Muscles
Stefano Bianchi
Jean-Louis Brasseur
Gerard Morvan
Lionel Pesquer
Dien Hung Luong
INTRODUCTION
The elementary structure shared by every skeletal muscle is the myofiber, which is enveloped by a thin layer of connective tissue called the endomysium. Muscle fibers are of two types: type I and type II. Type I fibers, the red myofibers, are smaller and more apt to prolonged contractions, while the larger type II fibers are responsible for powerful contractions of short duration. All skeletal muscles contain both types of fibers but the proportions differ depending on functional demands. Myofibers are packed together to form muscle fascicles that are surrounded by the perimysium, fibroadipose septa that contain vessels and nerves. Groups of fascicles form muscles that are surrounded by a thick epimysium.
When muscles contract, the forces generated by the fascicles are transmitted to fibroadipose septae and then to internal tendons or laminae that converge on the distal tendon or tendon laminae. Muscle fibers parallel to the axis of muscle contraction provide the maximum range of muscle excursion, while oblique fibers have a shorter range but increase the force of contraction. Muscle size, shape, and internal architecture reflect mainly functional requirements.1,2,3,4 Muscles vary in size from a few millimeters to very long muscles such as the sartorius, the longest muscle of the human body.
Ultrasound Technique of Examination and Normal Ultrasound Appearance
A short clinical history and a basic physical examination are always performed before ultrasound (US) examination of muscles.1 Data concerning the onset and location of symptoms, the presence of a mass, ecchymosis, or local tenderness on palpation or muscle contraction are required to target the zone of interest and aid interpretation of the ultrasound findings.
For the lower limbs, the patient lies supine or prone. For the upper limbs, the patient sits with the arm on the examination couch and the limb positioned according to the region of interest. Special positions, such as squatting for easier detection of herniation of anterolateral leg muscles, can be used when appropriate.
The frequency of the transducer must be adapted to the depth and size of the muscle. For superficial muscles, 10 to 17 MHz transducers are used, whereas 3.5 to 7 MHz transducers are necessary for deeper structures.3,4,5 Focus adjustment for depth optimizes spatial resolution. Power or color Doppler allows assessment of vascularity. Power Doppler may be more sensitive than color Doppler, but this seems to depend on the hardware adjustments. Pulsed Doppler, sonoelastography, and three-dimensional or fusion imaging are not used in daily routine. Appropriate annotations are required to allow better understanding of ultrasound images by clinicians, 3 including the name of the region, side, anatomic landmarks, and the scanning plane.
Muscles must be examined in their longitudinal and axial planes (Fig. 11.1). The transducer must be perpendicular to the muscle fibers to avoid anisotropy, which can lead to decreased echogenicity of the perimysium, internal septa, aponeuroses, and tendons. In doubtful cases, tilting the transducer can show that the hypoechoic appearance is related to anisotropy. Examination during muscle contraction also reduces artifacts by modifying the orientation of the septa (Fig. 11.2). Beam steering can reduce artifactual anisotropy. Harmonic and compound imaging improve the signal-to-noise ratio and tissue contrast.6 Panoramic scans allow better comprehension of images by clinicians and improve the accuracy of measurements (Fig. 11.3).7
Local tenderness caused by increasing pressure with the transducer helps to focus the ultrasound examination toward the area of interest. Transducer pressure may reduce the thickness of the subcutaneous tissues and improve the assessment of underlying structures, but pressure can efface fluid collections and muscle hernias. Dynamic ultrasound performed during muscle contraction and relaxation helps to detect subtle muscle injuries and muscle hernias, analyze fibrous scars, and reduce anisotropy.1,8
Ultrasound examination must include not only the whole muscle, including its proximal and distal insertions, but also adjacent muscles. Contralateral examination helps in assessing small or doubtful lesions, unilateral muscle agenesis or atrophy/hypertrophy, and allows better evaluation of anatomic variations.
Ultrasound of normal muscles shows regular alternation of myofibers and connective tissue components.1,2,3,4,5 On longitudinal sonograms, myofibers appear as hypoechoic structures separated by hyperechoic thinner bands reflecting the perimysium (fibroadipose septa). The overall appearance is regular alternation of parallel hypo-and hyperechoic bands. Axial images of muscles show hypoechoic structures containing short echoic bands or dots due to the perimysium. Peripheral muscle fascia appears as thick hyperechoic bands on both planes. Vessels and nerves run through focal interruptions of the fascia. When correctly examined, internal and terminal aponeuroses and tendons are hyperechoic on both axes but appear hypoechoic if the transducer is not perpendicular.
ACCESSORY MUSCLES
General Considerations
Accessory muscles (AMs) are congenital anomalies9,10,11,12,13,14,15,16 that are usually asymptomatic and go unnoticed but can be clinically relevant. Frequently they present as asymptomatic masses that can be confused with soft tissue tumors.17
If located in fibro-osseous tunnels, they can cause neurologic symptoms due to nerve compression.18,19,20,21,22,23 In active patients, they can cause pain during exercise due either to ischemia related to increased intrafascial pressure or to overuse tendinopathy.
If located in fibro-osseous tunnels, they can cause neurologic symptoms due to nerve compression.18,19,20,21,22,23 In active patients, they can cause pain during exercise due either to ischemia related to increased intrafascial pressure or to overuse tendinopathy.
The diagnosis of AMs is based mainly on recognition of the typical location and imaging features. Although AMs are congenital, patients often report them as new masses. This can be explained by two mechanisms: hypertrophy due to sports activity, particularly in young patients, and awareness of a swelling after weight loss and thinning of the subcutaneous fat. A clinically evident AM appears as an indolent soft tissue mass covered by normal skin. Signs of inflammation and adhesions to the skin are absent. If the diagnosis is suspected on inspection or palpation, muscle contraction increases firmness, as in normal muscles.
Since AMs are made of normal muscle tissue, they often present at imaging as small, bulky masses with regular internal organization identical to adjacent muscles. When they present as masses, the diagnosis is relatively easy if the possibility of AM is kept in mind and an appropriate imaging modality is used. In other presentations, a high degree of clinical suspicion is necessary to detect smaller AMs. In doubtful cases, bilateral examination can help in demonstrating normal contralateral anatomy, although the rare possibility of bilateral AMs must be kept in mind.
Standard radiographs are of limited value. Computed tomography (CT) shows a mass with density and shape similar to that of adjacent muscles. At magnetic resonance imaging (MRI), anomalous muscles resemble normal muscle morphology and signal/texture on all sequences including after gadolinium injection. Tendons can be detected by ultrasound and MRI, thus confirming the diagnosis.
Knowledge of the appearance and typical locations of AMs is necessary to avoid confusion with pathologic masses and unnecessary biopsy or surgery.
Upper Extremity
Shoulder
AMs found at the anterior aspect of the shoulder include accessory heads of the biceps, coracobrachialis, and subscapularis muscles.24 The AM located on the anterior surface of the subscapularis muscle has the most clinical importance24 as it may cause problems at surgery if it covers the anterior circumflex artery, the main nutrient vessel of the humeral head. To avoid damage, it is necessary to locate the artery by splitting the subscapularis AM belly. The muscle inserts into the anterior aspect of the lateral part of the subscapularis tendon and extends inferiorly. Ultrasound shows the muscle position, size, and internal structure (Fig. 11.4) and by using color Doppler, defines the precise location of the anterior circumflex artery and its relation to the subscapularis AM. This can be important for presurgical planning.
Elbow
Anconeus epitrochlearis (AE) is a small, often bilateral, AM, and its prevalence is estimated from 1% to 34%. In a recent review of asymptomatic subjects, the AE muscle was seen on MRI in 23% of individuals.25 It originates from the posterior aspect of the medial condyle of the humerus and inserts into the medial aspect of the olecranon. As it extends into the cubital tunnel, it can compress the ulnar nerve (UN), particularly during full elbow flexion. Chronic intermittent pressure on the nerve occurring during repetitive flexion and extension of the elbow can cause intraneural edema and subsequently internal fibrotic changes responsible for ulnar neuropathy.26
Due to its small size, the AE cannot be palpated at physical examination, and imaging is required. Standard radiographs are useful in excluding osteoarthritic changes, the main cause of ulnar neuropathy at the level of the
cubital tunnel, but are useless in detection of the AE. Ultrasound detects the AE and also evaluates the UN. Due to its superficial location, the AE is easily identified by ultrasound (Fig. 11.5). On axial scans, the AE appears as a hypoechoic structure located superficial to the UN in the cubital tunnel. Scanning during progressive elbow flexion may show anterior displacement of the muscle and impingement on the posterior aspect of the UN. Internal UN changes secondary to long-standing friction include swelling, irregular hypoechoicity, and loss of the normal internal fascicular pattern.
cubital tunnel, but are useless in detection of the AE. Ultrasound detects the AE and also evaluates the UN. Due to its superficial location, the AE is easily identified by ultrasound (Fig. 11.5). On axial scans, the AE appears as a hypoechoic structure located superficial to the UN in the cubital tunnel. Scanning during progressive elbow flexion may show anterior displacement of the muscle and impingement on the posterior aspect of the UN. Internal UN changes secondary to long-standing friction include swelling, irregular hypoechoicity, and loss of the normal internal fascicular pattern.
Wrist
Anomalous wrist muscles can be true AMs or anomalous insertions of normal muscles. The most frequent anomalous insertion is proximal extension of the lumbrical muscles. The most common AMs are the accessory abductor digiti minimi (AADM), extensor digitorum brevis manus, and the digastric flexor digitorum superficialis muscle of the index finger,27 which can be encountered as incidental findings in asymptomatic subjects or present as clinically evident masses or compression of the median or the ulnar nerves in the carpal tunnel or Guyon canal.
Variations of the palmaris longus muscle belly are quite common.27 Normally, the muscle belly is located proximally and has a very thin tendon that lies in the subcutaneous tissues just superficial to the median nerve and the flexor digitorum tendons, and inserts in the proximal edge of the transverse carpal ligament and the palmar aponeurosis. Occasionally, the muscle belly is in a central or distal position. When located distally, the muscle has a long proximal tendon, giving the appearance of a “reversed” palmaris longus (Fig. 11.6). This variation is mostly asymptomatic and appears as local swelling of the palmar aspect of the distal forearm/wrist, but rarely it has a mass effect on the median nerve and the flexor tendons. Ultrasound easily shows this superficially located anomalous muscle in both axial and sagittal planes.
The AADM is the most common AM of the wrist and is present in approximately 24% of normal individuals.27 It originates from the palmar carpal ligament and palmaris longus tendon, and inserts on the abductor digiti minimi and the medial aspect of the base of the proximal phalanx of the little finger.28 The AADM runs inside Guyon canal, a fibro-osseous canal on the ulnar aspect of the palmar wrist that contains the ulnar artery and nerve (Figs. 11.7, 11.8). When present, the AADM is usually located in the anterior part of the canal, but it can also run between the artery and the UN. Although usually asymptomatic, it may cause compression of the nerve against the pisohamate ligament. In this case, a positive Tinel sign can be elicited by gently tapping over the canal. Muscle size may be an important factor. Patel et al.23 found a difference in the thickness of the AADM muscle between asymptomatic subjects (mean 1.7 mm) and patients (mean 4 mm) with clinical signs of UN entrapment at the wrist. Axial sonograms are best for demonstrating this anomalous muscle inside Guyon canal and show muscle thickness and the shape and size
of the UN. Ultrasound has the advantage over MRI of dynamic examination. Ultrasound performed with active abduction of the little finger can show an increase in the AADM muscle thickness and impingement on the UN. Color and power Doppler allow accurate evaluation of the ulnar artery and its internal flow, thus differentiating nerve compression by the muscle from hypothenar hammer syndrome.
of the UN. Ultrasound has the advantage over MRI of dynamic examination. Ultrasound performed with active abduction of the little finger can show an increase in the AADM muscle thickness and impingement on the UN. Color and power Doppler allow accurate evaluation of the ulnar artery and its internal flow, thus differentiating nerve compression by the muscle from hypothenar hammer syndrome.
The extensor digitorum brevis manus is present in 1% to 3% of individuals.28,29 It arises from the distal radius and the distal radiocarpal ligament and inserts into the index or middle finger. It appears clinically as a fusiform lump alongside the extensor tendon of the index finger and is easily mistaken for a dorsal ganglion or tenosynovitis of the extensor tendons. Active resisted extension of the index or middle finger results in increased firmness of the mass and indicates the correct diagnosis. Radiographs are normal. Ultrasound is able to identify the typical echotexture of the muscle and its relationship with the extensor tendons (Fig. 11.9).
On the ventral wrist, an unusually situated muscle belly of the flexor digitorum superficialis muscle may cause symptoms related to carpal tunnel syndrome. The anomalous muscle belly can be seen on ultrasound entering the carpal tunnel during extension of the fingers (Fig. 11.10). Dynamic scanning during flexion and extension of the fingers shows the muscle entering and exiting the tunnel. An unusual proximal origin of the lumbricals inside the carpal tunnel can also be encountered. These muscles are pulled into the tunnel during flexion of the fingers and may cause median neuropathy. Dynamic scanning with flexion and extension of the fingers is essential for a proper diagnosis of anomalous lumbrical muscles.
Lower Extremity
Thigh-knee
The tensor fasciae suralis (TFS) is a rare anomalous muscle in the popliteal region that presents as a popliteal mass. It arises from the semitendinosus muscle, runs superficial to the medial head of the gastrocnemius
(MHG), and continues as a thin and long tendon that joins the Achilles tendon.30,31 In the diagnostic workup of a popliteal mass, ultrasound is commonly used because it differentiates cystic from solid lesions, assesses the popliteal artery, and demonstrates the relation of a mass to the surrounding tendons and nerves.
(MHG), and continues as a thin and long tendon that joins the Achilles tendon.30,31 In the diagnostic workup of a popliteal mass, ultrasound is commonly used because it differentiates cystic from solid lesions, assesses the popliteal artery, and demonstrates the relation of a mass to the surrounding tendons and nerves.
The actual prevalence of TFS is unknown. Sporadic reports present its ultrasound and MRI appearances.31,32 Both modalities show the typical internal architecture of fibroadipose septa and muscle fibers inserting into a central tendon (circumpennate muscle). Axial images are particularly helpful in demonstrating the location of the aberrant muscle and its relation to other anatomic structures, whereas sagittal images provide better details of its internal architecture. In evaluating a popliteal solid mass, it is important to be aware of the possibility of a TFS to avoid unnecessary surgical exploration.
Ankle
The largest AM at the ankle is the accessory soleus (AS), which is located between the Achilles tendon and the soleus muscle.9,11,12,13,15,16,33,34,35 Usually it originates from the posterior aspect of the tibia and anterior aspect of the soleus muscle. Depending on the insertion, five types of AS have been described. In type 1, the muscle inserts into the Achilles tendon and ends 1 to 2 cm from the calcaneum. In types 2 to 3, the muscle inserts directly (type 2) or through a short tendon (type 3) anterior to the Achilles tendon on the upper aspect of the calcaneum. In types 4 to 5, the AS inserts directly (type 4) or by its tendon (type 5) into the medial face of the calcaneum.
The AS usually presents on clinical examination as an indolent posteromedial mass that has a soft consistency when the patient is examined in the recumbent position, but becomes tense when the patient is examined standing and tiptoeing actively. The AS can be symptomatic and cause local pain due to ischemia related to overuse in sport. A large AS may compress the tibial nerve. Pain can also come from increased pressure inside the fascia, as indicated by symptom relief following fasciotomy.
Several imaging modalities show the AS (Fig. 11.11). Standard radiographs can suggest the diagnosis by showing a fusiform opacity obliterating the normal triangular fatty space of Kager on the lateral view of the ankle, although this is a nonspecific appearance. Ultrasound shows a muscle located anterior to the Achilles tendon, best evaluated by longitudinal images over the Achilles
tendon that show the aberrant muscle replacing the fat. More distal longitudinal and transverse images can evaluate the distal insertion and differentiate the five types of AS. CT has been described as a better modality in the evaluation of larger AS due to its greater field of view compared with ultrasound. The internal density of the AS is similar to normal muscle.35 MRI is the most effective modality because it demonstrates the entire muscle in both sagittal and coronal planes.13,16 As for ultrasound, axial images are best suited to assess its relation to adjacent neurovascular structures. Differentiating AS from a soft tissue tumor is the main goal of imaging.12 For this, ultrasound and MRI are effective because they demonstrate the normal internal muscle structure.
tendon that show the aberrant muscle replacing the fat. More distal longitudinal and transverse images can evaluate the distal insertion and differentiate the five types of AS. CT has been described as a better modality in the evaluation of larger AS due to its greater field of view compared with ultrasound. The internal density of the AS is similar to normal muscle.35 MRI is the most effective modality because it demonstrates the entire muscle in both sagittal and coronal planes.13,16 As for ultrasound, axial images are best suited to assess its relation to adjacent neurovascular structures. Differentiating AS from a soft tissue tumor is the main goal of imaging.12 For this, ultrasound and MRI are effective because they demonstrate the normal internal muscle structure.
Accessory flexor hallucis longus (AFHL) is a rare and small muscle located lateral to the flexor hallucis longus (FHL). It is usually asymptomatic, but it can sometimes cause impingement and tenosynovitis of the FHL. MRI is the best modality for its evaluation since ultrasound does not allow optimal assessment of deep structures.
The prevalence of the AFHL is reported to be 8%.36 The muscle originates from the posterior aspects of the tibia or fibula or from other posterior calf structures, runs inside the tarsal tunnel, and inserts into the quadratus plantae or the flexor digitorum longus. Inside the tarsal tunnel, it is trapped between the FHL and the flexor retinaculum posterior to the neurovascular bundle, and can cause compression of the tibial nerve.20 On imaging, AFHL resembles the AS. However, they are situated in different positions. While AS lies superficial to the flexor retinaculum, the AFHL is located deep to the retinaculum. Knowledge of the existence of this AM is important, and it must be distinguished from other causes of tarsal tunnel syndrome.
Peroneus tertius is a small muscle, often considered to be a part of extensor digitorum longus. It originates on the distal fibula and inserts on the base of the fifth metatarsal.
Peroneus quartus (PQ), the most frequent ankle AM, is found in 22% of subjects.37 Its origin is almost always from the peroneus brevis muscle, while its insertion is highly variable. The most frequent insertions are on the peroneus brevis and longus tendons, the base and head of the fifth metatarsal, and the trochlear process of the calcaneum. The PQ lies posteromedial to the peroneal tendons and has a variable appearance ranging from a large muscle with a very short tendon or, conversely, a short muscle with a long thin tendon. Most patients are asymptomatic, but some present with signs of peroneus brevis tenosynovitis including retromalleolar pain and swelling. Local inflammation can result from attrition between the peroneus brevis, longus, and quartus. This can cause increased pressure on the peroneus brevis tendon and result in partial and then complete longitudinal splits in peroneus brevis.38 The diagnosis of PQ relies on imaging because it cannot be palpated clinically. Ultrasound and MRI can confirm the presence of PQ posteromedial to the peroneal tendons.39,40 Ultrasound may show an effusion inside the tendon sheath or a longitudinal split of peroneus brevis.41 The PQ tendon must be differentiated from a longitudinal split of the peroneus brevis tendon by showing the peroneus quartus tendon arising from its muscle belly on axial ultrasound or MRI. Symptomatic patients are usually treated by surgical excision of PQ,
repair of the peroneus brevis split, and tenosynovectomy. Utilization of the PQ tendon for the reconstruction of the peroneal retinaculum has been proposed in treating peroneal tendon instability (Fig. 11.12).42
repair of the peroneus brevis split, and tenosynovectomy. Utilization of the PQ tendon for the reconstruction of the peroneal retinaculum has been proposed in treating peroneal tendon instability (Fig. 11.12).42
Tip:
Accessory muscles have the shape and internal echotexture of other muscles. They also contract and relax like other muscles.
TRAUMATIC LESIONS
Introduction
Muscle injuries are common in athletes and the general population. Acute injuries include strains and tears, contusions, hematomas, and delayed-onset muscle soreness (DOMS). Muscle strain is the most common pattern of acute injury and involves mainly the lower extremities. The clinical features of muscle injuries typically include sudden onset of pain or tenderness.43 Risk factors for muscle injuries include performing eccentric contractions, muscles that cross more than one joint, and those that contain predominantly type II fibers.44 Previous muscle injury, increasing age, and adjacent joint disease also increase the risk.45
Tip:
Risk factors for muscle strains include eccentric contractions, muscles that cross two joints, and muscles that contain predominantly type II fibers.
Early diagnosis is essential in the treatment of muscle injuries. Poor assessment of the size of the lesion can lead
to inadequate treatment and expose patients to complications such as fibrous scar, myositis ossificans, calcific myonecrosis, and compartment syndrome, which increase disability. The role of imaging is to supplement clinical evaluation by describing the type, exact location, and severity of muscle injury. These data are essential since recovery is related to the size of the muscle injury and its location (e.g., injuries of semimembranosus or the central tendon of rectus femoris have a poorer prognosis). Additionally, avulsions may require surgical repair.46,47
to inadequate treatment and expose patients to complications such as fibrous scar, myositis ossificans, calcific myonecrosis, and compartment syndrome, which increase disability. The role of imaging is to supplement clinical evaluation by describing the type, exact location, and severity of muscle injury. These data are essential since recovery is related to the size of the muscle injury and its location (e.g., injuries of semimembranosus or the central tendon of rectus femoris have a poorer prognosis). Additionally, avulsions may require surgical repair.46,47
MRI and ultrasound are widely used in daily routine to assess muscle injuries. Ultrasound is quicker, more available, cheaper, and better tolerated by patients than MRI. High-quality transducers and recent advances in software technologies provide depiction of subtle findings. Nevertheless, accuracy depends also, and even mainly, on the experience of the sonographer. Deep structures such as pelvitrochanteric muscles or adductor or hamstring origins are more difficult to assess with ultrasound, especially in patients with high body mass index,48 and MRI may be preferable. Comparison of ultrasound and MRI in hamstring injuries shows that sensitivity is equal in acute injuries and suggests that ultrasound should be preferred because of its cheaper cost.49 Ultrasound has higher sensitivity in the detection of recent fluid collections,50 whereas MRI allows better analysis of residual abnormalities and remains the modality of choice for healing control and follow-up.
Delayed-onset Muscle Soreness
DOMS is a benign condition that consists of tenderness or stiffness to palpation and/or movement after strenuous muscle activity. Symptoms typically begin 24 to 48 hours after exercise, peak after 2 or 3 days, and disappear after 7 to 10 days. They are located initially at the myotendinous junction and tend to spread to the entire muscle.51,52 The severity and duration of symptoms depend on the muscle group injured, the level of the athlete, and muscle performance. The pathomechanism of DOMS is still unclear, and various theories (lactic acid accumulation, connective or muscle tissue damage, muscle spasm, local inflammatory changes with enzyme release) have been suggested.53
Magnetic resonance shows increased intensity signal on fluid-sensitive sequences without evidence of bleeding, initially located at the myotendinous junction. The edema can progress to the entire muscle and can then be seen and better appreciated on axial images. In severe injuries, edema can even extend into the subcutaneous tissue. MRI changes occur later than the onset of symptoms, about 3 to 5 days after the precipitating exercise and can be observed for up to 80 days after the onset of symptoms.54,55
Ultrasound is normal or shows ill-defined focal areas of homogeneously increased echogenicity that can spread to the entire muscle.56,57 Transverse sonograms are most helpful in detecting these subtle abnormalities, and comparison with adjacent normal muscles or the opposite limb is frequently necessary to confirm the diagnosis.
Tears
Muscle tears are secondary to two main pathomechanisms: extrinsic tears from direct external trauma and intrinsic tears caused by forced contraction of an elongated muscle.58,59
Extrinsic injuries mainly affect the lower limbs and range from simple disorganization to rupture of muscle fibers and supporting connective tissue associated with a local hematoma. Injuries are more serious if the affected muscle is trapped between the extrinsic compression and the adjacent bone. This explains why in thigh trauma, hamstring injuries are usually less serious than injuries to the quadriceps.58
Intrinsic injuries are the result of simultaneous abnormal stretching and forced contraction of a muscle. Lower limb muscles and muscles that cross two joints (e.g., hamstring muscles, rectus femoris) and/or have complex internal architecture are most frequently affected.
Ultrasound Appearance of Acute Traumatic Lesions and Post-traumatic Lesions
Extrinsic Injuries
Ultrasound examination of extrinsic injuries must first assess possible damage to the subcutaneous tissues such as hematoma, fat fracture, or fascial tear. Ultrasound examination of the fascia in the axial plane is important since fascial injuries can be painful and easily overlooked when there are no associated deep muscle injuries. Small injuries can be more painful and more likely to lead to chronic disability than larger lesions. Next, the ultrasound examination assesses the internal muscle architecture. The degree of muscle damage is usually better assessed on axial sonograms. In minor injuries, ultrasound shows only swelling and disorganization of the muscle architecture with loss of parallelism between muscle fascicles. Moderate tears affect <50% of the muscle on axial images, whereas serious ruptures affect >50% of the muscle. Local hematomas are usually absent in minor injuries. In moderate tears, bleeding is often seen as hemorrhagic infiltration of the muscle without a collection. In serious ruptures, well-defined hematomas are frequently seen, and they can lead to enlargement of the muscle. Recent hematomas appear as echoic collections that change shape during compression with the transducer. A recent hematoma can be drained under ultrasound guidance, usually 10 to 14 days after injury, to lower internal pressure within the muscle compartment, help healing, and possibly reduce the chance of secondary ossification, although evidence for these benefits does not exist. Organized hematomas are unsuitable for needle puncture. They are
more echogenic, show internal clots, and are not affected by local compression (Fig. 11.13).
more echogenic, show internal clots, and are not affected by local compression (Fig. 11.13).
Intrinsic Injuries
Intrinsic injuries are usually located at the myotendinous junction, but they may also be found inside the muscle belly.60,61,62 They can be classified into four grades depending on ultrasound appearances.
Grade 1. Muscle injury without bleeding. These injuries affect only the muscle fibers without tearing the vascularized connective tissue. Therefore, there is no bleeding. Ultrasound shows an ill-defined local hyperechoic area without alteration of the muscle architecture. These subtle findings are more evident on MRI, which shows feathery hyperintense areas on T2-weighted sequences and a normal appearance on T1-weighted images. Pain relief is usually obtained after about a week.
Grade 2. Myoaponeurotic injury without hematoma. In grade 2 injuries, there are ruptures of the junctions between the muscle fascicles and the perimysium. There are also secondary changes in the internal muscle architecture and bleeding but no hematoma. Injuries are located at the insertion of the muscle fibers on the “connective skeleton,” that is, the aponeurosis and tendon. Ultrasound shows an ill-defined heterogeneous area with disorganization of the muscle architecture (Fig. 11.14). MRI demonstrates hyperintense signal on T2- and T1-weighted images. Pain usually resolves over 3 weeks.
Grade 3. Myoaponeurotic injury with hematoma. The hallmark of grade 3 injury is the presence of a local hematoma. The myoaponeurotic tear appears as a hematoma adjacent to an intramuscular septum, which is often difficult to detect, especially at ultrasound. In injuries at an aponeurosis, the hematoma develops along one side of the aponeurotic septum.61,62 This is by far the most common type of injury. Examples include the proximal myotendinous junction of rectus femoris, the distal insertion of the long head of biceps femoris, the MHG (Fig. 11.15), the proximal septum of adductor longus, and the myotendinous junction of semimembranosus.59 About 90%
of such injuries occur in the lower limbs; the other 10% are mainly located on the biceps and brachialis muscles, and the muscles of the abdominal wall. Both ultrasound and MRI show the local hematoma. The ultrasound appearances of hematomas vary over time. Hematomas initially contain hypoechoic fluid, then solidify and become hyperechoic over the next few days, before liquefying again after 3 to 4 days and developing an anechoic center and echogenic periphery. Healing then occurs as centripetal filling-in over weeks or months, depending on the size of the hematoma. Hematomas may gradually enlarge and should be monitored by serial examination in case drainage becomes appropriate. The distinction between hematoma and reactive edema is not always easy, especially with MRI. With this type of injury, sports activity should be stopped for about 4 to 6 weeks.
of such injuries occur in the lower limbs; the other 10% are mainly located on the biceps and brachialis muscles, and the muscles of the abdominal wall. Both ultrasound and MRI show the local hematoma. The ultrasound appearances of hematomas vary over time. Hematomas initially contain hypoechoic fluid, then solidify and become hyperechoic over the next few days, before liquefying again after 3 to 4 days and developing an anechoic center and echogenic periphery. Healing then occurs as centripetal filling-in over weeks or months, depending on the size of the hematoma. Hematomas may gradually enlarge and should be monitored by serial examination in case drainage becomes appropriate. The distinction between hematoma and reactive edema is not always easy, especially with MRI. With this type of injury, sports activity should be stopped for about 4 to 6 weeks.
Grade 4. Muscle tear. Grade 4 injury consists of a complete tear and retraction of the muscle. These injuries are observed mainly at the inferior portion of the rectus femoris or the conjoint tendon of the hamstring muscles but can also affect the long head of biceps femoris and the MHG. Both ultrasound and MRI show a defect in and proximal retraction of the muscle. The proximal stump can be surrounded by local hematoma, resulting in the typical “bell clapper” appearance (Fig. 11.16). Dynamic ultrasound examination during muscle contraction can help in detecting the retraction. Bleeding can extend along fascial planes and into and along the subcutaneous tissues, which explains why bruising is located distal to the site of the muscle injury. This injury usually requires 2 to 3 months of rest from sports activities.
Tip:
Dynamic ultrasound examination during muscle contraction confirms whether a muscle tear is complete and shows the extent of muscle retraction.
The size of the injury and the degree of healing are important elements for deciding when activities can be resumed as recovery time is proportionate to injury severity. Recurrent tears are frequent, especially in serious injuries or in patients with a previous injury.63,64,65,66,67,68,69,70,71,72 Proximal injuries have a poorer prognosis.72,73 To prevent recurrence and guide treatment, relationships between the size of the injury and the duration of rest have been proposed, especially using MRI.73,74,75,76 At ultrasound, the presence of regular contours of the injury and the disappearance of central hyperemia on color Doppler are the two factors that confirm the healing process. Nevertheless, it is important to recognize that ultrasound findings must be correlated with clinical data before physical activity is resumed. Ultrasound can assess different post-traumatic
lesions such as chronic tears, muscle fibrosis, calcification, myositis ossificans, and muscle hernias.77,78
lesions such as chronic tears, muscle fibrosis, calcification, myositis ossificans, and muscle hernias.77,78
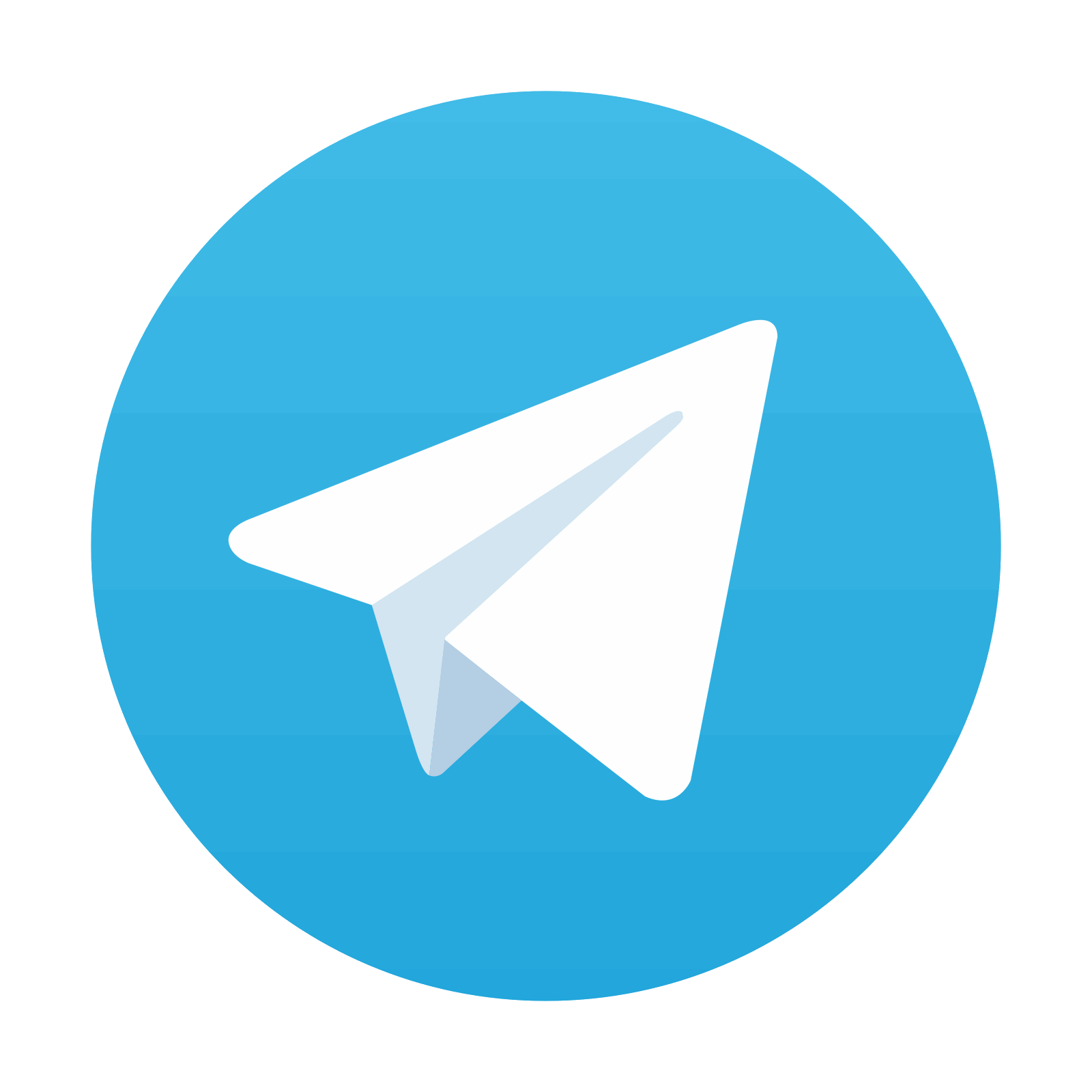
Stay updated, free articles. Join our Telegram channel

Full access? Get Clinical Tree
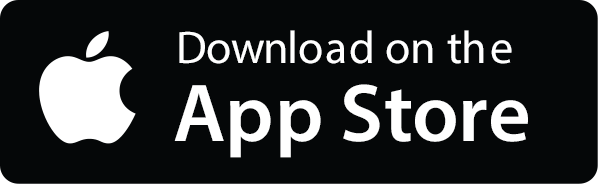
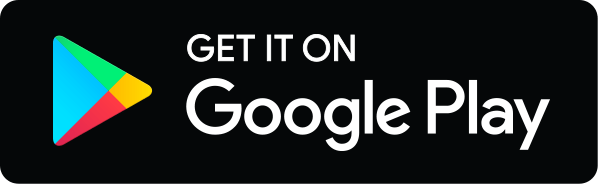
