Fig. 13.1
Images from a SPECT-MIBI scan of a 64 year old male with a high grade RCA stenosis. (a) Demonstrating no defect and rest (b) and a septal defect at stress indicative of reversible ischemia, and presumed viable myocardium
Echocardiography
Stress echocardiography consists of the utilization of physical or pharmacological stressors to standard 2D echocardiography. Physical and pharmacological ischaemic stressors include exercise, dobutamine or dipyridamole and have comparable diagnostic and prognostic accuracies as radionuclide stress perfusion imaging, with the added benefit of its low cost, wide and easy availability, and lack of radiation burden [7].
Stress echocardiography for the purpose of viability assessment relies on the functional behavior of myocardium at various levels of pharmacologic stress. Dobutamine is the pharmacological agent which is inexpensive and has been the most frequently used. Dobutamine stress echocardiography has been widely investigated and is highly specific in its use in viability assessment. The change in function, or lack thereof, is taken as being areas/segments of myocardium that lack viability (Table 13.1). A normal stress echocardiogram in patients suspected of having coronary artery disease equates to an excellent prognosis. The risks conferred from radionuclide stress perfusion imaging or coronary angiography can thus be safely avoided. Whilst stress echocardiography offers several advantages, it is highly operator dependent. Additionally, in the ischemic cascade, wall motion abnormalities (the basis of viability assessment with stress echocardiography) occur after perfusion defects, thus echocardiographic evaluation of viability would suffer from this disadvantage as well.
Table 13.1
Findings of stress echocardiography corresponding to varying degrees of myocardial dysfunction and viability
Rest + Stress = Diagnosis |
---|
Normokinesis + Normo-Hyperkinesis = Normal |
Normokinesis + Hypokinesis, Akinesis, Dyskinesis = Ischemia |
Akinesis + Hypokinesis, Normokinesis = Viable |
Akinesis, Dyskinesis + Akinesis, Dyskinesis = Necrosis |
Cardiac Magnetic Resonance Imaging
Cardiac magnetic resonance imaging (CMR) provides excellent spatial and temporal resolution and enables assessment of anatomy, function and perfusion. The use of CMR for viability assessment can be performed akin to echocardiography (utilizing a stress agent and evaluating myocardial function response) [8]. While assessment of altered wall motion during stress and rest adds specificity to a CMR, more commonly viability assessment with CMR relies on determination of scar burden. This is accomplished with the use of gadolinium contrast injection and delayed enhanced imaging with an inversion recovery sequence. Gadolinium is a T1 shortening agent, which becomes “trapped” in myocardial tissues demonstrating expanded extracellular space. The extracellular myocardial space can be expanded secondary to numerous mechanisms, thus not all delayed enhanced tissue in the myocardium represents true scar tissue (Fig. 13.2). The mechanism by which the extracellular space is expanded in nonviable myocardium (scar) is the lack of live myocytes, and a concomitant increase in fibrous tissue. The application of an appropriately timed inversion pulse to diminish signal from normal myocardium (which itself demonstrates enhancement, though not to the degree of scar) markedly improves the contrast resolution for scar detection, and is routinely performed at 10–15 min post contrast injection, thus the moniker “delayed contrast-enhanced cardiac magnetic resonance” (DCMR) (Fig. 13.3).
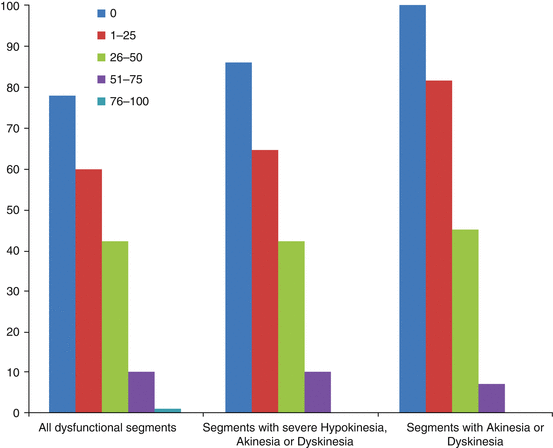
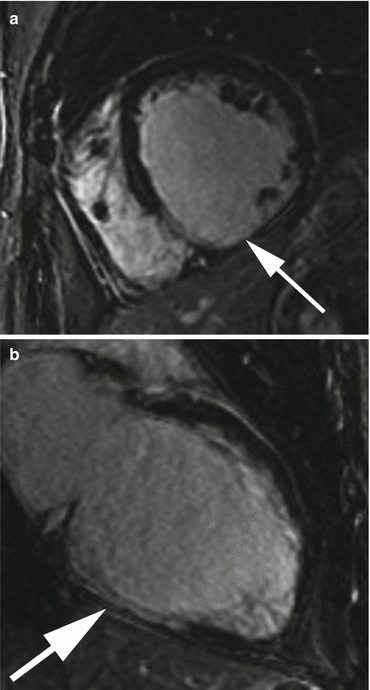
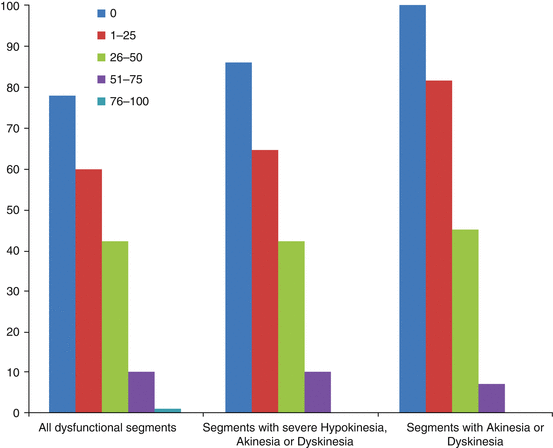
Fig. 13.2
Diagram depicting the extracellular distribution of gadolinium chelates in normal myocardial tissue
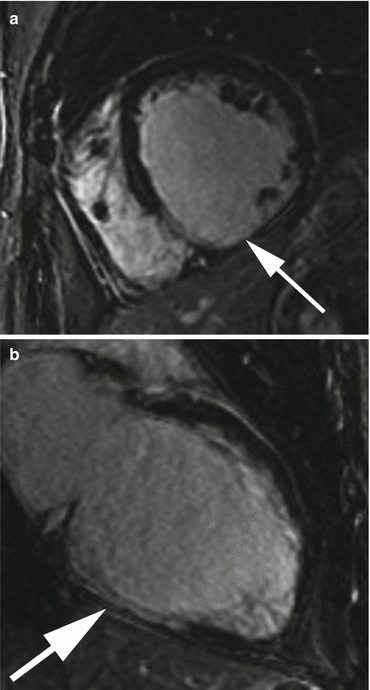
Fig. 13.3
Images from a cardiac MRI depicting near transmural delayed enhancement in the RCA territory (inferior wall) as seen on (a) short axis and (b) two-chamber long axis views. There is greater than 50 % transmural extension in the involved segments suggesting non viable myocardium in this patient with triple vessel disease. The lack of scar in the LAD and circumflex territories suggest possible recovery of function with revascularization in these segments which demonstrated moderate hypokinesis on CINE imaging (not pictured)
A landmark paper by Raymond Kim [9] demonstrated the ability of MRI to stratify the likelihood of recovery of myocardial function on the basis of the extent of transmural delayed enhancement (Fig. 13.4). At a cutoff of greater than 50 % transmural enhancement as detected by DCMR, it is felt that the probability of recovery is low enough to consider such a segment nonviable. It should be noted that, as outlined in Fig. 13.4, even in segments with enhancement less than 50 %, recovery is not guaranteed, and in fact when the degree of enhancement is beyond the immediate subendocardium, can be poor.
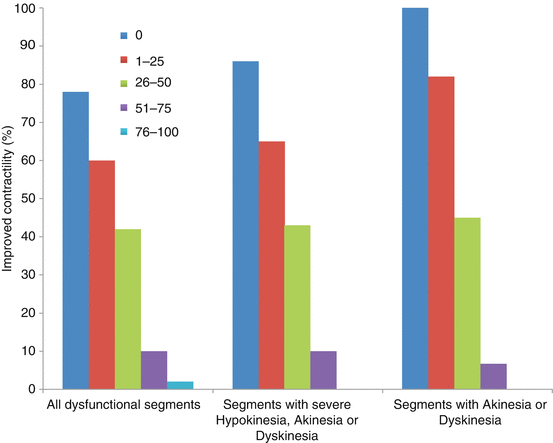
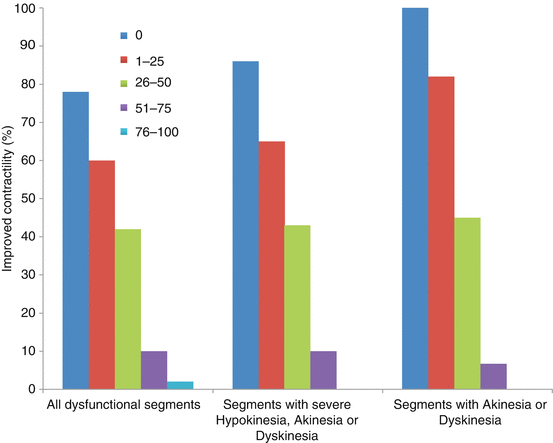
Fig. 13.4
Depiction of the likelihood of functional recovery in myocardial segments post revascularization as a function of the degree of transmurality of delayed enhanced tissue/scar as seen with DCMR
DCMR is limited in patients with implantable defibrillators, claustrophobia, or severe renal impairment unable to receive intravenous contrast. Other drawbacks are its high cost and lengthy procedure time. Additionally, there have been no large scale trials evaluating how integrating DCMR viability assessment into the workup of patients for potential revascularization affects patient outcome.
The decision regarding which modality to use is driven by local expertise and availability. SPECT and ECHO are considered suitable choices in a select patient population where PET and CMR are not available. However when these options are available, they are the preferred choice because of their higher overall accuracy. The results of viability imaging is just one facet in the decision making process. The clinical scenario, symptoms and patient comorbidities need to be considered before a treatment decision is made.
Evidence Behind Viability Assessment
While there is a reasonable basis to the theories of viability testing prior to revascularization, large scale randomized trials have failed to demonstrate an improvement in patient outcomes when revascularization decisions were guided by viability assessment. The most frequently mentioned of these trials is the STICH trial. This study was a multicenter, nonblinded, randomized trial evaluating the role coronary artery bypass grafting (CABG) played in patients with CAD amenable to CABG with reduced ejection fractions of 35 % or less [10]. A substudy of the STICH trial evaluated the effect viability assessment with either SPECT or low dose dobutamine stress echo had on patient outcome [11]. The results of this substudy demonstrated no significant association between myocardial viability, as determined by SPECT or echo, and patient outcome on multivariate analysis. Additionally, this trial did not demonstrate a significant interaction between viability and medical versus surgical management with regards to outcomes. These results differ from a relatively large body of literature suggesting a differential survival benefit between surgery and medical management based on viability assessment.
Many limitations of this study have been identified. Less than 50 % of the entire STICH cohort had viability assessment. In addition, the viability testing used is not classified as state of the art without integration of PET or MRI. Also it is probable that the actual results of the viability testing itself affected clinical decision making. The most pertinent limitation for the purposes of this chapter is the lack of focus on CMR or CT in this study. Though it has been suggested that even with if DCMR were evaluated as part of the STICH trial that the outcome would have been no different, one cannot be sure of such a conclusion [10]. It is plausible that the improved spatial resolution of CMR and CT for the discrimination of viable/non-viable myocardium would allow better selection of suitable revascularization candidates.
To date no large scale studies have been completed evaluating the outcomes of patients evaluated with CT for the purpose of myocardial viability assessment.
CT for Viability
The use of CT for viability assessment follows the same principles as DCMR. Iodine based IV contrast agents act to enhance the extracellular space just as gadolinium does. The discussion with regards to gadolinium enhancement kinetics in the previous section can be directly applied to iodine based agents as well. As such delayed enhanced images of the heart following iodine injection (such as used for routine cardiac CTA) can allow for scar detection.
One of the major benefits of using DCMR for scar detection is the improved ability to separate scar from normal myocardium afforded by the increased contrast resolution provided by the nulling of normal myocardium. CCTA for the purposes of scar detection does not have this luxury. When evaluating a CT for this purpose one has to detect scar (extensive hyperattenuation) on the background of myocardium which also enhances (moderate hyperattenuation). Thus attention to technique is paramount. Studies evaluating CT for the purposes of scar detection/viability assessment suggest to acquire delayed phase images earlier than done for DCMR (7 min vs 10–15 min), to use low kVp techniques to improve contrast resolution and to inject a larger volume of contrast than is normally done for CCTA [12].
Dual-energy CT (DECT) provides a very promising additional technical advance in allowing further differentiation of scar from normal myocardium when assessing for viability. DECT offers multiple possible ways to help improve the contrast resolution between scar and potentially viable myocardium. Reconstruction of lower monochromatic energy level datasets, and via material decomposition with iodine maps are two techniques that can help to improve the performance of viability imaging with DECT [13–15].
This concept is offers significant hope as the lower inherent contrast resolution of CT as compared to MRI typically hinders the ability to separate myocardial infarction/scar from normal myocardium.
Scanning at two different kVps nearly simultaneously allows creation of virtual monochromatic energy level images. Monochromatic energy levels can be created from data sets acquired with both dual source and single source scanners that employ rapid kVp switching. The benefit of reconstruction of monochromatic energy images from a dual energy system is that this can be done in the raw projection space, which has been shown to diminish beam hardening artifacts, which may remain a problem when evaluating virtual monochromatic energy images from a dual source system which are reconstructed in image space [16]. In an animal model the diminution in beam hardening artifact, especially in the basal inferolateral wall, has been documented using rapid kVp switching dual energy with datasets reconstructed at 70 keV in projection space [16] (Fig. 13.5).
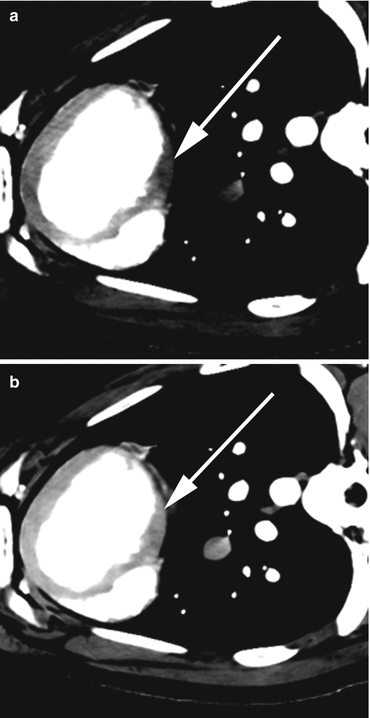
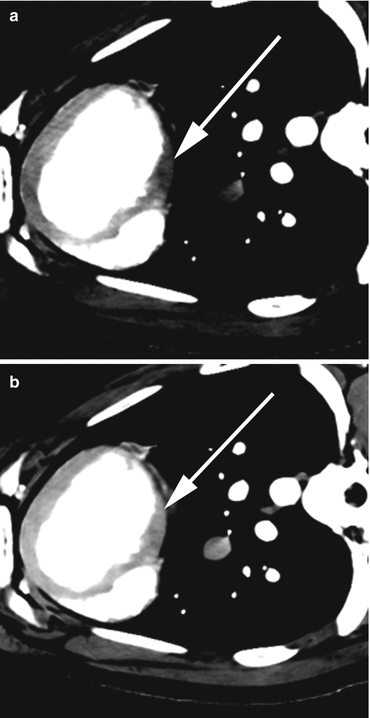
Fig. 13.5
(a) Images from a dual energy CT examination in a porcine model, demonstrating beam hardening/low attenuation artefact (white arrow) in the inferobasal myocardium at 140 kVp. (b) This artefact is reduced (white arrow) on the 70 kEv monochromatic energy image created from the source 100 kVp (not pictured) and the 140 kVp datasets
In addition to the advantages of increased myocardial homogeneity and reduction in beam hardening, dual energy cardiac CT allows for the selection of an energy level closer to the K-edge of iodine (33.2 keV) which can enable the maximizing of the attenuation of iodine containing areas, such as scar [17]. This technique has been explored when evaluating for pulmonary emboli with low contrast volume techniques [15]. One must be aware that as one creates virtual monochromatic data sets of based on lower keV values, image noise will increase but contrast to noise ratio is generally maintained (Fig. 13.6). This is where the added benefit of reconstruction in projection space and diminishing beam hardening artifacts for lower monochromatic energy levels, closer the K-edge of iodine may be realized.
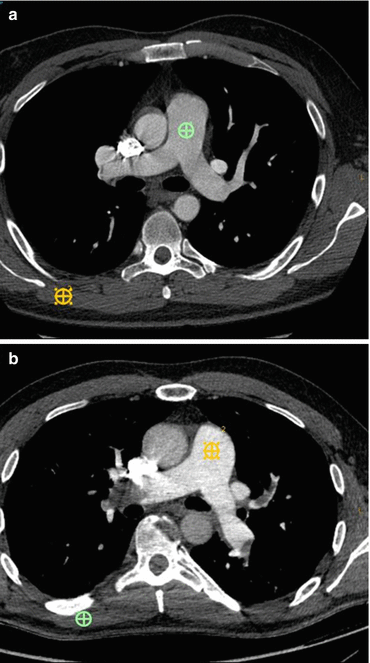
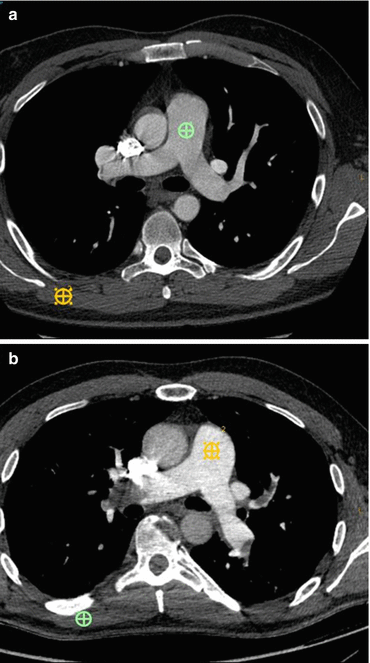
Fig. 13.6
Images from different patients undergoing CT exams for PE. (a) Depicts a standard 120 kVp dataset, with reduced image noise (standard deviation of green region of interest (ROI) in the pulmonary artery [PA]=20 HU) but average CNR of 12 (calculated by the attenuation of the pulmonary artery – attenuation of the latisimuss dorsi [orange ROI]/noise in the PA). (b) Is a virtual monochromatic 50 kEv dataset, demonstrating increase image noise (SD of the orange region of interest in the PA=26 HU), but because of the improved contrast afforded by imaging close to the k-edge of iodine the CNR is actually greater, calculated as before at 14
For detection of scar and thus viability with a dual source CT, one group also looked at the creation of linearly blended datasets using set average weighting factors for the raw data at 100 and 140 kVp. They found that images created from a linear blend of 60 % 100 kVp and 40 % 140 kVp data improved contrast to noise ratio, signal to noise ratio and accuracy of measured infarct volume [18]. It is possible that images created from non linear blending offer an even better way to evaluate for scar [19].
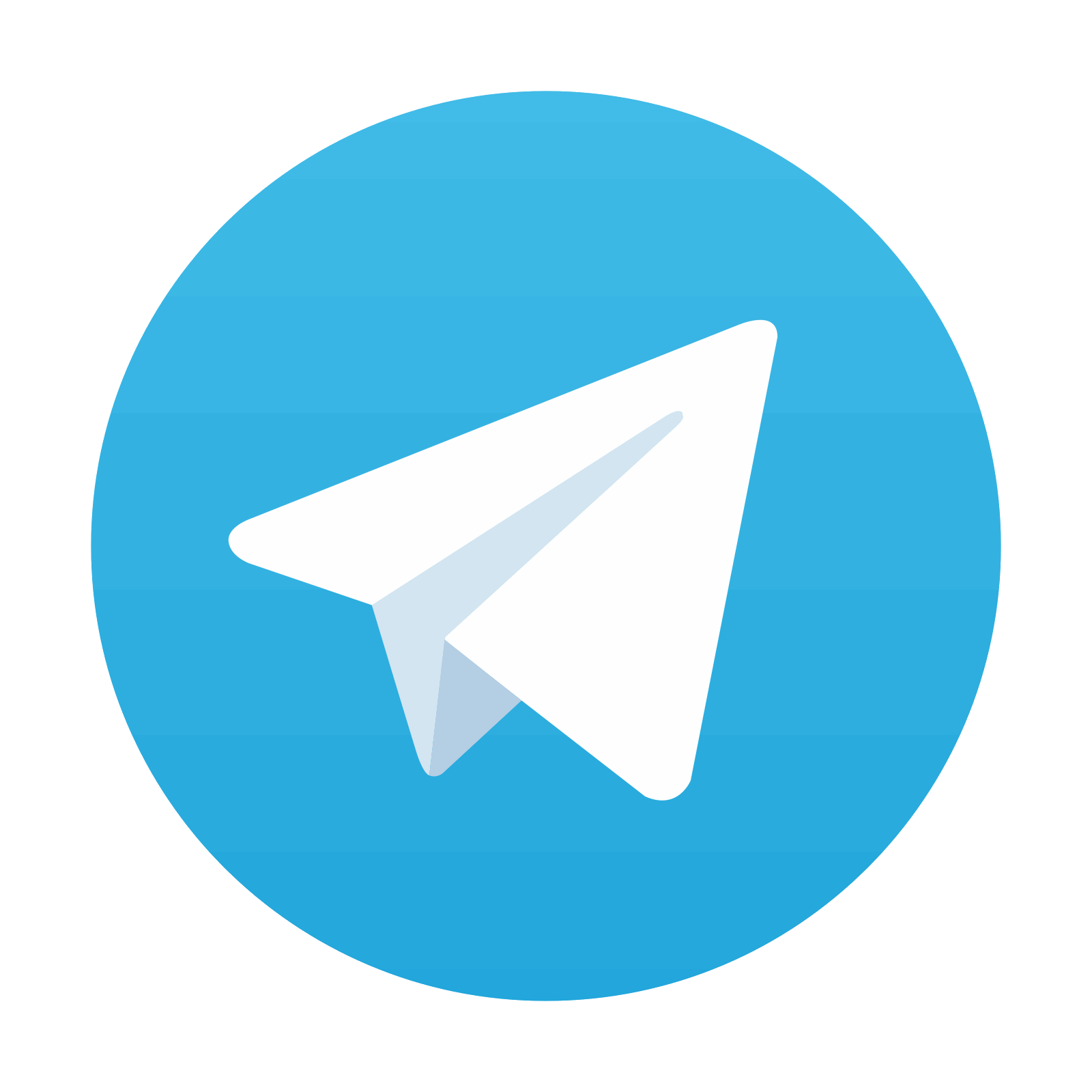
Stay updated, free articles. Join our Telegram channel

Full access? Get Clinical Tree
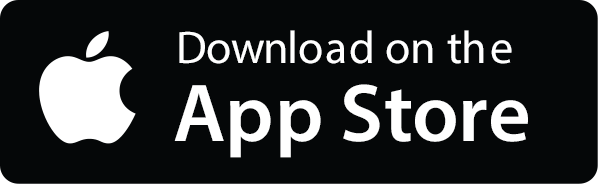
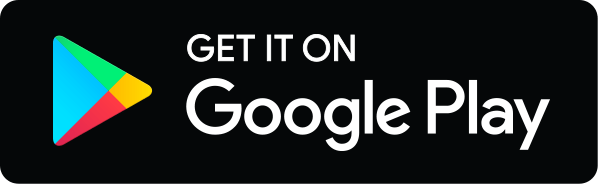