(1)
Department of Nuclear Medicine, Kuwait University, Safat, Kuwait
5.1.1 Bone Structure
5.1.2 Blood Supply
5.1.3 Bone Remodeling
5.1.4 Bone Marrow
5.2 Bone Diseases
5.2.4 Fibrous Dysplasia
5.2.5 Trauma
5.2.6 Growth-Plate Injury
5.2.7 Metabolic Bone Diseases
5.2.8 Arthropathy
5.3.1 Primary Bone Tumors
5.3.2 Metastatic Bone Disease
Abstract
Bone is a rigid connective tissue which provides support and protection for body organs and tissues. Within certain bones such as the skull, vertebrae, and ribs, marrow cavities serve as sites of blood formation. Bone also has an important function in mineral homeostasis.
5.1 Anatomical and Physiological Considerations
Bone is a rigid connective tissue which provides support and protection for body organs and tissues. Within certain bones such as the skull, vertebrae, and ribs, marrow cavities serve as sites of blood formation. Bone also has an important function in mineral homeostasis.
5.1.1 Bone Structure
Structure of normal adult bone can be summarized in four categories:
A.
Gross Level
Skeleton consists of two major parts, axial skeleton and appendicular skeleton. The axial skeleton includes the skull, spine, and rib cage (ribs and sternum), while the appendicular skeleton involves the bones of the extremities, pelvic girdle, and pectoral girdle (clavicles and scapulae).
B.
Tissue Level
The bone is divided into two types of tissues forming the skeleton: compact (cortical) and spongy (trabecular or cancellous) bone. In mature bone, compact bone forms an outer layer (cortex) which surrounds an inner medulla consisting of loose trabecular spongy bone. The architecture is arranged in the Haversian system. The spongy portion contains hematopoietic cells, which produce blood cells, fat, and blood vessels. The spongy bone has a turnover rate of approximately eight times greater than that of cortical bones and hosts hematopoietic cells and many blood cells. The compact bone constitutes 80 % of the skeletal mass and contains 99 % of the total body calcium and 90 % of its phosphorus.
C.
Cellular Level
Three types of cells are seen in the bone: (1) osteoblasts that produce the organic bone matrix, (2) osteocytes that produce the inorganic matrix, and (3) osteoclasts which are active in bone resorption [1]. Osteoclasts are derived from the hematopoietic system in contrast to the mesenchymal origin of osteoblasts. Osteocytes are derived from osteoblasts that have secreted bone around themselves [2].
D.
Molecular Level
At the molecular level, bone matrix is composed primarily of organic matrix (approximately 35 %) including collagen and glycoproteins and inorganic matrix (approximately 55 %), which includes hydroxyapatite, cations (calcium, magnesium, sodium, potassium, and strontium), and anions (fluoride, phosphorus, and chloride) [3, 4].
Table 5.1 summarizes the major constituents of bone and their function.
Table 5.1
Bone structures and their functions
Major structural elements | Function |
---|---|
Bone cells | |
Osteoblasts | Synthesize collagen and proteoglycans, stimulate osteoclast resorptive activity |
Osteocytes | Maintain bone matrix |
Osteoclasts | Resorb bone, assist with mineral homeostasis |
Bone matrix | |
Organic matrix | |
Collagen fibers | Provide support and tensile strength |
Proteoglycans | Control transport of ionized materials through matrix |
Sialoprotein | Promotes calcification |
Osteocalcin | Inhibits calcium/phosphate precipitation, promotes bone resorption |
Laminin | Stabilizes basement membranes in bone |
Osteonectin | Binds calcium to bones |
Albumin | Transports essential elements to matrix |
Inorganic matrix | |
Calcium | Crystallizes to provide rigidity and compressive strength |
Phosphate | Regulates vitamin D and thereby promotes mineralization |
5.1.2 Blood Supply
The pattern of the skeletal blood supply varies with the age-group. In children epiphyseal, metaphyseal, and diaphyseal vessels are present. In adults all vessels communicate together. Nutrient and periosteal arteries feed a rich network of vessels to supply the cortex and medulla (Fig. 5.1). This vasculature takes the form of interconnecting capillaries, sinusoids, and veins. It is estimated that blood flow to spongy bone containing marrow is 5–13 times higher than in cortical bone [5].
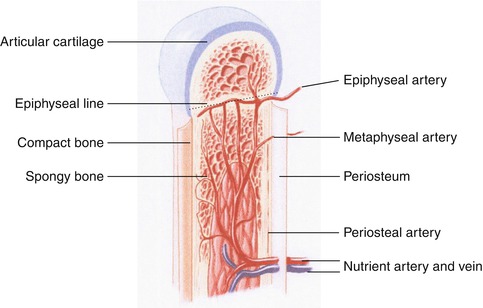
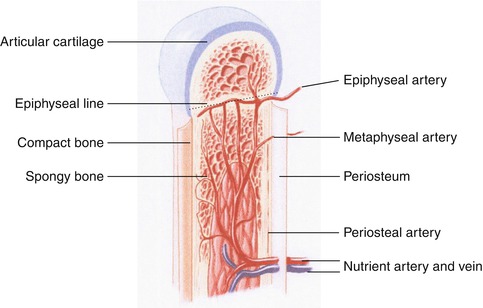
Fig. 5.1
Diagram illustrating blood supply to a long bone
5.1.3 Bone Remodeling
Within all bones a balance between osteogenesis and bone resorption continuously occurs, even in normal nonviolated bone. Remodeling occurs throughout life, with removal and replacement of bone at different rates in different parts of the skeleton. Bone remodeling is regulated by parathyroid hormone, vitamin D, and numerous other factors. It is estimated that 18 % of the skeleton is replaced yearly in adults, indicating that the entire skeleton is replaced every 5 years. The process is more active in spongy bone, with a yearly replacement rate of approximately 25 % compared with 2 % for compact bone [5].
5.1.4 Bone Marrow
Normally, almost the entire fetal marrow space is occupied by red (hematopoietic) marrow at birth. Conversion from red to yellow, nonhematopoietically active marrow starts in the immediate postnatal period. This process begins in the extremities and progresses in general from the peripheral to the central skeleton and from diaphyseal to metaphyseal regions in individual long bones. By approximately the age of 25 years, marrow conversion to the adult pattern is complete (Fig. 5.2). In adults, hematopoietic bone marrow usually is confined to the skull, vertebrae, ribs, sternum, pelvis, and proximal portions of the humerus and femur. Fatty marrow in other bones may contain islands of hematopoietic tissue, however, and for this reason variations on the normal adult pattern of hematopoietic bone marrow are frequently encountered. Acquired alterations in the distribution of hematopoietic bone marrow may be due to surgery, trauma, infection, and other destructive processes. Furthermore, with increasing demand for red cells, reconversion of yellow to red marrow may take place. This process follows the reverse order of the initial red to yellow marrow conversion. Accordingly, it starts in the axial skeleton, followed by the extremities from proximal to distal [6, 7].
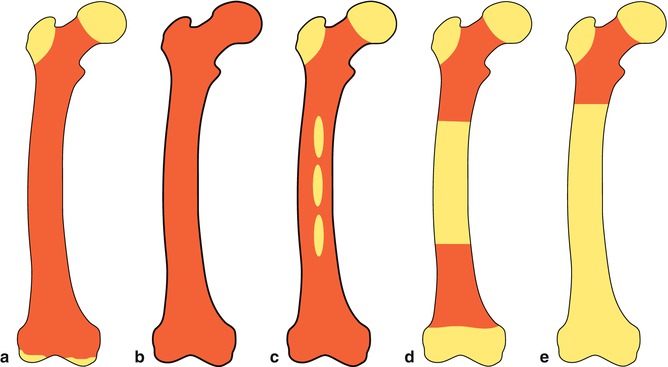
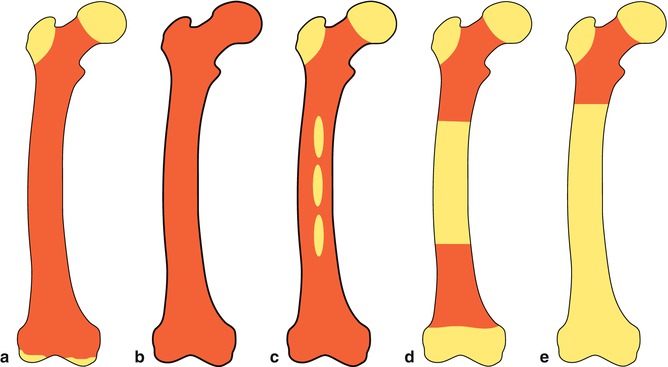
Fig. 5.2
Bone marrow distribution in a long bone illustrating changes during development over the years till the adult pattern is reached by about 25 years of age, (a) birth; (b) 7 years; (c) 14 years; (d) 18 years; (e) 25 years old
5.1.5 Response of Bone to Injury
The principle response of bone to injury and disease is reactive bone formation. This reactive bone goes through stages. It is disorganized early but later may remodel to normal bone. This new disorganized bone is termed woven bone (Fig. 5.3) and is active with no lamellar arrangement.
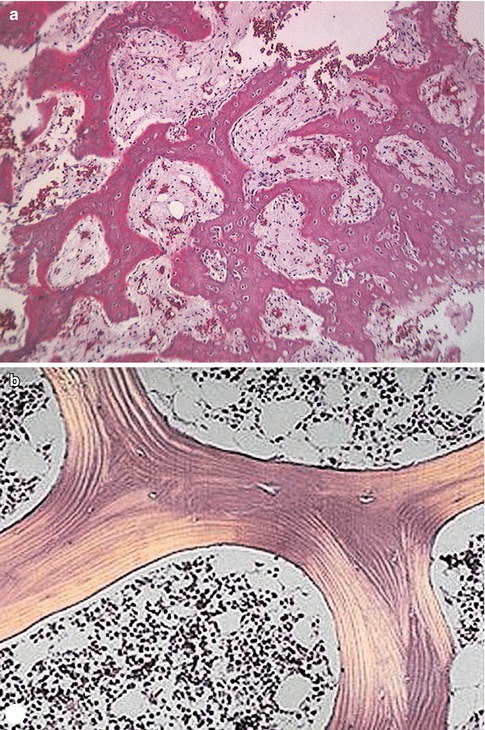
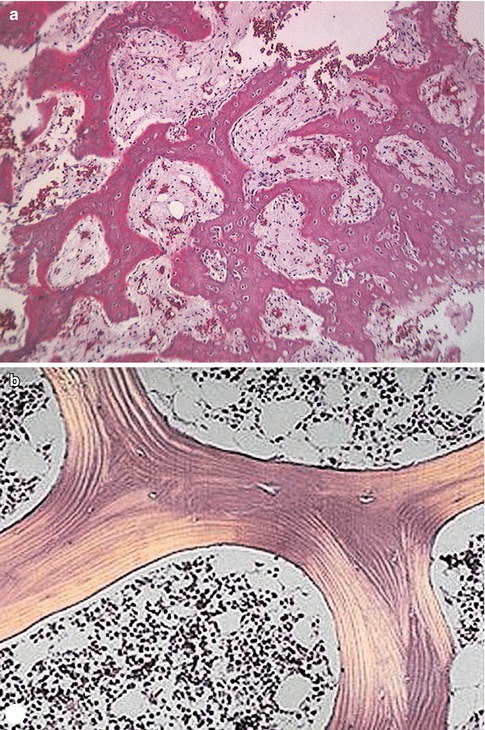
Fig. 5.3
(a, b) Photomicrographs illustrating the difference between woven and lamellar bone. The irregular and disorganized nature of woven bone (a) is easily seen compared to lamellar bone depicted in (b). The bony spicules in lamellar structure are even, with occasional lacunae containing osteocytes. Cellular marrow is seen between the spicules of bone
While several factors affect the uptake of diphosphonates in the skeleton, blood flow and extraction efficiency are the most important. Increased flow of blood produces increased uptake. Pathological foci containing woven bone show increased uptake of 99mTc diphosphonates due to higher extraction [8].
5.2 Bone Diseases
Bone scintigraphy shows many patterns, some specific, in a variety of benign and malignant bone diseases. Many of these patterns are better understood once the underlying pathophysiological changes of different bone diseases are appreciated.
5.2.1 Nonneoplastic Bone Diseases
5.2.1.1 Skeletal Infections
5.2.1.1.1 Definitions
The term osteomyelitis optimally indicates infection involving the cortical bone as well as the marrow. When infection starts in the periosteum, such as in cases of direct extension bone infection, it produces periostitis. At this stage, infection may not yet involve the cortex or marrow and the condition is called infectious periostitis. When infection penetrates the cortex, the term infectious osteitis is used. When marrow is involved as well, the term osteomyelitis is applied (Fig. 5.4).
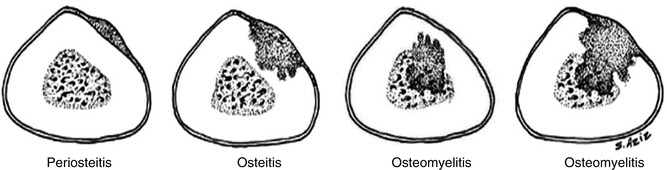
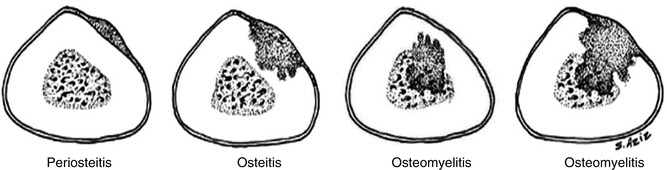
Fig. 5.4
A comparison of the extent of infection in osteomyelitis compared with the extent of infection in periostitis and in osteitis
5.2.1.1.2 Classification
Osteomyelitis may be classified based on several factors [9, 10] including route of infection, patient age, etiology, or onset. In hematogenous osteomyelitis, the metaphyses of long bones are the most common site. Nonhematogenous osteomyelitis occurs as a result of penetrating trauma, spread of a contiguous soft tissue infection, or inoculation (as in drug addicts). In these situations, infection may occur in any part of the bone. Infantile osteomyelitis refers to that occurring prior to 1 year of age; the juvenile type occurs between 1 year and the age at closure of the physes; adult type occurs after closure of the physes. While gram-positive bacteria such as Staphylococcus aureus are the most frequent cause, many different organisms have been encountered in osteomyelitis [11–13].
5.2.1.1.3 Pathophysiological Changes
Acute Hematogenous Osteomyelitis
Acute hematogenous osteomyelitis occurs most commonly in children, affecting males approximately twice as often as females. It has a predilection for the metaphyses of long bones, where blood flow is rich and relatively sluggish and bone is relatively porous in comparison to the diaphysis. The process starts by implantation of organisms in the bone marrow. In children between 1 and approximately 15 years of age, the blood supply to the medullary space of the bone enters through the nutrient artery and then passes through smaller vessels toward the growth plate. Once these vessels reach the metaphyseal side of the growth plates, they turn back upon themselves in loops to empty into large sinusoidal veins, where the blood flow is slower. The epiphyseal plate separating the epiphyseal and metaphyseal blood supplies acts as a barrier to the spread of infection (Fig. 5.5a), making joint involvement less common in this age-group. On the other hand, in infants and adults, the terminal branches of the nutrient artery extend into the epiphysis, as there is no growth-plate barrier. This vascular communication between epiphyses and metaphyses facilitates the spread of infection to adjacent joints (Fig. 5.5b).
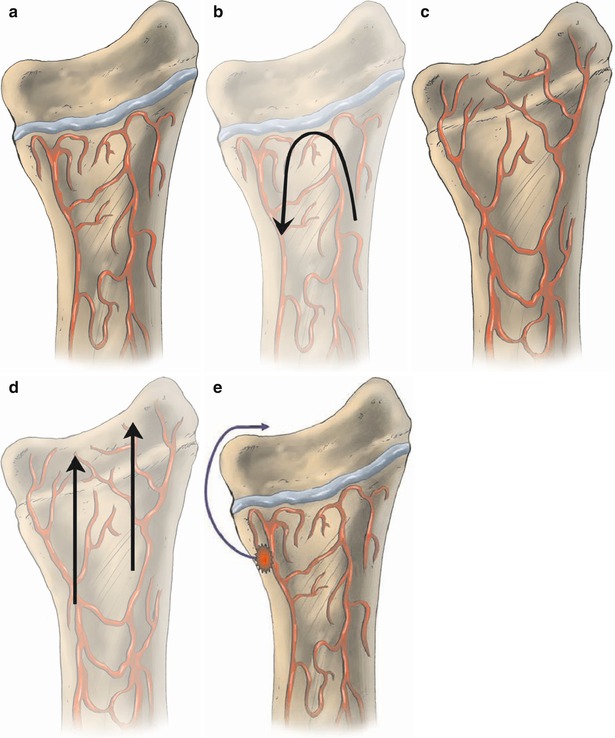
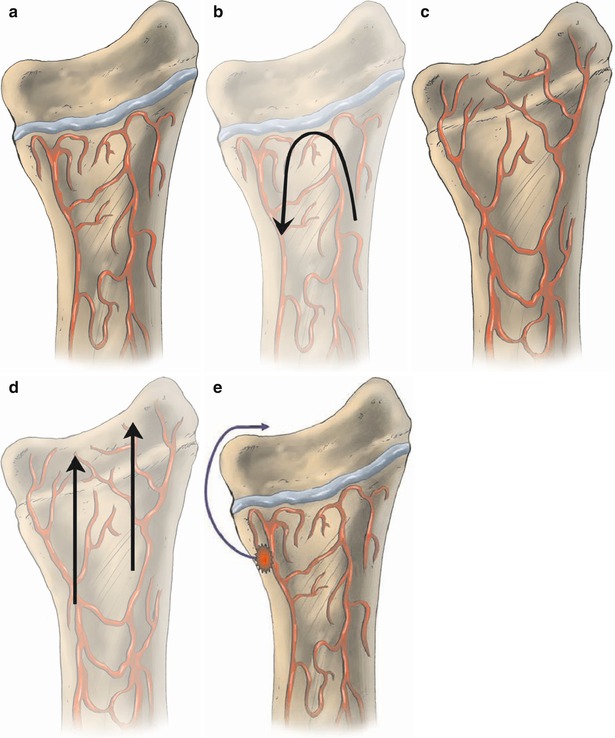
Fig. 5.5
(a–e) Diagram illustrating the vascular communication between the metaphysis and epiphysis of long bones. When the growth plate (a) is present, it acts as a barrier and vessels turn on themselves forming loops. This acts to prevent infection that is most commonly present in the metaphysis from extending to epiphysis and adjacent joint (b). On the other hand, in neonates after the closure of the growth plate (c), infection extends more easily (d) to the joint since there is free vascular communication between metaphysis and epiphysis. (e) illustrates the path of severe infection which is able to involve the joint, when the growth plate is present, by breaking through the bone
When infection lifts the periosteum, the blood supply may be impaired, causing focal necrosis of bone or sequestrum. In some cases, infection may stimulate osteoblastic activity, particularly from the periosteum, osteomyelitis forming new subperiosteal bone that may envelop the infectious focus (involucrum).
Chronic Osteomyelitis
Chronic osteomyelitis has variously been defined as symptomatic osteomyelitis with a duration ranging from 5 days to 5 weeks [14]. Since the pathology of osteomyelitis varies with age, microorganisms, prior therapy, underlying diseases, and other factors, it is somewhat inappropriate to depend only on the duration of the disease to define chronicity. Chronic osteomyelitis has less marked inflammatory cell reactions and may occur without preceding acute inflammation. Microscopically, chronic osteomyelitis shows predominantly lymphocytes and plasma cells rather than polymorphonuclears (Fig. 5.6). There is also fibrosis and a variable amount of necrotic tissue, and sequestra may form in some cases. The presence of necrotic tissue may also lead to draining sinuses or organization in the medullary cavity, forming a cystic cavity (Brodie’s abscess). Because these abscesses are avascular, levels of antibiotics sufficient to eradicate the bacteria may not be achieved during treatment. Accordingly, bacteria may remain indolent for a long time (inactive disease). Reactivation of the disease may occur later, even years after the initial episode (active disease).
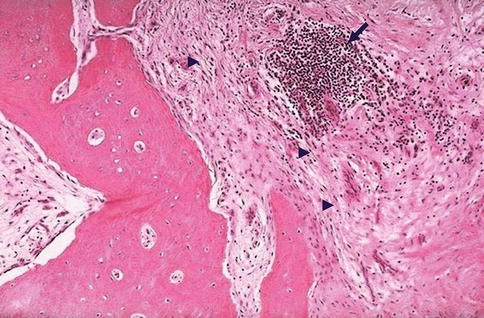
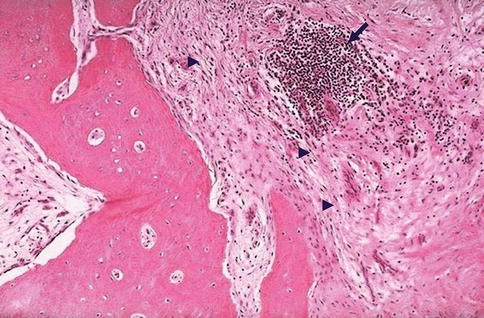
Fig. 5.6
Chronic osteomyelitis. A photomicrograph of a specimen of bone from a patient with long-standing chronic osteomyelitis. Note the presence of numerous lymphocytes (arrow) as well as fibrosis (arrowheads) within the marrow space
Vertebral Osteomyelitis (Spondylodiscitis)
This form of osteomyelitis has some unique features. The disease occurs most frequently in adults with a mean age of 50–70 years, although it also occurs at all other ages, including in children. The most common site is the lumbar region, followed by the thoracic and cervical spine. Infection usually originates at a distant site with hematogenous extension to vertebral bodies and the intervening space via the ascending and descending branches of the posterior spinal artery. Other causes include extension of infection from adjacent structures and complications from spinal surgery and trauma.
In adults, the causative organism generally settles in the richly vascularized subchondral vertebral end plates with eventual progression of infection into the adjacent intervertebral disk, which is relatively avascular. In childhood, infection often starts at the disks, which are nourished by small perforating vessels. In either case, local spread of infection eventually occurs and causes end-plate destruction, disk space narrowing, and collapse. These changes may take weeks to be seen on radiographs [14, 15]. Since the disk is almost invariably involved in vertebral infections, the term spondylodiscitis is preferred [16].
Diabetic Foot Osteomyelitis
This is a common complication of diabetes, particularly when angiopathy is present. It occurs in 15 % of adult diabetic patients and, without prompt diagnosis and treatment, may lead to amputation. Ulceration of the foot is 50 times more common in diabetics [17]. More than 90 % of osteomyelitis of the foot of diabetic patients occurs as a result of the spread of infection from adjacent foot ulcers [18].
Osteomyelitis Associated with Sickle Cell Disease
Erythrocytes become viscous and sickle abruptly when exposed to hypoxia, since hemoglobin S is sensitive to hypoxemia. This may compromise the microvascular flow and may cause infarction, the most common skeletal complication of sickle cell disease. For symptomatic sickle cell patients, distinguishing infarction from osteomyelitis is critical. Although less common than infarctions, osteomyelitis is the second most frequent bacterial infection in children with sickle cell disease after pneumonia.
Periprosthetic Infection
Infection is a clinically important, and increasingly rare, complication after joint replacement. Currently reported incidence of infection after total hip or knee arthroplasties is only 0.5–2 % and is less than 3 % following revision surgery and occurs mostly within 4 months of operation [22]. Infection occurs mostly within 4 months of operation.
The incidence of loosening associated with infection is high and is found in up to 80 % of infected prostheses [23]. Heterotopic bone formation following arthroplasties is not uncommon and is present in about 50–55 % of hip prostheses and 10 % of knee prostheses [24] and may simulate infection clinically.
Infectious (Septic) Arthritis
Infectious (septic) arthritis refers to the invasion of synovial space by microbes. The synovial space contains synovial fluid, which is produced by a rich capillary network of the synovial membrane. This is a viscous fluid that serves to lubricate, nourish, and cushion the avascular joint cartilage. When the synovial space is infected, bacterial hyaluronidase decreases the viscosity of the synovial fluid. Pain is then felt with stress on the joint capsule.
Acute septic arthritis is normally caused by bacteria, while fungal and mycobacterial pathogens are seen more commonly in chronic arthritis. Microorganisms reach the joint by a hematogenous route, contagiously from an adjacent osseous infection, or through traumatic/surgical inoculation. The joints most commonly involved in children are the hip (35 %), knee (35 %), and ankle (10 %). When the synovium becomes hyperemic, flow to adjacent extra-articular bone will also increase via anastomoses from the synovial vascular network to juxtaepiphyseal and epiphyseal vessels supplying the epiphysis and metaphysis. Accordingly, increased uptake of bone-seeking radiopharmaceutical may be seen typically in and around affected joints [25–27].
The acute condition is a medical emergency. Delay in the diagnosis and treatment may result in destruction of the articular cartilage and permanent disability. Additionally, pus in the joint space increases the intracapsular pressure with epiphyseal ischemia. Other sequelae include dislocation, deformity, and destruction of the femoral head and neck. Hence, drainage and antibiotic therapy must be initiated without delay [14, 25].
5.2.1.2 Multimodality Imaging of Skeletal Infections
Several imaging modalities have been utilized for detection of osteomyelitis, including standard radiography, computerized tomography (CT), magnetic resonance imaging (MRI), and nuclear medicine techniques. The choice of modality depends on clinical presentation, duration of symptoms, site of suspected infection, previously known underlying pathology (such as fracture, infection, or tumor), and other factors [14].
5.2.1.2.1 Imaging Acute Osteomyelitis
Standard radiographs are not sensitive for early detection of osteomyelitis, as the changes are evident only after 10–21 days from the time of infection [28]. Bone scintigraphy is very sensitive in the early diagnosis of osteomyelitis [15] and can show the abnormality as early as 24 h after infection [29]. Typically, there is focally increased flow, blood pool activity, and delayed uptake (Fig. 5.8). When the bone has not been previously affected by other pathological conditions (nonviolated), the bone scan has high accuracy and is a cost-effective modality for diagnosis of osteomyelitis with both sensitivity and specificity of 90–95 % [15]. Osteomyelitis may present as cold lesions on bone scan and usually represent an aggressive form [30–32]. Cold foci on bone scan in cases of osteomyelitis are thought to be secondary to increased intraosseous and subperiosteal pressure. Periarticular distribution of the abnormal uptake that is largely limited to the joint capsule and has a uniform pattern indicates septic arthritis. Osteomyelitis, on the other hand, shows abnormal uptake beyond the confines of the joint capsule or shows nonuniform uptake within the joint capsule [33, 34].
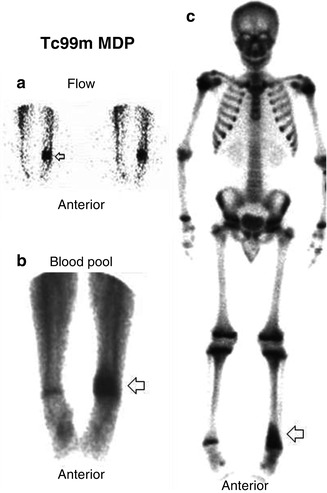
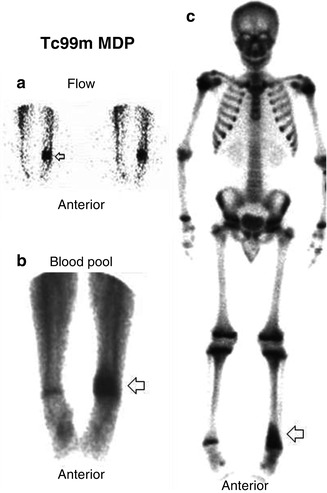
Fig. 5.8
(a–c) A case of osteomyelitis in a nonviolated bone as seen on 99mTc multiphase bone scan. Regionally increased flow (a), blood pool activity (b), and delayed uptake (c) are noted in the left distal femur
If the bone has been affected by a previous pathology (violated), particularly after orthopedic surgical procedures, the bone scan will still be highly sensitive but with poor specificity [15]. In such situations, unless the bone scan is unequivocally negative, an additional modality should be used, particularly scanning with leukocytes labeled with 111In-oxine or 99mTc-hexamethylpropylene amine oxime (HMPAO). Overall, 111In-leukocyte studies have a sensitivity of approximately 88 % and a specificity of 84 % for osteomyelitis [15]. This modality is particularly useful for excluding infection in previously violated bone sites such as in postsurgical and post-traumatic condition. Combined labeled leukocytes and bone scans have a better accuracy than labeled-leukocyte scans alone and can help to localize abnormal foci [35–37]. Best results for detecting osteomyelitis were observed when using combined 111In-WBC and 99mTc-MDP SPECT with SPECT/CT with sensitivity in the range of 84–97 % and specificity 98–100 % [38].
Since labeled-leukocyte scans show uptake by active bone marrow, it may be difficult to differentiate this physiological marrow uptake from abnormal uptake due to infection. Bone marrow scans using 99mTc-sulfur colloid or nanocolloid help in differentiation and improve the specificity [39]. SPECT/CT has proven to improve the accuracy of these imaging procedures and enhances interobserver agreement. Labeled antibodies have also been used. 111In- or 99mTc-labeled human nonspecific polyclonal antibodies (IgG) and several monoclonal antibodies such as labeled antigranulocyte antibodies, anti-NCA-90 (LeukoScan), and anti-NCA-95(fanolesomab) are used to diagnose skeletal infections. Early studies suggested similar or better accuracy (90 %) to WBC scan [40]. However, recent studies showed variable results and suggest that LeukoScan does not achieve the level of accuracy to replace WBC imaging for orthopedic infection.
5.2.1.2.2 Imaging Other Forms of Osteomyelitis
Since bone scan is nonspecific and cannot differentiate neuroarthropathy from osteomyelitis in diabetic patients and cannot differentiate healing from infection in post-traumatic conditions [41], labeled leukocytes are used in combination with bone scan (Fig. 5.9) sequentially or simultaneously although it can be used alone using SPECT/CT (Fig. 5.10). False-positive studies are not uncommon because of the presence of the bone marrow due to reconversion. Accordingly when labeled-leukocyte scan is positive, a bone marrow scan is used to differentiate physiological activity from accumulation due to infection. FDG PET/CT has also been used for the diagnosis of diabetic foot osteomyelitis. Several studies concerning FDG PET imaging in diabetic patients with osteomyelitis provide conflicting results. These differences can be explained by small numbers of included patients and heterogeneity in inclusion criteria.
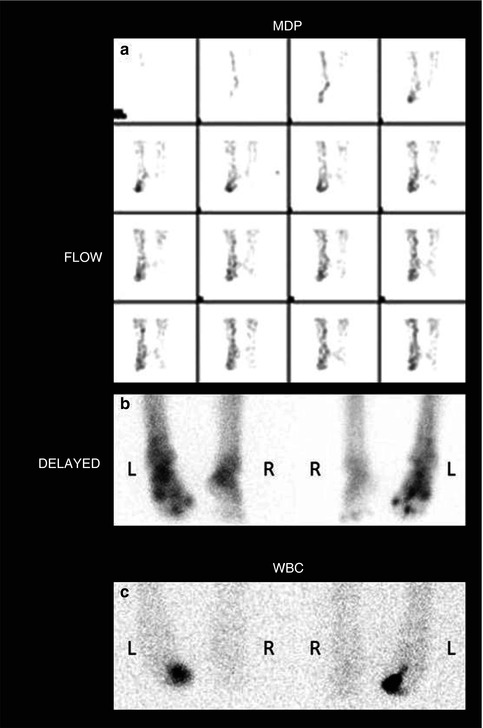
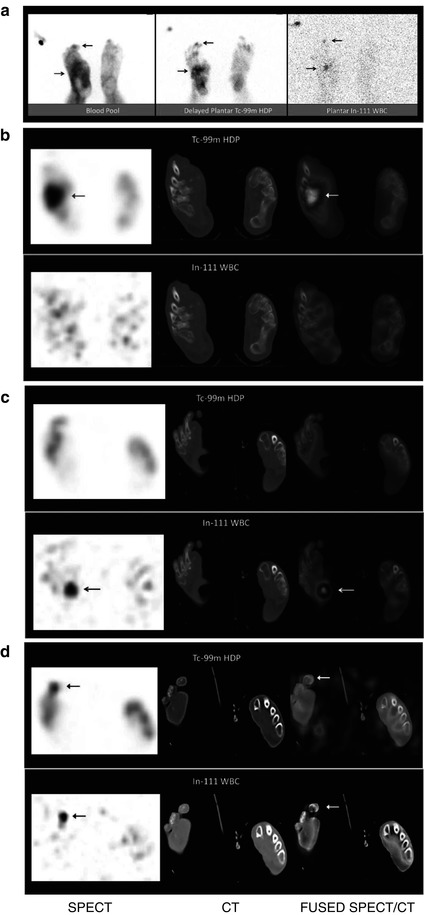
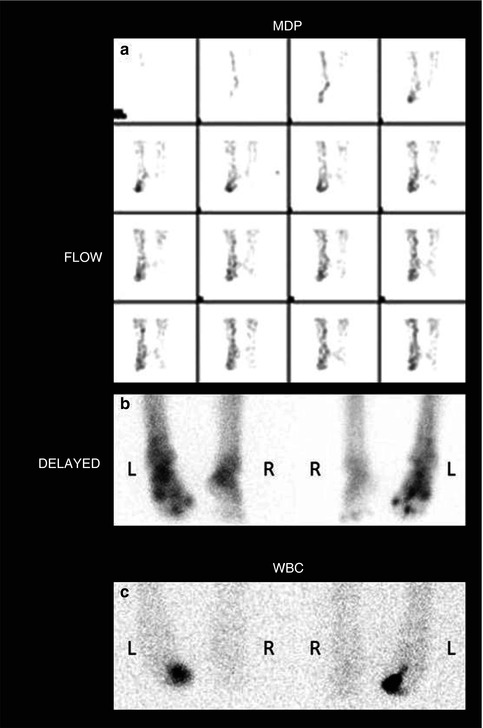
Fig. 5.9
(a) Combined bone and labeled white blood cells for a 50-year-old patient with a long history of diabetes. 99mTc-MDP bone scan shows increased flow to the right foot (a) and multiple areas of increased delayed uptake in the right foot including joints and the right middle toe (b). 111In-labeled white blood cell study shows focal accumulation in the right middle toe (c) in the area of proven osteomyelitis
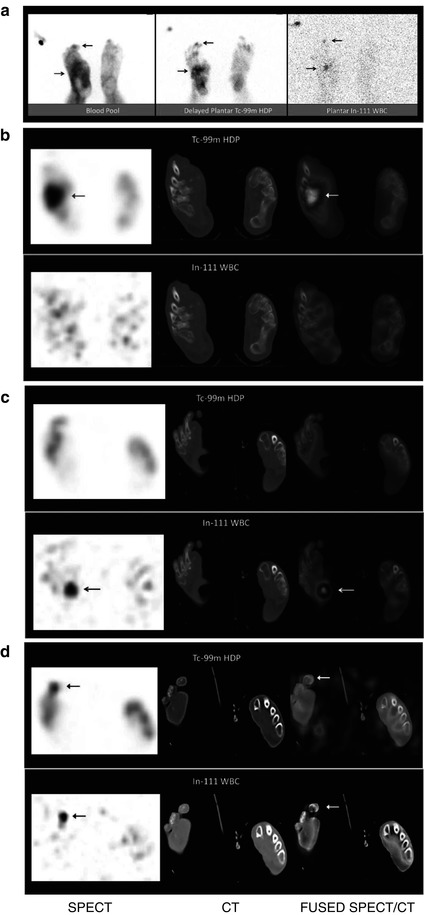
Fig. 5.10
A 59-year-old male diabetic patient S/P right great toe amputation presented with discharging ulcers on the right foot plantar surface, R/O osteomyelitis. (a) The blood pool image demonstrates foci of increased blood pool activity involving the probable distal 3rd toe and mid right foot. The delayed bone scan plantar image demonstrates foci of increased radiotracer uptake in the same regions. On the 111In-WBC plantar image, there are two foci of abnormal uptake also probably in the same areas (arrows). (b) In the selected dual-isotope SPECT/CT transaxial slices, there is increased 99mTc-HDP uptake in the right intermediate and lateral cuneiforms and intercuneiform joint without corresponding abnormality on the simultaneously obtained 111In-WBC images (arrows). These findings are consistent with arthritic changes. (c) In the adjacent dual-isotope SPECT/CT transaxial slices, there is increased 111In-WBC uptake in a region of plantar ulcer without corresponding abnormal uptake on 99mTc-HDP bone scan consistent with soft tissue infection (arrows). (d) In another dual-isotope SPECT/CT transaxial slices, there is focal increased uptake in the right 3rd distal phalanx on both bone scan and In-WBC scan images consistent with a small focus of osteomyelitis (arrows). Based on these images, the patient was effectively treated with soft tissue debridement of the large plantar ulcer and distal right 3rd toe partial amputation as well as antibiotics and was saved from a major foot amputation (Courtesy of Dr. S. Heiba with thanks)
The same combined approach using bone scan, labeled leukocytes, and bone marrow scan, if leukocyte scan is positive, is used for periprosthetic infection. Combined 111In-WBC and 99mTc-sulfur colloid SPECT/CT are adequate tools to diagnose (prosthetic) bone and joint infections. With a sensitivity of 100 %, specificity of 91 %, and accuracy of 95 %, it seems to be significantly better than FDG PET. 99mTc-WBC is a very sensitive tool (95 %) for imaging of infection in patients with metallic implants. Specificity is also high (93–100 %) with SPECT/CT, but it seems dramatically lower (53 %) in the case of 99mTc-WBC SPECT alone. The improvement of specificity by addition of CT to SPECT is of substantial importance, as has been shown in multiple studies. For patients with metallic implants, FDG PET has a good sensitivity (91–100 %) for diagnosis of infection. Specificity, however, is strongly dependent on the used criteria to report infection based on both localization and intensity of FDG uptake, ranging from 9 to 97 %. Specificity is generally higher in hip prostheses, compared with knee prostheses. An adequate criterion seems to consider uptake at the bone—prosthesis interface (with exclusion of the head and the tip) as positive for infection, with 92 % sensitivity and 97 % specificity. This criterion remains to be validated in a prospective study design as another study failed to reproduce this observation. For both SPECT and PET, specificity improves considerably when the scintigraphic images are fused with CT. For SPECT, this holds true for combining 111In-WBC or 99mTc-WBC with 99mTc-MDP or 99mTc-sulfur colloid. Adding CT also enhances interobserver agreement [38, 42]. 99mTc-labeled antigranulocyte antibodies (99mTc-anti-NCA-95 IgG) showed excellent sensitivity for detection of relapsing post-traumatic osteomyelitis (100 %) with good specificity (89 %) using hybrid SPECT/CT, with 100 % interobserver agreement.
For vertebral osteomyelitis, combined gallium and bone scans are used (Figs. 5.11 and 5.12). Labeled-leukocyte study is not accurate for diagnosing this condition. PET/CT and MRI are also used. Again, the same concept is used for the diagnosis of chronic active osteomyelitis with combined bone and gallium study. The detection of (chronic) osteomyelitis with FDG PET is feasible and adequate, with high sensitivity and specificity as it can differentiate bone healing from active infection [43]. FDG PET/CT is useful for the diagnosis and monitoring of vertebral osteomyelitis [41, 44]. For suspected chronic osteomyelitis, SPECT/CT using 99mTc-MDP bone and gallium-67 has proved useful in detection and localization of active disease [45].
For suspected osteomyelitis in sickle cell disease patients, combined bone scan and bone marrow scan is used since bone marrow activity is absent in cases of osteonecrosis but not in osteomyelitis. Occasionally gallium-67 may be used in nonconcussive cases with cold bone scan pattern. Figure 5.11 represents an algorithm for imaging diagnosis of skeletal infections and (Table 5.2) summarizes the basis of scintigraphic patterns.
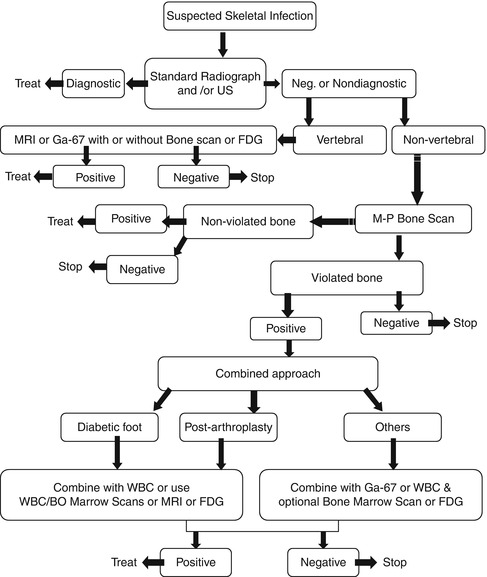
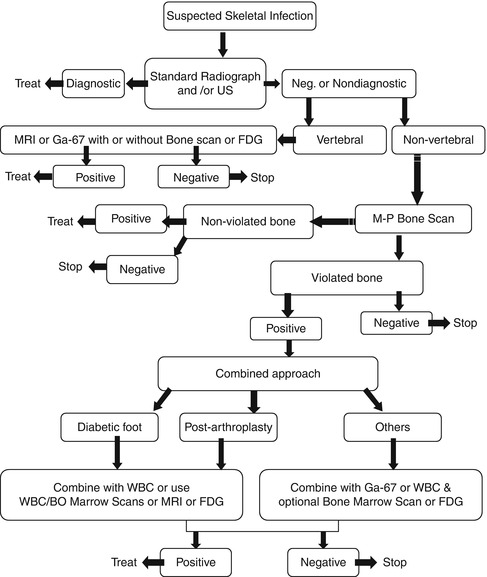
Fig. 5.11
Algorithm for the diagnosis of skeletal infection utilizing multiple modalities based on the location and probable pathophysiology of the suspected infection
Table 5.2
Correlation of imaging findings and pathophysiological features of infection
Vasodilation of blood vessels | Increased flow and blood pool activity on bone scan, increased 67Ga- and 99mTc-nanocolloid accumulation |
Pathological change at the site of infection | Imaging pattern |
Increased permeability and chemotaxis | Increased accumulation of 111In- or 99mTc-labeled WBC |
Increased secretion of iron-containing globulin by injured and stimulated WBC | Increased accumulation of Ga-67 |
Formation of woven bone | Increased uptake of 99mTc-MDP on delayed images with persistent accumulation beyond 3–4 h |
Increased expression of glucose transporters | Increased accumulation of 18F-FDG on activated inflammatory cells |
5.2.2 Avascular Necrosis (Osteonecrosis)
Avascular necrosis of bone results from imbalances between the demand and supply of oxygen to osseous tissues. There are many causes for osteonecrosis (Table 5.3). In some cases the underlying cause cannot be determined, and in this situation, the term primary, idiopathic, or spontaneous osteonecrosis is used.
Table 5.3
Causes of oseonecrosis
Trauma (e.g., fracture or dislocation) |
Hemoglobinopathies (e.g., sickle cell anemia) |
Exogenous or endogenous hypercortisolism (e.g., corticosteroid medication, Cushing’s syndrome) |
Renal transplantation |
Alcoholism |
Pancreatitis |
Dysbaric (e.g., Caisson disease) |
Small vessel disease (e.g., collagen vascular disorders) |
Gaucher’s disease |
Hyperuricemia |
Irradiation |
Synovitis with elevation of intra-articular pressure (infection, hemophilia) |
Idiopathic (spontaneous osteonecrosis) |
Following the interruption of blood flow, blood-forming and mesenchymal cells of the marrow as well as primitive osteoblasts are involved first and die 5–12 h after the interruption of the blood supply. Bone cells including osteocytes and mature osteoblasts die 12–48 h later, followed by the fat cells, which are most resistant to ischemia and die 2–5 days after the interruption of blood flow. This sequence of events may explain why bone marrow scintigraphic changes of decreased uptake appear earlier than bone scan abnormalities, since the bone marrow is affected earlier than the bone cells, which are relatively more resistant to ischemia [46].
The reparative process is initiated and carried out by neovascularization through the collateral circulation, advancing from the periphery of the area of necrosis or by recanalization of occluded vessels. This granulation tissue provides all the elements necessary for the formation of bone matrix and new bone deposition by young osteoblasts. This repair process may be altered. Often bone collapse results from structural weakening and external stress. Bone collapse and cartilage damage can result in significant deformity.
The different scintigraphic patterns of femoral head avascular necrosis are correlated with the sequence of pathological events. In stage I, during the first 48 h, the morphology of bone is preserved and the radiographs are normal. Osteoblastic uptake on bone scan varies from being absent to almost normal. This reflects the greater resistance of mature osteoblasts to ischemia. Subsequently, a cold area of necrosis develops on bone scintigraphy. This avascular pattern will be seen immediately if interruption of the blood supply is abrupt and severe.
Stage II begins with the reparative process. Hyperemia is frequent and there is diffuse demineralization of the area surrounding the necrotic tissue. This stage is characterized scintigraphically by increased technetium diphosphonate uptake starting at the boundaries between the site of necrosis and the normal tissue beginning in 1–3 weeks. This increased uptake eventually will advance around a central photopenic area, lasting for several months.
As the reparative process is completed, uptake returns to normal. However, in cases with bone collapse (stage III), increased uptake may persist indefinitely. Stage IV is characterized by collapse of articular cartilage with degenerative changes on both sides of the joint and resultant increased periarticular uptake.
When osteonecrosis occurs in growing skeleton, it is included in the group of disorders collectively called osteochondrosis. Osteochondrosis involves the epiphyses or apophyses of the growing bones. The process is due to osteonecrosis in some cases, to trauma or stress in others (Table 5.4). In addition to avascular necrosis, the osteochondrosis often demonstrate similar pathological features such as transchondral fractures, reactive synovitis, and cyst formation.
Table 5.4
Osteochondroses
Involved bone | Disease |
---|---|
Capital femoral epiphysis | Legg-Calvé-Perthes disease |
Metatarsal head | Freiberg’s disease |
Carpal lunate | Kienböck’s disease |
Tarsal navicular | Köhler’s disease |
Capitellum of humerus | Panner’s disease |
Phalanges of the hand | Thiemann’s disease |
Tibial tuberosity | Osgood-Schlatter disease |
Proximal tibial epiphysis | Blount disease |
Vertebral body | Scheuermann’s disease |
Patella | Sinding-Larsen-Johansson |
Calcaneus | Serress disease |
Ischiopubic synchondrosis | Van Neck’s disease |
5.2.2.1 Post-traumatic Osteonecrosis
Following a fracture, bone death of variable extent on either side of the fracture line is relatively common. Necrosis of a relatively large segment of bone following fracture or dislocation, however, is generally restricted to sites that possess a vulnerable blood supply with few arterial anastomoses. These sites include femoral head, the body of the talus-scaphoid bone, the humeral head, the carpal hamate and lunate, and the tarsal navicular bone [47].
5.2.2.2 Legg-Calvé-Perthes Disease
This condition represents osteonecrosis of the femoral head in pediatric populations, especially boys 4–7 years old. The blood supply to the adult femoral heads is via the circumflex femoral branches of the profunda femoris artery. This adult pattern of femoral head vascularity usually becomes established after closure of the growth plate. In infancy and childhood, variable vascular patterns can be noted. The changing pattern of femoral head vascular supply with age may explain the prevalence of Legg-Calvé-Perthes disease in persons between the age of 4 and 7 years and the high frequency of necrosis following femoral neck injury in children.
Bone scintigraphy is an integral part of the workup of patients suspected of having the condition. Pinhole imaging must be used routinely in patients with suspected Legg-Perthes disease rather than parallel hole. Pinhole imaging is preferred to SPECT in the diagnosis of this condition in children. Bone scintigraphy is a sensitive as well as specific modality for the diagnosis of this condition showing typically a cold area with or without a rim of increased uptake (Fig. 5.14).
Bone scintigraphy has proven also to have prognostic value. Conway introduced a prognostic classification [49] of two pathways; pathway A is defined by the early appearance of a lateral column formation (before any radiologic sign) in the capital femoral epiphysis indicating early and rapid revascularization. This pathway is associated with good outcome. Pathway B is defined by centrally extended scintigraphic activity from the base of the capital femoral epiphysis or by the absence of the activity in the epiphysis (lateral column formation) after 5 months.
5.2.2.3 Dysbaric Osteonecrosis
This type of osteonecrosis occurs in patients subjected to a high-pressure environment, such as deep-sea divers. The exact cause of ischemia is debated. Immobilization of gas bubbles blocking the vascular channels is considered to be the major factor by many investigators. The presence of intravascular gas bubbles is seen even after ultrasound and other techniques [49] have documented asymptomatic decompression. Shoulders, hips, knees, and ankles are commonly involved in this type.
5.2.2.4 Sickle Cell Disease Necrosis
Sickle cell disease is a relatively common hereditary hematological disorder. The disease is caused by the replacement of glutamic acid of B-chains with valine. The disease has numerous consequences. Osteonecrosis and osteomyelitis are the most common complications [50]. Since sickle cell osteonecrosis most commonly involves the femoral and humeral heads although it can affect any bone of the skeleton, it is possible that the increased length of the nutrient arteries supplying the marrow in the long bones makes them more susceptible to occlusion. Neonates who have sickle cell disease do not often develop osteonecrosis because of the high fetal hemoglobin level. Although the pathogenesis of the vascular occlusion leading to an infarct is not entirely clear, vaso-occlusion of the marrow is considered to be one of the main culprits in sickle cell crisis. Since hemoglobin S is sensitive to hypoxemia, erythrocytes become viscous and sickle abruptly when exposed to hypoxia. This may compromise the microvascular flow and may cause infarction [14]. Signs of acute infarction are nonspecific and may also occur in acute osteomyelitis which may occur as a primary event or may be superimposed on infarcts; necrotic bone is a fertile site for such secondary infections [51]. Thus, recognition of bone marrow infarction often relies on the use of imaging modalities. MRI has not been found to have the specificity or sensitivity of radionuclide studies [52]. The scintigraphic diagnosis may be straightforward using 99mTc-MDP scan which shows photon-deficient areas early on. SPECT and pinhole are very valuable particularly in resolving photon-deficient areas in the middle of the increased uptake at the reparative process. In this stage it can be difficult to differentiate osteonecrosis from osteomyelitis, and adding bone marrow scanning may be essential.
5.2.2.5 Idiopathic (Primary or Spontaneous) Osteonecrosis
This is a unique entity with cases presenting no clear underlying disorders. Most commonly it affects the femoral head; it is usually bilateral and leads to secondary osteoarthritis. It also affects the femoral condyles, tibial plateau, wrists, and humeral heads.
5.2.2.6 Spontaneous Osteonecrosis of the Femoral Head
Primary osteonecrosis of the femoral head affects adult men more frequently than women and is usually seen between the fourth and seventh decade of life. Unilateral and bilateral involvement may be detected. The reported incidence of bilateral disease has varied from 35 to 70 %. Despite the high frequency of bilateral involvement, the condition usually first manifests as unilateral. To demonstrate photopenia in the femoral heads, SPECT is more sensitive (85 %) than planar imaging (55 %) [53].
5.2.2.7 Spontaneous Osteonecrosis of the Knee
This entity occurs most characteristically in the medial femoral condyle. It can also affect the medial portion of the tibial plateau, the lateral femoral condyle, or the lateral portion of the tibial plateau alone or in combination with the medial femoral condyle. It characteristically affects older women and is characterized by abrupt onset of knee pain. X-rays are usually normal at the time of presentation and may even remain so for the entire course of the disease. Scintigraphy is a more sensitive modality and will be helpful in early detection. Scintigraphy may reflect the likely pathogenesis of microfractures with vascular disruption. In the first 5 months, there is increased flow, blood pool activity, and uptake on delayed images. From 5 months to approximately 2 years, blood flow decreases as well as the blood pool activity, while delayed uptake may persist. After 2 years, the bone scan tends to return to normal except in patients who develop joint collapse and secondary osteoarthritis [53]. Osteochondritis dissecans (which affects young patients and does not classically involve the weight-bearing surface of the femoral condyle) should not be confused with spontaneous osteonecrosis. Also osteoarthritis, commonly affecting the knee, is usually limited to the subchondral bone, whereas osteonecrosis tends to involve the adjacent shaft.
5.2.3 Complex Regional Pain Syndrome-1 (CRPS-1)
Complex regional pain syndrome-1(CRPS I) or reflex sympathetic dystrophy is a clinical syndrome which has been defined according to the criteria of the International Association for the Study of Pain (IASP) characterized by pain, allodynia, hyperalgesia, edema, abnormal vasomotor and pseudomotor activity, movement disorder, joint stiffness, regional osteopenia, and dystrophic soft tissue changes [54]. The pathophysiology is not well understood. It is believed that an imbalance between the sympathetic and neuroceptive sensory systems occurs after an event usually trauma. The sympathetic fibers control the tone of distal arterioles and capillaries. It is now believed that the pathophysiology of this syndrome is, at least in part, a disease of both the central and peripheral nervous system [54].
A unifying pathophysiological mechanism in RSD can be proposed, related to an initial triggering injury causing an imbalance between the nociceptors and the autonomic nervous system (sympathetic and parasympathetic) to the affected area. As a result, vasomotor disturbances take place with vasodilation as a prominent feature, leading to increased blood flow to the synovial and osseous tissues. The synovium reacts with cell proliferation and eventually secondary fibrosis. There is a lack of inflammatory cellular infiltration. The adjacent bone undergoes increased turnover locally, with some resorption. Synovial histopathological changes have been found in patients with this condition. The most common changes are proliferation of synovial cells, subsynovial fibrosis, and vascular proliferation. This explains the presence of radiographic and bone scintigraphic changes typical of RSD; vascular changes can be demonstrated on 99mTc-diphosphonate blood pool images, which show increased periarticular activity. The clinical course of the condition, which may be under-recognized and could vary with the location, consists of three stages: acute, dystrophic, and atrophic [55].
Stage 1 is characterized by pain, stiffness, tenderness, and swelling of the involved joint. In stage 2, there is still pain, tenderness, and wasting of subcutaneous tissues and muscles. Thickened fascia and loss of color with cold skin are also seen. Stage 3 may last for months or becomes chronic. This stage is characterized by pronounced wasting of the muscles and subcutaneous tissue. The skin is atrophic and smooth-appearing contractures are frequent.
The scintigraphic pattern depends on the duration or stage of the disease [27]. In the first or acute stage (20 weeks), all three phases of bone scan show increased activity (Fig. 5.12). After 20 and up to 50 weeks during the dystrophic phase, the first two phases are normalized, while the delayed-phase images show increased periarticular uptake. After 50 weeks (atrophic phase), the flow and blood pool images show decreased perfusion, with normal uptake on delayed images. In children with RSD, decreased perfusion and uptake are the most common manifestations (Table 5.5). A unilateral decrease in the metaphyseal band of activity may be the most striking feature. Bone scintigraphy can be used not only to help in the diagnosis but also to monitor the disease with treatment.
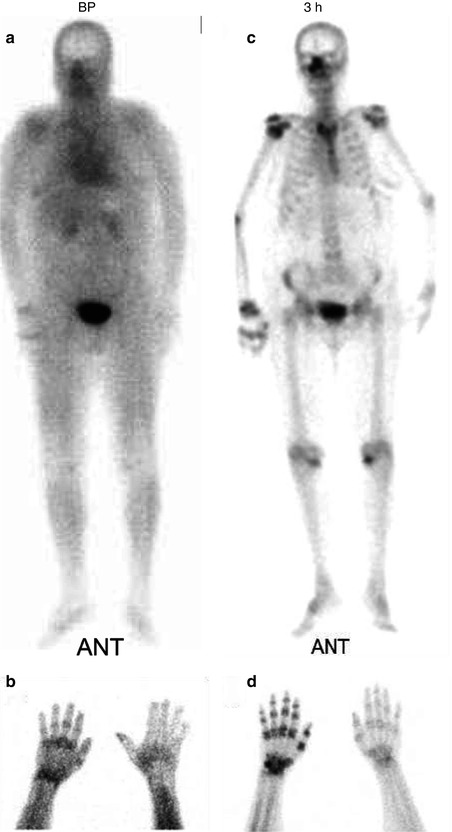
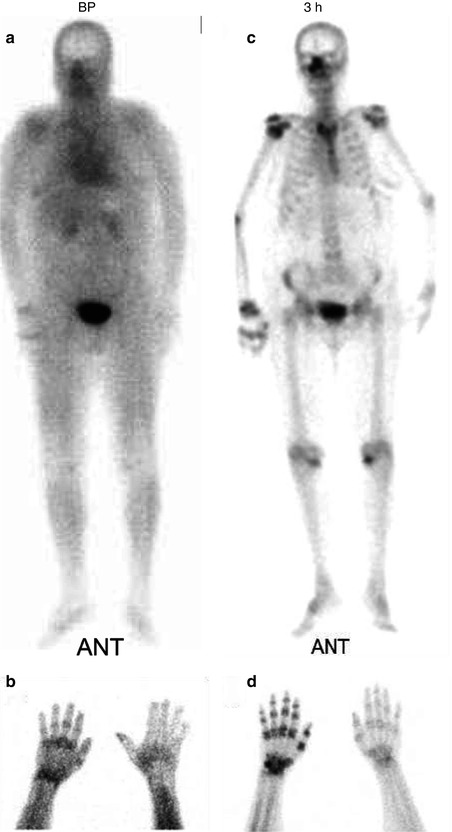
Fig. 5.12
(a–d) 99mTc-MDP whole-body and spot images of a 40-year-old male with CRPS-1 (RSD) involving the right upper extremity. Whole-body blood pool images (a) and blood pool spot image of the hands (b) show increased activity in the right shoulder wrist and hand. Delayed whole-body and spot images (c, d) show periarticular increased uptake in the right shoulder, elbow, wrist, and hand
Table 5.5
Scintigraphic patterns of CRPS-1
Pattern on bone scans | Flow on angiogram | Blood pool | Uptake in delayed images |
---|---|---|---|
Typical | Increased | Increased | Increased |
Atypical | |||
In children and adolescents | Decreased | Decreased | Increased |
Paralysis, immobilization | Decreased | Decreased | Increased |
Subacute | Normal | Normal | Increased |
Late phase of CRPS-1 | Normal, decreased | Normal, decreased | Variable |
Persistent use of painful limb | Decreased | Decreased | Decreased |
5.2.4 Fibrous Dysplasia
Fibrous dysplasia is a benign, developmental, noninherited condition. The etiology is not known. The condition may involve one bone (monostotic) or many bones (polyostotic) and results in the enlargement and deformity of the involved bone. Pathologically it is characterized by slow, progressive replacement of the medullary cavity of the bone by fibrocollagenous tissue containing poorly formed and randomly arranged trabeculae of woven bone, islands of cartilage, and cystic formations of varying size. Elevated serum alkaline phosphatase levels have been observed in about one third of patients, usually with the polyostotic form.
The lesions are monostotic in 70–80 % of patients and polyostotic in up to 30 % of cases. Multiphase bone scans show increased blood flow and blood pool activity and increased uptake of bone-imaging agents [27].
The uptake is usually intense, reflecting hyperemia as well as osteoid matrix. However, not every case has intense uptake since rarely it shows barely increased uptake probably due to concurrent bone infarct [56]. F-18 FDG is usually negative except in case reports. Variability of FDG uptake may be due to differing number of actively proliferating fibroblasts. The characteristic acceleration of bone turnover is not associated with increased glucose metabolism [57]. A study using 11C-methionine PET in two cases of fibrous dysplasia indicated the presence of viable tumorlike cells [58].
5.2.5 Trauma
Trauma to the musculoskeletal system may affect bone, cartilage, muscles, and joints. To each of these structures, trauma may cause immediate damage and late changes.
5.2.5.1 Fractures
A fracture is defined as a break in the continuity of a bone. Fractures can be classified according to several features. Based on the extent of the break, fractures are classified as complete or incomplete. A complete fracture breaks the bone all the way through, while with incomplete fracture the bone is broken but stays as one piece. Fractures are also classified as open (previously called compound) if the skin is broken and closed (previously called simple) when the skin at the site of fracture is not broken [59].
At sites of preexisting abnormalities that weaken the bone, a minimal force that usually would not cause the fracture of a normal bone may produce a pathological fracture. A transchondral fracture (osteochondritis dissecans) represents fragmentation and separation of portions of cartilage or cartilage and bone. This type is most prevalent in adolescents and occurs typically in the head of the femur, ankle, kneecap, elbow, and wrist [59].
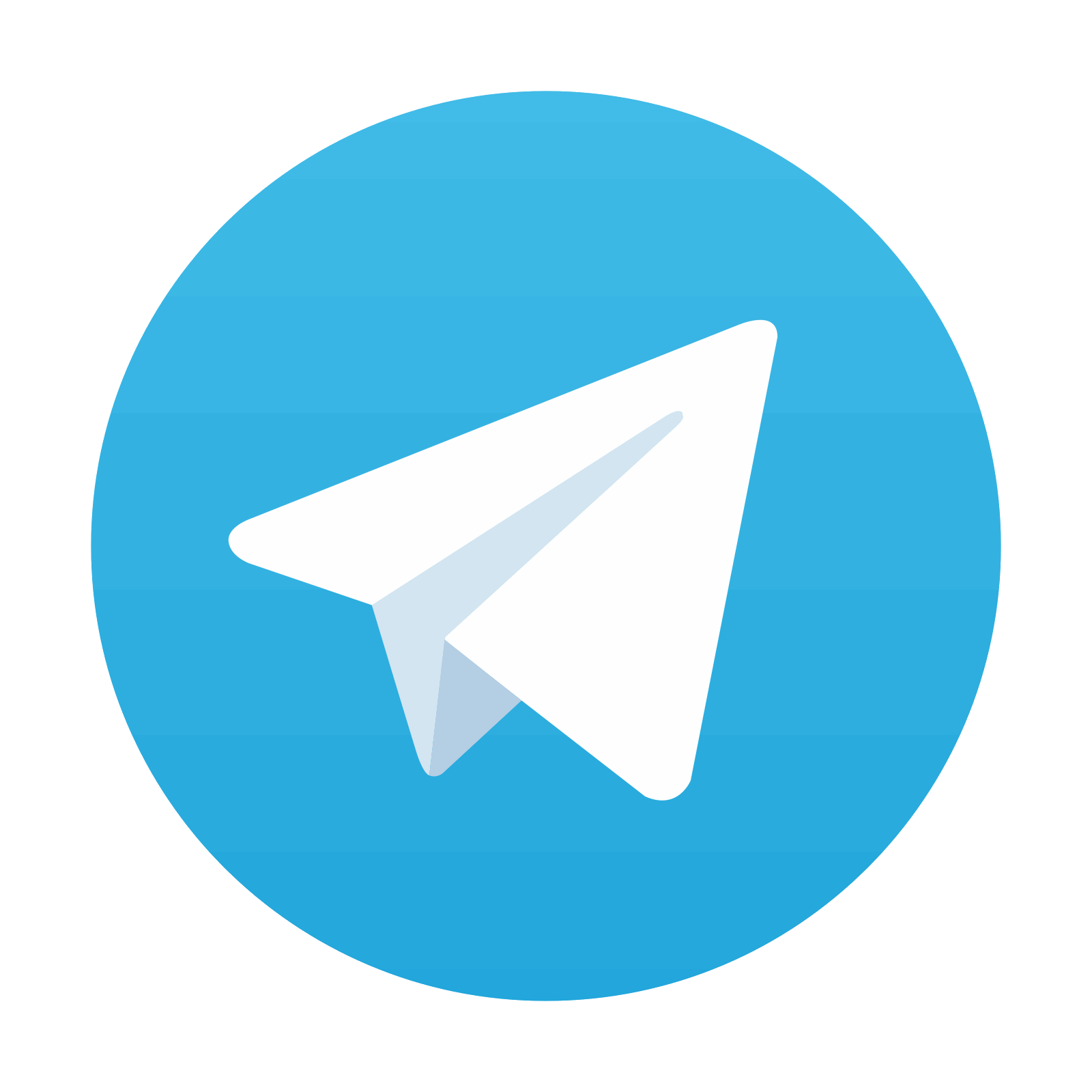
Stay updated, free articles. Join our Telegram channel

Full access? Get Clinical Tree
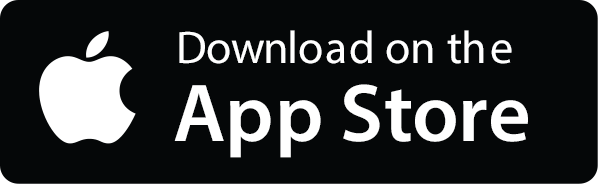
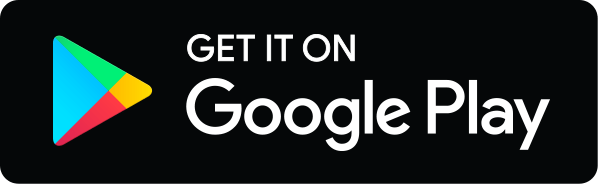
