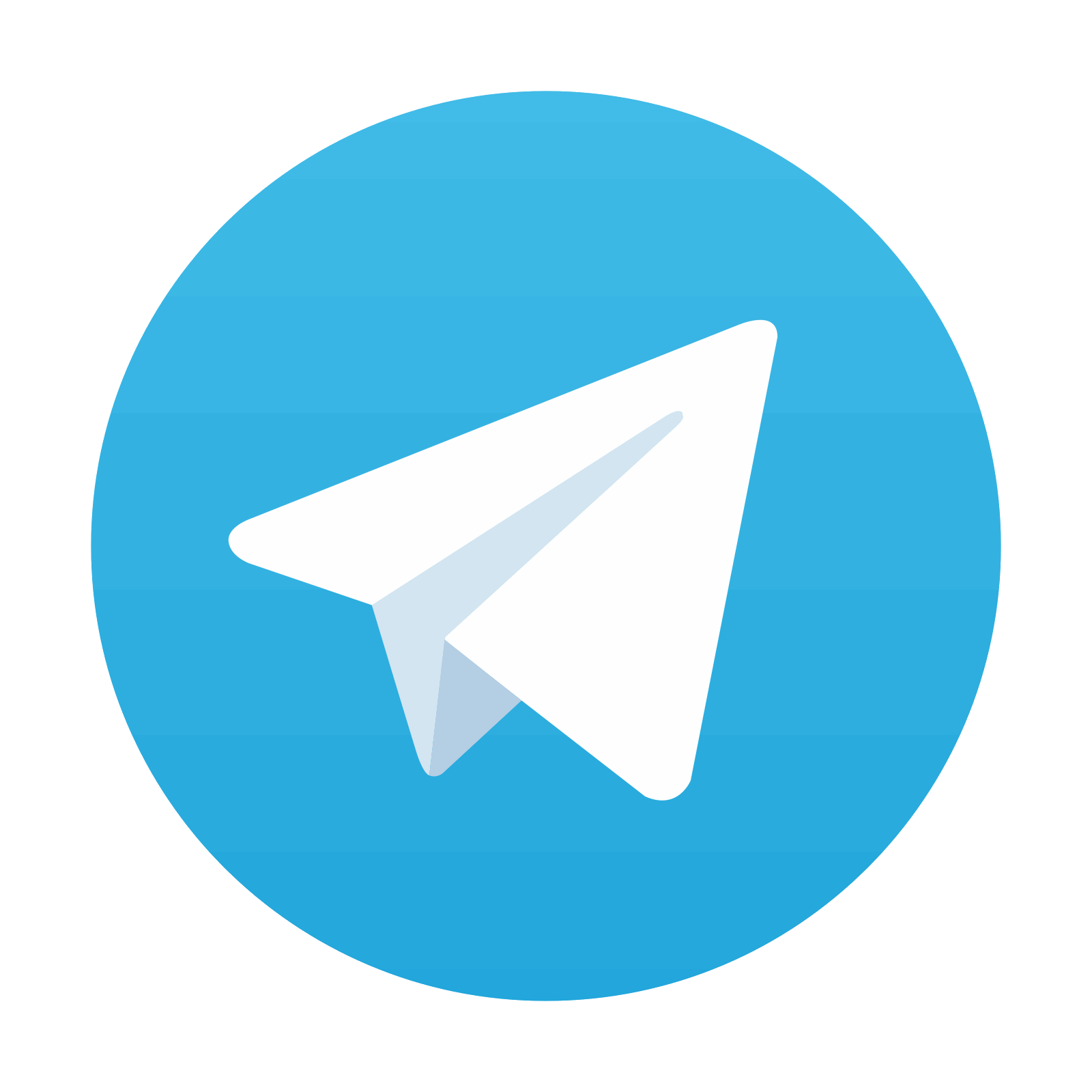
Stay updated, free articles. Join our Telegram channel

Full access? Get Clinical Tree
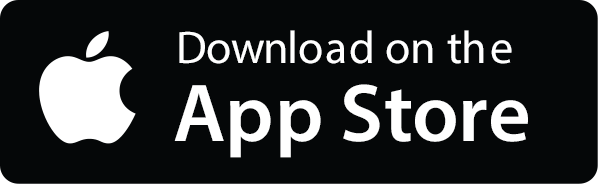
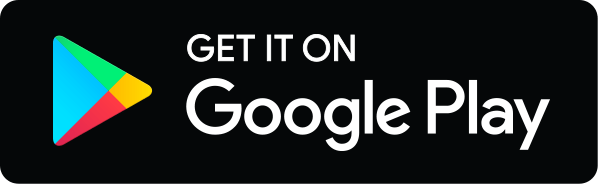