Stomach, ileum, colon
1. Well-differentiated endocrine tumor (“carcinoid”)
(A) Benign behavior
Confined to mucosa–submucosa, nonangioinvasive
Size: £1cm (stomach and small intestine) or £2 cm (colon)
(B) Uncertain behavior
Confined to mucosa–submucosa, angioinvasive
Size: >1 cm (stomach or small intestine) or >2 cm (colon)
2. Well-differentiated endocrine carcinoma (“malignant carcinoid”)
Low-grade malignant tumor
Deeply invasive or metastatic
3. Poorly differentiated endocrine carcinoma
Small-cell carcinoma
High-grade malignant tumor
4. Mixed endocrine/exocrine carcinoma
Moderate- to high-grade malignant tumor
Pancreas
1. Well-differentiated endocrine tumor
(A) Benign behavior
Confined to the pancreas, nonangioinvasive
Size: <2 cm; mitoses: £2; Ki67 positive cells/10 HPF: ≤2%
(B) Uncertain behaviour
Confined to the pancreas
Size: ³2 cm; Mitoses: >2, or angioinvasive; Ki67 positive cells/10 HPF: >2%
2. Well-differentiated Endocrine Carcinoma Low-grade malignant tumour with gross local invasion/metastases Ki67 positive cells/10 HPF: >5%
3. Poorly-differentiated Endocrine Carcinoma Small-cell carcinoma High-grade malignant tumour; Ki67 positive cells/10 HPF: >15%
4. Mixed Endocrine/Exocrine Carcinoma Moderate to high-grade malignant tumour
Table 18.2
WHO 2010 working principles for neuroendocrine neoplasms
Neoplasm definition to embrace all grades of endocrine tumors (low, intermediate, and high grade) |
Neuroendocrine connotation recommended as description of shared neural–endocrine markers |
Standard definition of neuroendocrine tumor (NET) for low- to intermediate-grade neoplasms and neuroendocrine carcinoma (NEC) for high grade |
NET subdivision according to grade (ICDO codes 8240/3 and 8249/3); potential malignancy formalized |
NEC subdivision in LCNEC and SC-NEC (ICDO codes 8013/3 and 8041/3) |
Synonyms for NET: carcinoid, well-differentiated endocrine tumor/carcinoma; for NEC: poorly differentiated endocrine carcinoma, high-grade neuroendocrine carcinoma |
TNM recommended (AJCC–UICC–WHO 2010) |
For carcinoids only (excluded G3 neoplasms) |
Blueprint from ENETS proposals 2006–2007 for stomach, ileum, and colon but with significant differences for appendix and pancreas (generate data) |
Diagnosis by site according to the above uniform grading and staging parameters |
Minimal histopathology report recommendation |
Neoplasm definition as NET or NEC |
Grade definition as G1–G3 |
pTNM definition (when possible) |
Table 18.3
WHO 2010 general neuroendocrine neoplasm categories
WHO 1980 | WHO 2000 | WHO 2010 |
---|---|---|
I Carcinoid | 1. Well-differentiated endocrine tumor (WDET)a | 1. NET G1 (carcinoid)b |
2. Well-differentiated endocrine carcinoma (WDEC)a | 2. NET G2b | |
3. Poorly differentiated endocrine carcinoma/small cell carcinoma (PDEC) | 3. NEC (large cell or small cell type)b, c | |
II Mucocarcinoid | 4. Mixed exocrine–endocrine carcinoma (MEEC) | 4. Mixed adenoneuroendocrine carcinoma (MANEC) |
III Mixed forms carcinoid–adenocarcinoma | ||
IV Pseudotumor lesions | 5. Tumor-like lesions (TLL) | 5. Hyperplastic and preneoplastic lesions |
Table 18.4
AJCC anatomic stage and prognostic groups for neuroendocrine tumors
Group | T category | N category | M category |
---|---|---|---|
Stage 0 | Tis | N0 | M0 |
Stage I | T1 | N0 | M0 |
Stage IIA | T2 | N0 | M0 |
Stage IIB | T3 | N0 | M0 |
Stage IIIA | T4 | N0 | M0 |
Stage IIIB | Any T | N1 | M0 |
Stage IV | Any T | Any N | M1 |
In order to assure an optimal treatment of gastroenteropancreatic neuroendocrine tumors (GEP NETs), a standardized diagnostic procedure is required. The European Neuroendocrine Tumor Society (ENETS) has developed diagnostic and prognostic stratification criteria, based on histological typing, differentiation, grading, and TNM staging. Immunostaining for the neuroendocrine markers synaptophysin and chromogranin and for the proliferation marker Ki67/MIB1 is mandatory, while immunostaining for hormones, receptors, and other markers is optional. The grading proposal stratifies tumors in G1 (<2 mitotic counts/10 HPF and/or Ki67 index ≤2%), G2 (2–20 mitotic counts/10 HPF and/or Ki67 index between 3%—intended as >2%—and 20%), and G3 (>20 mitotic counts/10 HPF and/or Ki67 index >20%).[15] The tumor grading, together with histopathology type and staging, reflects on the potential metastatic spread and, therefore, on the therapy options (surgery, biotherapy, and chemotherapy) [16].
This classification applies easily to NETs of the gastroenteropancreatic pancreatic (GEP) tract (Table 18.1), while it has been criticized for lung (and thymic) tumors, which are often classified according to Travis. Travis recognized low-grade NETs—typical and atypical carcinoids—and high-grade NETs, large-cell neuroendocrine carcinoma (LCNEC) and small-cell lung cancer (SCLC). The most prevalent high-grade NET, accounting for 89% of cases according to the SEER database of National Cancer Institute, is SCLC. The WHO diagnostic criteria for a typical carcinoid are carcinoid morphology and <2 mitoses/10 HPF (high-power field), absent necrosis, and 0.5 cm or larger. An atypical carcinoid has carcinoid morphology with 2–10 mitoses/10 HPF and/or areas of necrosis [17–19].
Most NETs are sporadic, but occasionally, they may be part of inherited syndromes, known as multiple endocrine neoplasia type 1 and 2 (MEN1 and MEN2). Five other inherited diseases show a more heterogeneous clinical pattern, with mainly nonendocrine tumors (von Hippel–Lindau (VHL), neurofibromatosis type 1 (NF1), tuberous sclerosis, succinate dehydrogenase, and McCune–Albright syndromes). Germline mutations in five genes have been recognized to be responsible for familial pheochromocytomas: the von Hippel–Lindau gene (VHL), causing VHL syndrome; the RET gene, leading to multiple endocrine neoplasia (MEN) type 2; the neurofibromatosis type 1 gene (NF1), associated with von Recklinghausen’s disease; and the genes encoding the B and D subunits of mitochondrial succinate dehydrogenase (SDHB and SDHD), which are associated with familial paragangliomas and pheochromocytomas. Hereditary GEP or bronchial NET and pituitary adenomas occur in MEN type 1.
The frequency of a malignant course of chromaffin tumors, pheochromocytomas, and paragangliomas depends strongly on the genetic background. According to the 2004 WHO classification, pheochromocytomas can be diagnosed as malignant only by the presence of metastases, usually to the liver, lymph nodes, lung, and bone, either at diagnosis or during follow-up (according to the so-called 10% rule, namely 10% bilateral, 10% extra-adrenal, 10% familial, 10% malignant) [13, 20]. Attempts to establish a histopathological score, considering the presence of diffuse cellular growth, high cellularity and monotony, tumor cell spindling, atypical mitotic figures, high mitotic count, etc., failed to assess the malignant potential due to inter- and intra-observer variation [21]. Malignant pheochromocytomas account for about 10% of all pheochromocytomas and are associated with capsular invasion and large tumor size (>5 cm in size and >80 g in weight) [22]. Pheochromocytomas in patients with MEN type 2, more than 90% of those in VHL disease, and almost 90% of those in NF1, are malignant [23]. Malignant paragangliomas have a higher prevalence, 15–35% when associated to the SDHB gene mutations [24–26].
In addition to their hormonal profile, NETs often have neuroamine uptake mechanisms and/or specific receptors, such as norepinephrine transporter and cell membrane somatostatin receptors, which can be of crucial importance in their localization and treatment [27].
Clinical Presentation
NETs, particularly those of GEP origin, tend to be well differentiated and slow growing, with a minority of aggressive forms, and therefore, they are often diagnosed when they have already metastasized, usually to the liver; thus curative surgery is no longer possible. They may present with symptoms related to the inappropriate peptide and neuroamine hypersecretion or, especially the nonfunctioning ones, which are the majority, with symptoms related to their mass effect. Even in functioning tumors, despite the presence of a distinct clinical syndrome, symptoms are frequently unrecognized, and diagnosis is often delayed.
Gastroenteropancreatic NETs
Gastric NETs (the so-called carcinoids) are typically multiple, small, and usually benign. They are associated with hypergastrinemia and can be of type 1, following atrophic gastritis, or type 2, in the Zollinger–Ellison syndrome. Type 3 gastric carcinoids are not associated with hypergastrinemia and are single large lesions, usually with distant metastases. Duodenal NETs frequently secrete gastrin and cause Zollinger–Ellison syndrome, as part of MEN1. Intestinal and appendix NETs (the so-called classic “carcinoids,” described by Oberndorfer) derive from enterochromaffin cells and are frequently nonfunctioning until they metastasize. Examples of functioning tumors are metastatic intestinal or appendicular carcinoids that may present with the carcinoid syndrome, typical or atypical, with cutaneous flushing, diarrhea, and abdominal pain, due to the overproduction and release in the systemic circulation of bioactive amines, mostly serotonin and histamine. Thirty to 40% of nonmetastasized carcinoids are discovered at an earlier stage during emergency surgery for acute abdomen [28]. Colonic carcinoids are frequently large, nonfunctioning, with a poor prognosis, while rectal lesions are small and rarely metastasize.
NETs in the pancreas are usually large, and up to 50% have synchronous liver metastases. Functioning pancreatic islet cell tumors may present with syndromes related to the hyperproduction of insulin, gastrin, VIP, glucagon, somatostatin, etc., thus forming markedly different clinical and pathological entities. Insulinomas are often small and benign lesions causing hypoglycemia. Pancreatic gastrinomas are less common than in the duodenum but generally malignant, causing Zollinger–Ellison syndrome in 25% of cases associated with MEN1. Glucagonomas cause diabetes and a characteristic rash (necrolytic migratory erythema). Finally, VIPomas may produce severe diarrhea, hypokalemia, and achlorhydria (WDHA or Whipple syndrome). Rare tumors may secrete ACTH, GH-RH, PTH-RP, and somatostatin [29].
Thoracic NETs
Thoracic NETs include typical and atypical carcinoids, LCNEC and SCLC, are the most common. The majority of patients with bronchial NETs have symptoms at presentation, such as cough, hemoptysis, and pneumonia (a classical triad), resulting from the lumen obstruction and ulceration of the tumor. Typical carcinoid presents characteristically as a central lesion, with signs and symptoms of bronchial obstruction. They have a relatively benign biological behavior. Carcinoid syndrome occurs when liver metastases are present, allowing the active amines produced by the tumor to enter the systemic circulation. Other syndromes related to hormonal overproduction include Cushing syndrome, due to ACTH secretion, and acromegaly, due to GH-RH secretion. Atypical carcinoids, frequently peripheral and functioning, are considered biologically aggressive, with lymphatic and hematogenous metastases. Large-cell neuroendocrine cancers are aggressive and rare form of NET, usually metastatic at diagnosis, causing rapid clinical deterioration. Paraneoplastic syndromes are sporadic. SCLCs are particularly aggressive and are associated with early hematogenous and lymphatic metastasis. SCLCs are extremely chemosensitive but usually relapse. Mediastinal syndrome, caused by lymph node metastases, is frequent at clinical presentation and is often accompanied by distant metastases, typically in the brain or bones. In many cases, paraneoplastic syndromes occur, particularly Cushing syndrome and the syndrome of inappropriate antidiuretic hormone secretion (SIADH) [30–32].
Chromaffin Cell NETs
Chromaffin cell tumors present with variable signs and symptoms, mostly related to catecholamine hypersecretion (pheochromocytoma has been described as “the great mimic”). The most frequent findings are hypertension, tachycardia, headache, pallor, and feelings of panic or anxiety. Hypertension is usually paroxysmal, sometimes severe, leading to hypertensive emergencies, superimposed on a state of normal blood pressure or of hypertension. Hyperglycemia, lactic acidosis, weight loss, nausea, fever, and flushing may also occur. Sometimes blood pressure may be normal, when tumor load is limited or when dopamine is preferentially secreted. In rare cases of prevalent epinephrine secretion, patients may present with hypotension [33].
Pheochromocytomas are catecholamine-producing NETs, also called neuroectodermal tumors, that originate from chromaffin cells located in the adrenal medulla or in extra-adrenal paraganglia. Extra-adrenal tumors are called extra-adrenal pheochromocytomas or paragangliomas. These tumors are found along the paravertebral and paraaortic axes, with a neck-to-pelvis distribution. Head and neck paragangliomas do not usually secrete catecholamines. The large majority (80–85%) of pheochromocytomas arise from the adrenal medulla, while about 15–20% are extra-adrenal. Catecholamine-producing paragangliomas are usually located in the abdomen [21, 33].
Biochemical Profile
Together with histopathologic analysis and clinical evaluation, serum hormone assays are important for diagnosis. Some markers are common to the majority of NETs, among these chromogranins A and B, pancreatic polypeptide, NSE, and α and β chains of chorionic gonadotropin. On the other hand, specific hormone markers suggest specific tumors, such as serotonin or its metabolite 5-hydroxy-indole-acetic acid (5-HIAA) in gastrointestinal and bronchial carcinoids, insulin and C-peptide in pancreatic insulinomas, or gastrin in duodenal gastrinomas.
Plasma CgA is a very sensitive (99%) marker of NETs. It correlates with tumor volume and with the outcome of therapy, although it is nonspecific, as it is elevated also in SCLC and in prostate carcinoma. Main causes of false-positives are therapy with proton pump inhibitors, renal impairment, atrophic gastritis, untreated hypertension, and pregnancy. It has been demonstrated that heterophile antibodies, due to autoimmune diseases or to sensitization to rodent proteins, may interfere with the assay. Recently, it has been suggested that alkaline phosphatase is a better predictor of survival than CgA [34–36].
Functioning chromaffin cell tumors secrete catecholamines, namely norepinephrine, epinephrine, and dopamine. These agents are catabolized to metanephrines, which may be found in high concentration in the urine. Urinary and plasma catecholamines, urinary metanephrines (normetanephrine and metanephrine), and urinary vanillylmandelic acid are usually measured for diagnosis. Currently, the plasma and 24-h urinary measurement of free metanephrines is the most sensitive method to accurately diagnose a pheochromocytoma/paraganglioma (96–100% and 92–99% sensitivity, respectively, and 87–92% and 64–72% specificity, respectively) [37]. Due to its low sensitivity and specificity, CgA is not used in chromaffin cell tumor assessment [38]. To avoid false-positive results, biochemical evaluation should not be performed while the patient is taking drugs that can cause increased catecholamines, such as phenoxybenzamine and tricyclic antidepressants, monoaminooxidase inhibitors, calcium channel blockers, and caffeine. Testing patients while they are taking these drugs is a major cause of false-positives. Dynamic tests, such as the clonidine test, are sometimes performed in norepinephrine-secreting tumors [39]. Head and neck paragangliomas are rarely hormonally active.
Role of Diagnostic Imaging
The localization of a NET and the assessment of the extent of disease are crucial for management. Diagnostic techniques include computed tomography (CT), magnetic resonance imaging (MRI), ultrasonography (US), contrast-enhanced US (CEUS), endoscopic US (EUS) and intraoperative US (IOUS), selective angiography with hormonal sampling, and nuclear medicine imaging by 111In-pentetreotide (OctreoScan®), 123I-metaiodobenzylguanidine (123I-MIBG), 99mTc-EDDA/HYNIC-Tyr3-octreotide scintigraphy, or more recently, somatostatin receptor PET with 68Ga-octreotide, 18F-DOPA, 18F-dopamine, and [11C]5-hydroxytryptophan. No technique is the gold standard, and specific sequences of exams might be needed for each tumor type. A combination of two or more imaging techniques is usually required for diagnosis and staging. Despite all efforts, a number of NETs (up to 50%) remain with an unknown primary site. Usually, radiological techniques (such as ultrasound, CT, or MRI) are useful in the localization of the primary tumor, particularly if nonfunctioning, while nuclear medicine aids in the evaluation of the extent of disease, staging, and therapy decision making.
Principles of the Clinical Use of Specific Radiotracers for Molecular Imaging of NETs
Nuclear medicine imaging is useful because it depicts target expression and function of macroscopic lesions within tissues. In fact, NETs are specifically characterized by the presence of neuroamine uptake mechanisms and/or somatostatin receptors at the cell membrane. Moreover, since high-glucose metabolism of tumors is directly related with cell proliferation (depending on the genetic expression of glucose transporters (GLUT)) [40], the capability of NET cells to accumulate [18F]FDG typically is related to cell differentiation and high malignancy. Thus, a high-glucose metabolic state can provide prognostic information. On the other hand, [18F]FDG PET does not provide useful information for tumors that are relatively slow growing [41].
Over the last 20 years successful imaging of NET involved the use of radiolabeled somatostatin analogs. Somatostatin is a peptide that exists in either a 14-amino-acid or a 28-amino-acid form. It is present in the hypothalamus, brainstem, gastrointestinal tract, and in the pancreas. SSTRs are found on cells of neuroendocrine origin as well as on activated lymphocytes. Six subtypes of SSTRs have been identified by molecular analysis (named sst1, sst2a, sst2b, sst3, sst4, and sst5). Each receptor exerts its action by inhibiting adenylyl cyclase activity. Each receptor subtype has a different binding affinity for native somatostatin and for somatostatin analogs. Subtype 2 has the highest affinity (K d 0.1–1 nM), whereas subtypes 3 and 5 are within the 10–100 nM range, and subtypes 1 and 4 exhibit a low affinity. Moreover, since it is most frequently expressed in NET tumors, somatostatin receptor subtype 2 is the best target for imaging. A strength of somatostatin imaging is that SSTRs density is markedly elevated in malignant tissues, from 80 to 2,000 fmol/mg protein compound, whereas the expression within normal neuroendocrine tissues is relatively low. SSTRs are overexpressed in GEP NETs [42].
So far, all radiotracers used for SSTR imaging are based on octreotide, which is a long-acting analog of the human hormone, somatostatin. The presence of octreotide-binding sites on tumors allows their in vivo visualization after the injection of the radionuclide-labeled octreotide analog. 123I-[Tyr3]-octreotide was the first radiocompound used for this purpose. However, its relatively short effective half-life and the high background of radioactivity within the abdomen were drawbacks that limited its clinical application. 111In-[DTPA D-Phe]-octreotide, or 111In-pentetreotide, has been used in subsequent development because of the more favorable stability and imaging characteristics in the delayed images. It is a registered trademark of Mallinckrodt Inc. with the name of OctreoScan®. Octreotide binds with high affinity to the sst2 and with moderate affinity to the sst3 and sst5 receptors.
NETs take up amino acids and transform them into biogenic amines by means of decarboxylation. In fact, they were also called APUD (amine precursor uptake and decarboxylation) tumors. NET cells can synthesize catecholamines in an enzymatic pathway, which begins from converting the amino acid tyrosine into l-DOPA. l-DOPA is decarboxylated to dopamine, oxidized to norepinephrine, and methylated to epinephrine, which is transported into synaptic vesicles. Reuptake of catecholamines is done presynaptically by norepinephrine transporters present on the cell membrane. For this reason, NETs can be imaged with both 18F-DOPA PET, which accumulates within cells due to the high activity of the aromatic amino acid l-DOPA decarboxylase, and radioiodinated MIBG which is taken up by the cell through the norepinephrine transporter and stored in neurosecretory vesicles by means of the catecholamine transporter. 18F-DOPA or 18F-dopamine PET and MIBG scintigraphy are highly sensitive for detecting tumors arising from the adrenal medulla, but they can be also taken up by non-adrenomedullary NETs.
Imaging of GEP NETs
Conventional Radiologic Imaging
Contrast-enhanced three-phase CT and MRI are useful for the detection of primary tumors and metastatic disease. The sensitivity and specificity of CT are 73 and 96% for pancreatic NETs and 82 and 92% for liver metastases. Mean sensitivity and specificity of MRI are 93 and 88% for pancreatic NETs, respectively; detection rates of 82 and 95% are reported for liver metastases. Since NETs are typically hypervascular, CT and MRI are performed early after the bolus of contrast is injected (arterial phase) (Fig. 18.1). Although tumor arterial enhancement is homogeneous in small NETs, heterogeneous enhancement is more common in lesions larger than 3 cm. However, the degree, uniformity, and timing of enhancement can be highly variable, and other lesions, such as intrapancreatic accessory spleen, can be commonly mistaken for a NET. Moreover, vascular heterogeneity is considered to be a sign of malignancy since malignant lesions often have heterogeneous vascular structure, perfusion, and vascular permeability [43, 44].
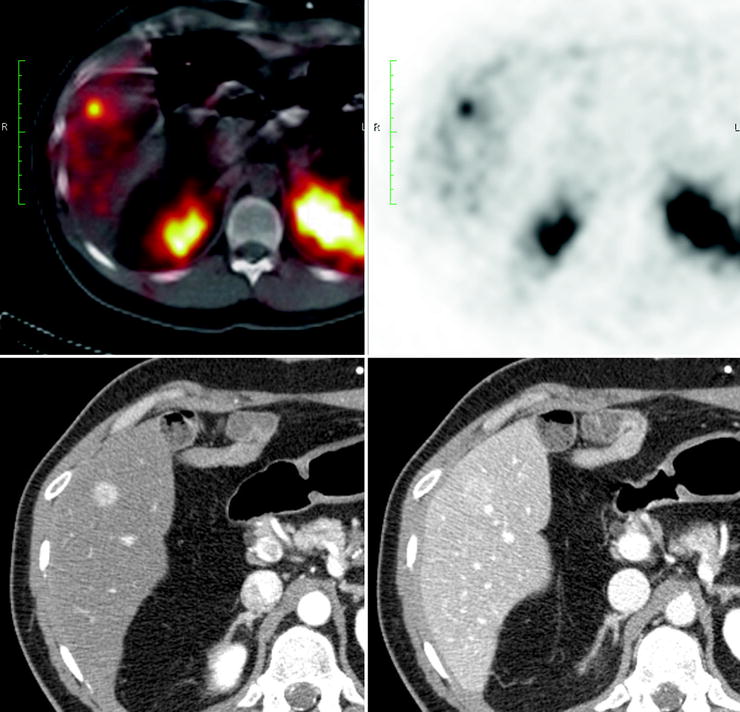
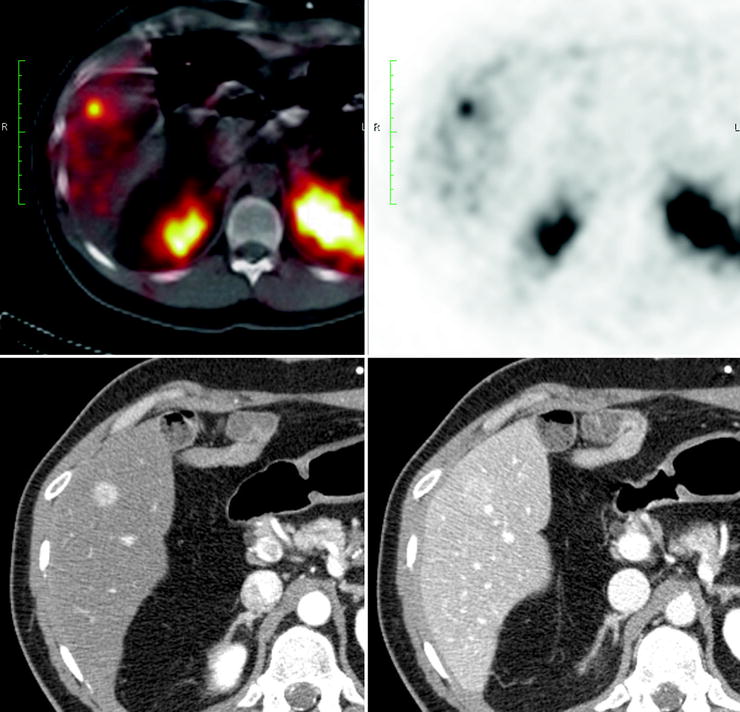
Fig. 18.1
Patient with a liver metastasis from an ileal neuroendocrine tumor. Upper images show SPECT/CT images demonstrating uptake of 111In-pentetreotide within a liver metastasis. The bottom left image shows the metastasis with a typical contrast enhancement on CT during the arterial phase. The lesion enhancement decreases during the venous phase when compared to the liver parenchyma (bottom right image)
Localization of GEP NETs may be difficult because they often present as small lesions and their anatomic location is widely variable. EUS is mainly useful in the diagnosis and staging of small and intramural lesions of the duodenum, pancreas, stomach, and rectum and can detect up to 60% of duodenal and up to 100% of pancreatic lesions. EUS and IOUS have a detection rate of 92% for insulinomas. Although the diagnostic accuracy of US for the detection of pancreatic NETs is high, the best performance of US is for the diagnosis and follow-up of liver metastases (sensitivity and specificity are 88 and 95%, respectively) [27, 28].
GEP NETs can spread to small-bowel mesentery. Mesenteric disease can be identified on CT as a contrast-enhancing mass containing calcifications [45]. Moreover, angio-CT can be useful to depict the involvement of vascular structures (such as mesenteric arteries) that may preclude surgical resection [46]. However, CT and MR imaging cannot always differentiate tumors and mesenteric metastasis from intestinal structures. Lymphoma or retractile mesenteritis can also have a similar CT appearance [47].
The liver is a common site of metastases. The metastatic lesions are typically hypervascular, and most can be detected on CT or MR images acquired during the hepatic arterial phase. However, liver metastases may be hypovascular (Fig. 18.2). Moreover, larger metastases may show heterogeneous enhancement due to central necrosis. For liver metastases, MRI fast spin echo has a high sensitivity while a single-shot sequence allows optimal distinction between hypervascular metastases from hemangiomas. Contrast-enhanced CT and MR imaging can also disclose occasional metastases to other intra-abdominal organs. Metastases to bone are easily missed on CT. Although MR imaging has a sensitivity of nearly 100%, the appearance of lesions may be identical to that of bone metastases from other primary tumors. Contrast-enhanced CT, MR imaging, and US are routinely used for monitoring lesions. Finally, video capsule and double-balloon enteroscopy can identify otherwise undetectable small intestine tumors [48–51].
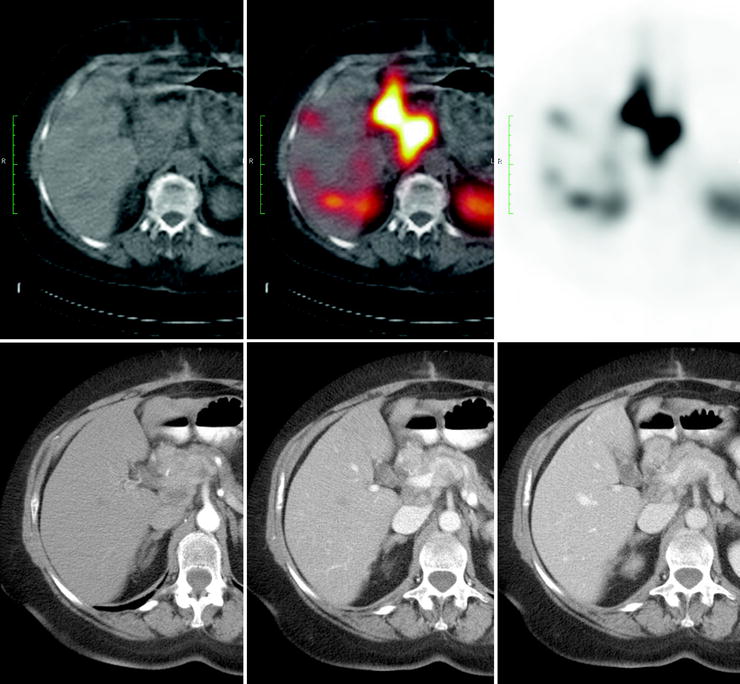
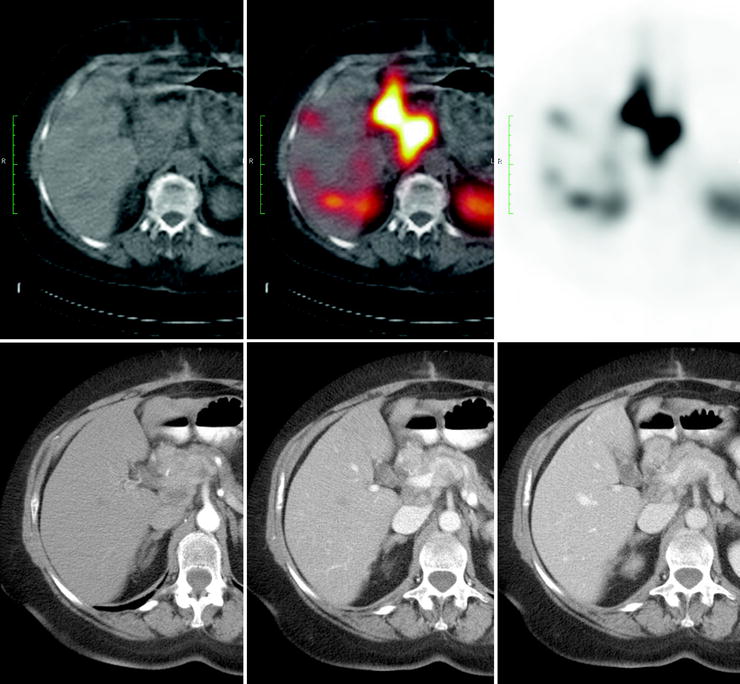
Fig. 18.2
Patient with a pancreatic NET and multiple liver metastases that result positive at SRS but negative at the three-phase contrast-enhanced CT
Nuclear Medicine Imaging
Somatostatin receptor scintigraphy (SRS) with OctreoScan® is useful for detecting NETs. However, there is a low sensitivity (50–60%) for detecting insulinomas, which are characterized by low expression of sst2 receptors [52]. Combined SST and anatomic imaging correctly localized in 81–96% of cases. In pancreatic NETs, contrast-enhanced three-phase CT or MRI is able to localize 60–94% of the primaries, angiography up to 75%, and SRS with OctreoScan® 77–85% of the primary lesions [53]. Recently, the use of SPECT/CT hybrid equipment can provide the surgeon with detailed functional and topographic information for tissue sampling and resectability [54] (Fig. 18.3).
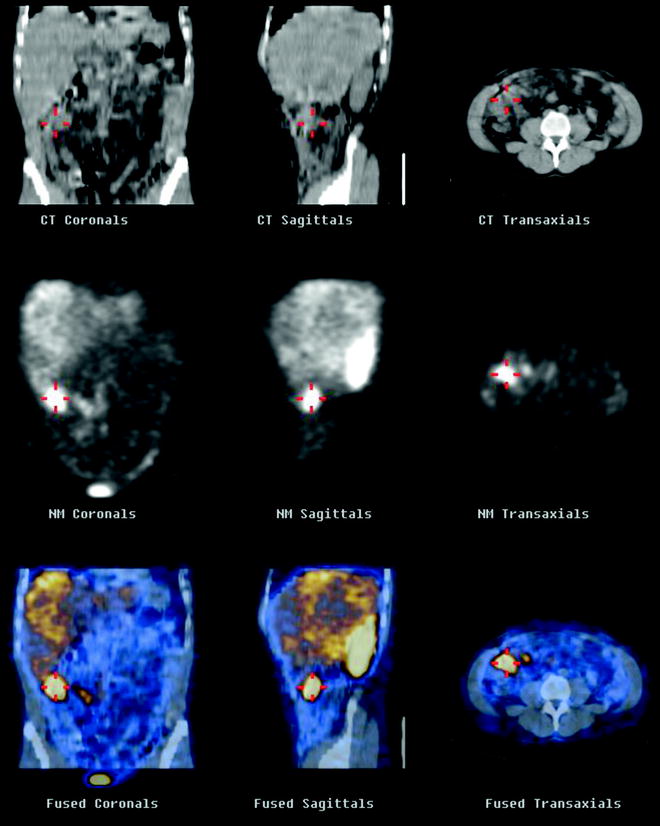
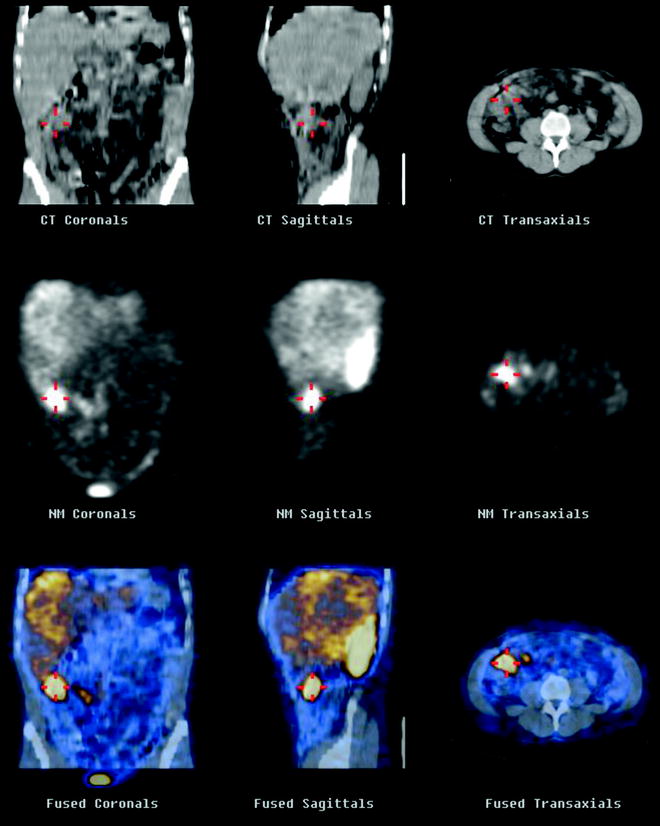
Fig. 18.3
SPECT/CT shows uptake of 111In-pentetreotide within a NET of the ileum
Information from Octreoscan® images modified the therapeutic strategy in ∼53% of cases by accurately staging NETs, especially by detection of metastases [55, 56]. The sensitivity of SRS for detecting metastases is 61–96%. Although MRI is considered to have the highest sensitivity for liver metastases, SRS is the most accurate imaging modality for the “one-shot” detection of liver and extrahepatic metastases in patients with GEP NETs (Fig. 18.4). This also applies to insulinomas, as it is believed that secondary insulinoma lesions may show positive SSTR more often than the primary [57–59]. However, the size of liver metastases seems to significantly affect sensitivity of SRS.
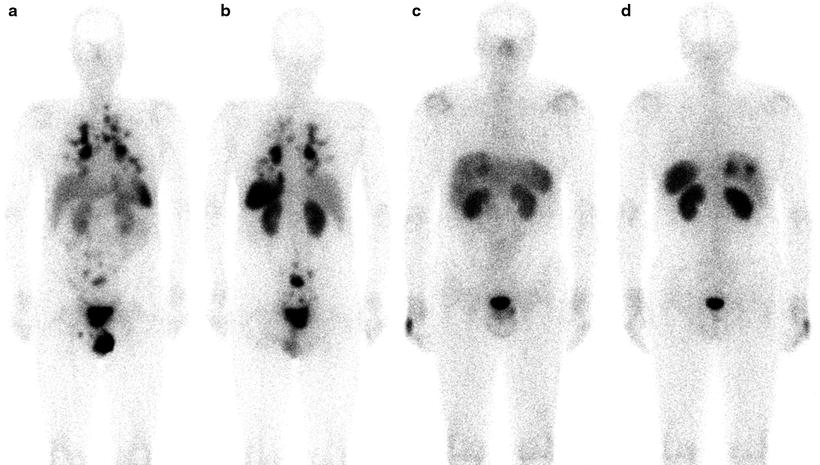
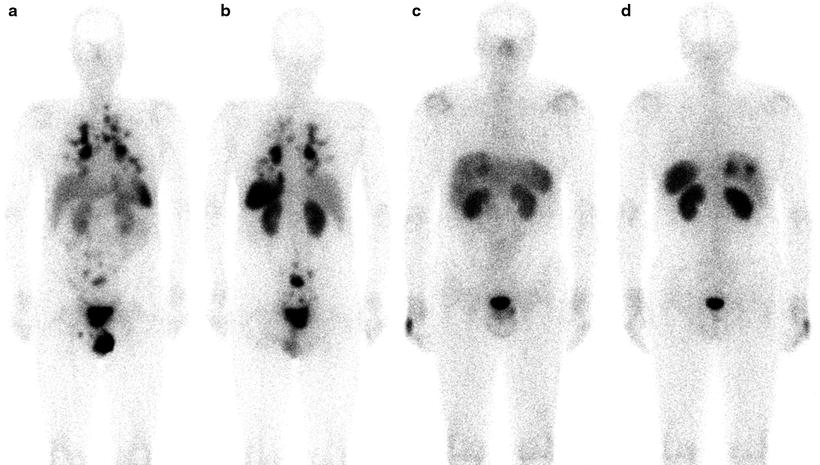
Fig. 18.4
SRS with 111In-pentetreotide. On the left, (a, b) a whole body scan shows a patient with an intestinal lesion, abdominal lymph nodes, and multiple lung metastases. On the right, (c, d) a patient with liver metastases
SRS with OctreoScan® may be used to select potentially responsive patients for treatment with the currently available octapeptide somatostatin analogs or with tumor-targeted radioactive treatment with radiolabeled (with 90Y or 177Lu) somatostatin analogs, since a high 111In-pentetreotide uptake is associated to a higher probability of response to radiolabeled somatostatin analogs [57, 58, 60–62]. The use of OctreoScan® scintigraphy to predict response to therapy is unclear since octapeptide somatostatin analogs [40, 63] may change SSTR expression. Similarly, it is unclear whether the sensitivity of SSTR imaging is reduced in patients concurrently receiving therapeutic doses of octreotide acetate, and consideration should be given to temporarily suspending therapy before the radiotracer administration.
In spite of these concerns, SRS with OctreoScan® is employed to monitor treatment efficacy. Changes in functional volume, assessed by scintigraphy, have been reported to be more useful than CT and RECIST (Response Evaluation Criteria in Solid Tumors) in monitoring tumor response after treatment and correlate well with the later clinical response [64] (Fig. 18.5).
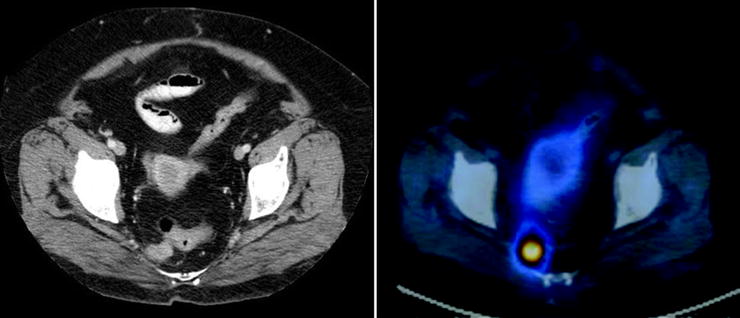
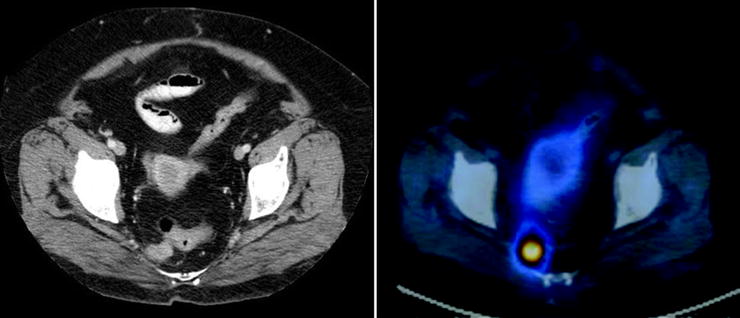
Fig. 18.5
SRS with 111In-pentetreotide. Peritoneal relapse of a GEP NET
The introduction of PET tracers including [11C]5-hydroxytryptophan, 18F-DOPA, and 68Ga-labeled octreotide increases the sensitivity of lesion detection and, with CT fusion imaging, provides an anatomic correlation to the areas of uptake [65–67].
In particular, somatostatin analogs labeled with 68Ga (produced by a generator, therefore not requiring an in-house cyclotron) offer the advantage of higher spatial resolution (about 4–5 mm) and signal-to-noise ratio and easier image quantification than SPECT [68, 69] (Fig. 18.6).
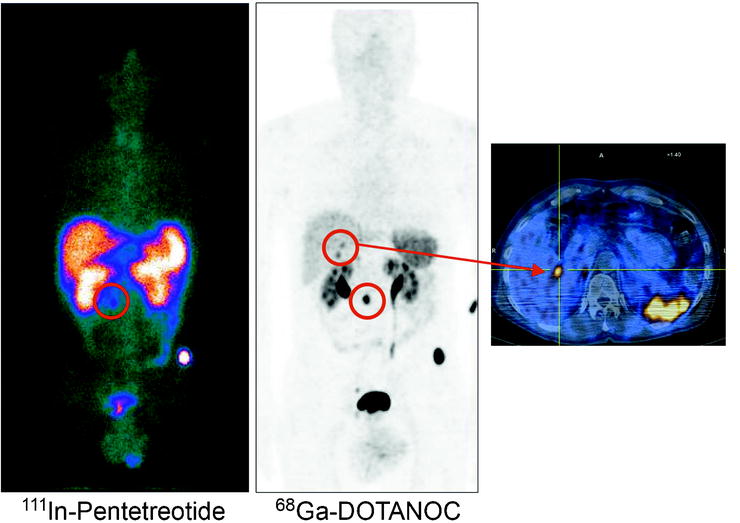
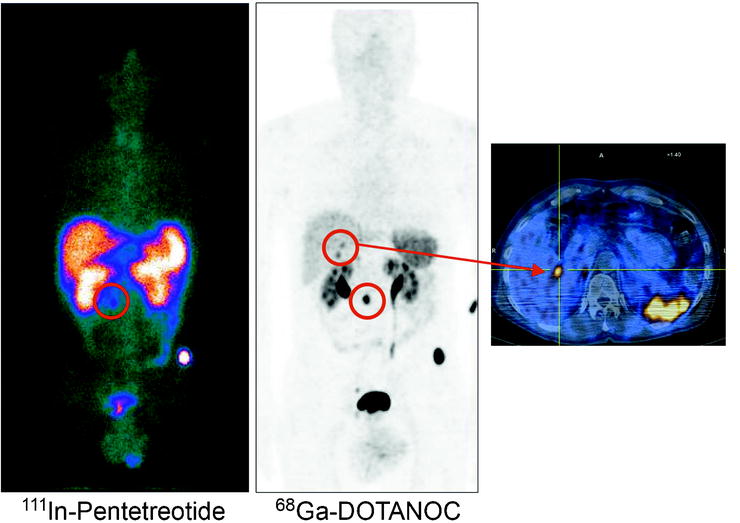
Fig. 18.6
Comparison between SRS with 111In-pentetreotide and PET with 68Ga-DOTANOC. The higher spatial resolution and signal-to-noise ratio of PET allow the identification of two small liver metastases which were not visualized by SRS
There are many different DOTA-octapeptides that can be labeled with 68Ga and used to image SSTR. The most commonly employed are 1,4,7,10-tetraazacyclododecane, 1,4,7,10-tetraacetic acid Tyr3 octreotide (DOTATOC), 1,4,7,10-tetraazacyclododecane,1,4,7,10-tetraacetic acid Thr8 octreotide (DOTATATE), and 1,4,7,10- tetraazacyclododecane, 1,4,7,10-tetraacetic acid 1-Nal3 octreotide (DOTA-NOC). Their affinity profiles for the somatostatin receptors are well known: ––TATE shows high binding affinity to sst2, while -TOC and –NOC recognize three SSTR subtypes (mainly sst2 and, with lower affinity, sst5 and sst3) [70, 71]. It is likely that 68Ga-DOTATOC and 68Ga-DOTATATE will become the new standard analogs since they can mimic as closely as possible the in vivo behavior of their 90Y- or 177Lu-labeled counterparts used for peptide receptor radionuclide therapy (PRRT) [62].
Although 68Ga-octreotide PET is still under evaluation, SSTR PET appears to be superior to CT and single-photon imaging for the diagnosis of NETs [68, 72]. 68Ga-DOTATOC PET also appears to be more accurate for the detection of bone metastasis than CT and conventional bone scintigraphy, with a sensitivity of 97% and a specificity of 92% [73]. A particular indication for the use of 68Ga-somatostatin PET/CT is for the detection of unknown primary NET (a recent work with DOTA-NOC showed 59% in detection rate compared to 39% of OctreoScan®), with the subsequent change in patients’ management (about 10%) [74] (Fig. 18.7).
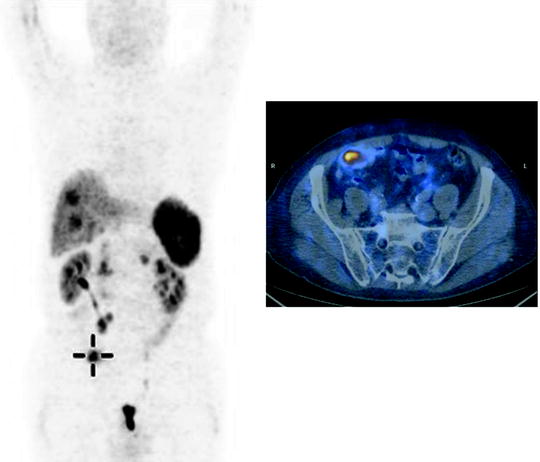
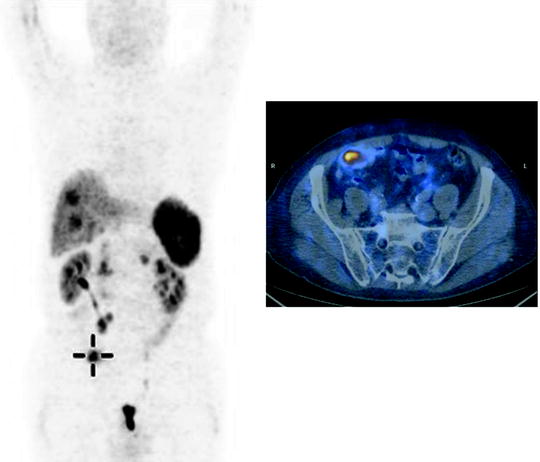
Fig. 18.7
Unknown primary NET with multiple liver and abdominal lymph node lesions. PET with 68Ga-DOTANOC shows a focal uptake in the ileum
Although there is no agreement about the use of 68Ga-somatostatin analogs in assessing response to somatostatin receptor radionuclide therapy [72], in many centers they are widely employed to estimate the intensity of uptake of the therapeutic counterparts and, therefore, to predict the response [75] to treatment.
Somatostatin analogs are more accurate than 123I-MIBG in detecting GEP NETs and their metastases. 123I-MIBG can be useful only when other methods have failed to depict NETs or when treatment with 131I-MIBG is being considered. The same results have been found about 18F-DOPA PET [76, 77].
Thoracic NET Imaging
Conventional Radiologic Imaging
Primary carcinoids of the lungs account for ∼10% of all NETs. Most carcinoids (typical and atypical) are located close to central bronchi, although in about 16–40% of cases, they are located in the peripheral lung. They typically show a spherical or ovoid shape with a well-defined border, but sometimes they develop along bronchi or pulmonary arteries. Punctate or diffuse calcifications are frequently observed on CT. Both typical and atypical carcinoids are usually hypervascular and demonstrate intense contrast enhancement (more than 30 HU). Atypical carcinoids are associated with hilar or mediastinal lymph node metastases. LCNEC, which is a poorly differentiated and high-grade NET, is morphologically between atypical carcinoid and SCLC. LCNEC and SCLC do not show any specific CT feature resulting similar to other common NSCLCs. However, CT plays a main role for staging and follow-up of all NETs of the lungs.
Nuclear Medicine Imaging
Molecular imaging can help differentiate the etiology of bronchial masses [81]. Since most of thoracic NETs express somatostatin receptors, especially subtype 2 and 5 (Fig. 18.8), 111In-pentetreotide scintigraphy is useful to detect most bronchial carcinoids >1 cm in diameter, including cases associated with ectopic GH-RH secretion [82, 83]. SRS is also helpful for radioguided surgery, allowing evaluation of the tumor bed after resection to detect any residual radioisotope uptake that corresponded to the presence of residual tumor [84, 85]. 68Ga-DOTATOC (–NOC or –TATE) PET/CT (Fig. 18.9) has the highest sensitivity with respect to mediastinal lymph node involvement and distant metastases.
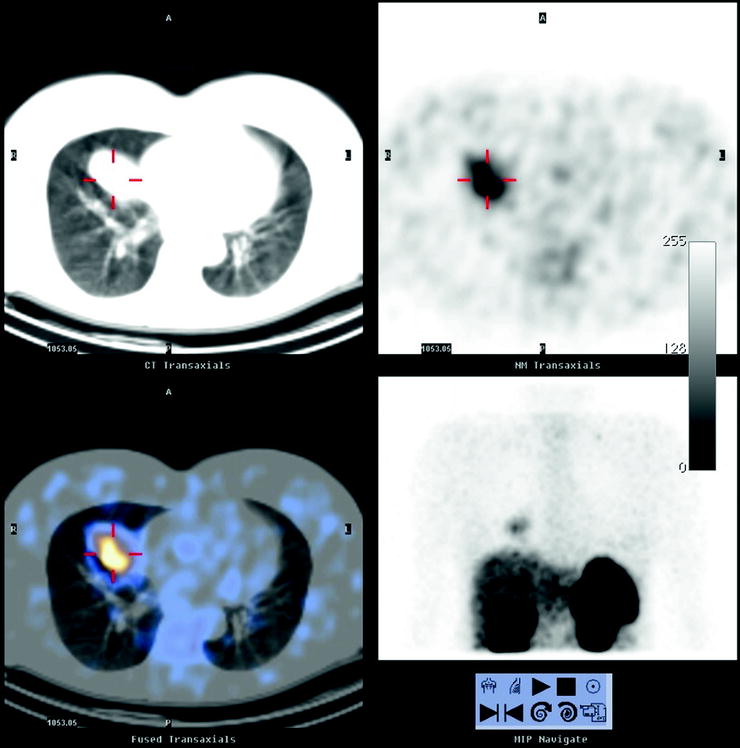
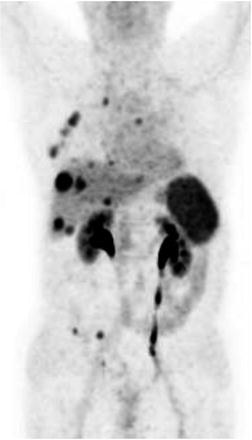
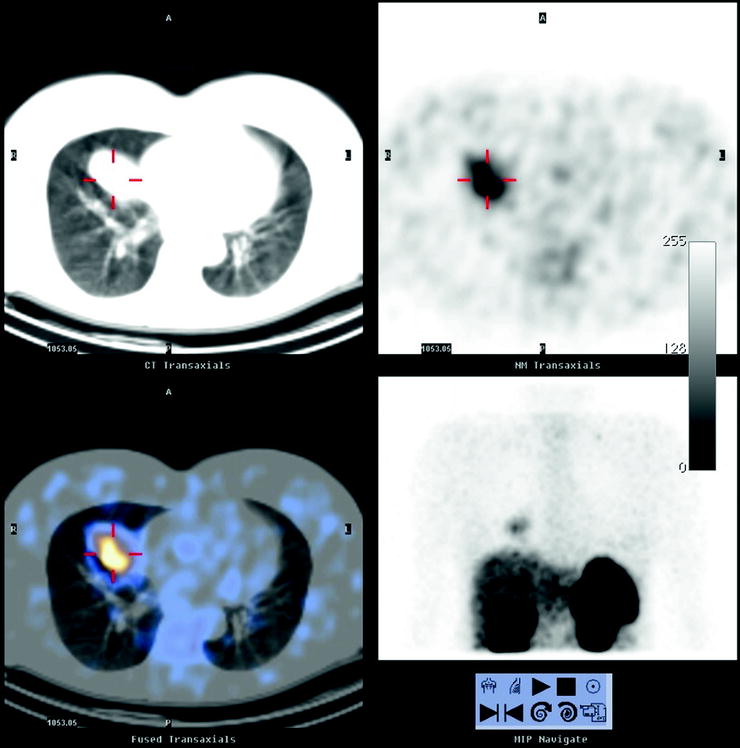
Fig. 18.8
Typical bronchial carcinoid. SRS with 111In-pentetreotide shows an intense uptake of the tracer within a mass in the medium lobe
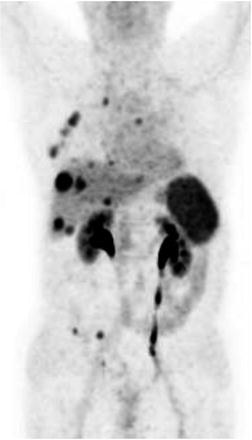
Fig. 18.9
PET with 68Ga-DOTANOC. Metastatic bronchial carcinoid
[18F]FDG PET/CT in NETs of the lungs shows variable [18F]FDG uptake according to tumor proliferation. A low [18F]FDG uptake (SUVmax < 2.5) is typical in bronchial carcinoids [86]. Tumors of a higher grade than typical bronchial carcinoids can have high [18F]FDG uptake. Atypical carcinoids can be more metabolically active and usually appear as a small pulmonary nodule with hilar or mediastinal lymph nodes showing high SUV. LCNECs had usually high [18F]FDG uptake, and PET/CT, as well as stand-alone CT, shows high accuracy in prediction of the presence of hilar and mediastinal nodal involvement. However, PET/CT seems to be better than CT alone in detecting distant metastases and leading to the changes in the clinical management. An SUVmax greater than 13.7 predicts a short survival period, suggesting the use of PET/CT with [18F]FDG not only in staging but also in predicting prognosis of LCNEC [87]. In SCLC, [18F]FDG PET is valuable for initial staging to distinguish localized vs. metastatic disease. Pandit et al. demonstrated that [18F]FDG PET/CT is also useful for prognosis, especially among treated patients [88].
Initial data about the combined use of [18F]FDG and 68Ga-DOTATOC have been reported [89]. Typical carcinoids, rich in SSRs, show high uptake on 68Ga-DOTATOC PET/CT imaging but low [18F]FDG uptake due to the low proliferative index. Increased avidity of [18F]FDG and/or decreased avidity for 68Ga-DOTATATE could identify aggressive tumors containing sites of possible dedifferentiation.
Imaging of Pheochromocytoma and Paragangliomas
Conventional Radiologic Imaging
CT and MRI are sensitive and specific for detecting adrenal pheochromocytomas (77–98% for CT and 95–100% for MRI). However, MRI and CT have a lower sensitivity (sensitivity of CT is 29%) for extra-adrenal lesions or metastases from malignant pheochromocytomas [90, 91]. In this setting specificity may be decreased since other tumors of neurogenic origin or mesodermal origin may resemble paragangliomas in both distribution and appearance [12, 92] (Figs. 18.10 and 18.11).
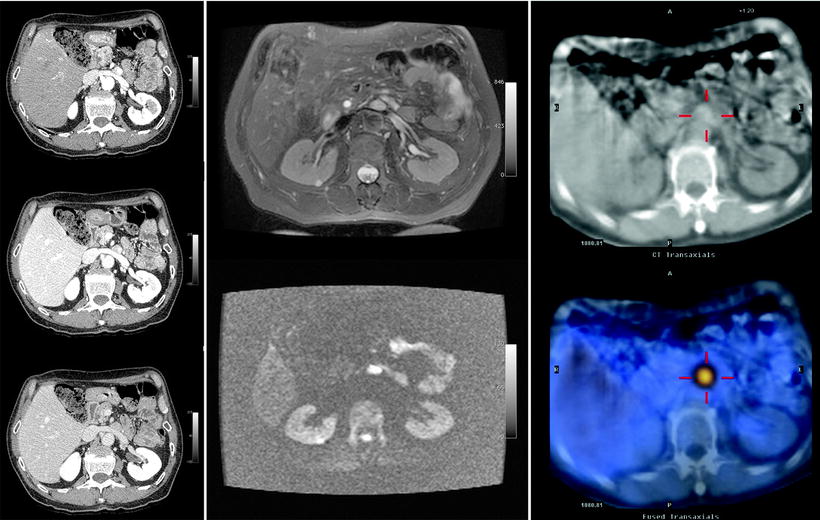
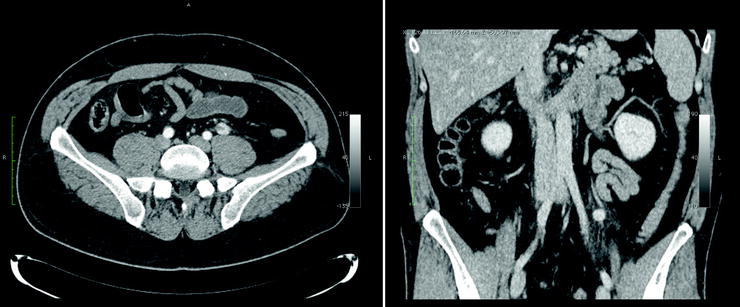
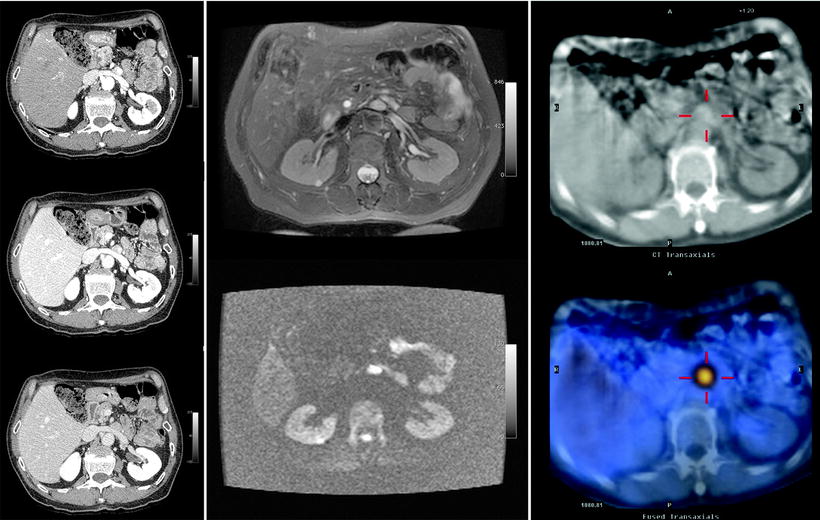
Fig. 18.10
Patient with an abdominal paraganglioma. The left images (from top to bottom) of a three-phase contrast-enhanced CT disclose a paraganglioma located in front of the left renal vein showing the typical contrast enhancement during the arterial phase (top). In the middle, MRI images show the same lesion hyperintense on T2-weighted image (top) and on DWI (bottom). The right images of a SPECT/CT study show the high uptake of 111In-pentetreotide within the paraganglioma
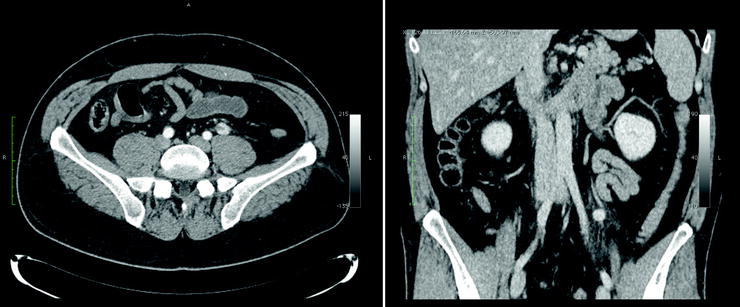
Fig. 18.11
CT shows a lesion with contrast enhancement during the arterial phase along the left ureter suggesting a diagnosis of paraganglioma. Subsequent SRS and 123I-MIBG scintigraphy were negative. After surgery, the lesion resulted to be a neurinoma at histopathology
Nuclear Medicine Imaging
Functional imaging with 123I-MIBG is the first choice among nuclear medicine methods [93–96]. 123I-MIBG scintigraphy is frequently used to confirm if the disclosed adrenal mass is indeed a pheochromocytoma (Fig. 18.12). 123I-MIBG scintigraphy has sensitivity and specificity of 90% and 95%, respectively. Moreover, the combined sensitivity of catecholamine measurements and 123I-MIBG scan approached 100% [97, 98]. In fact, a whole body scan 123I-MIBG is particularly helpful in the preoperative identification of multiple primary lesions (relatively frequent in norepinephrine-secreting pheochromocytomas) or metastases from malignant tumors (Fig. 18.13). Functional studies, such as 123I-MIBG, are also able to detect recurrences because the radiotracer accumulates specifically within the tumor and is not affected by postsurgical or postradiotherapy changes (Fig. 18.14).
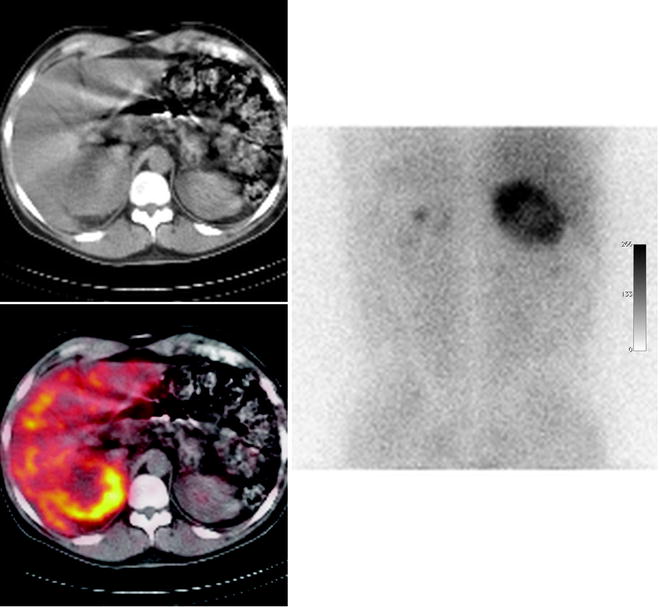
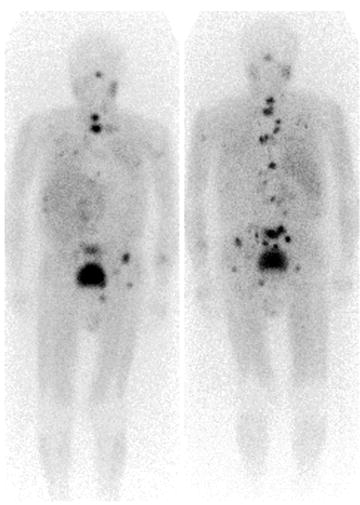
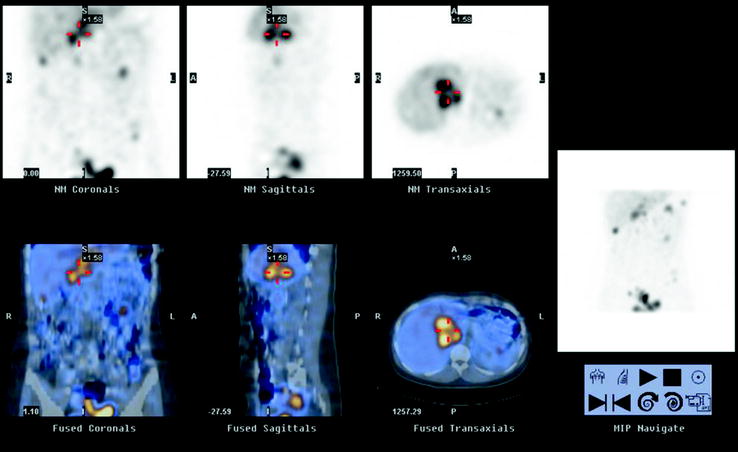
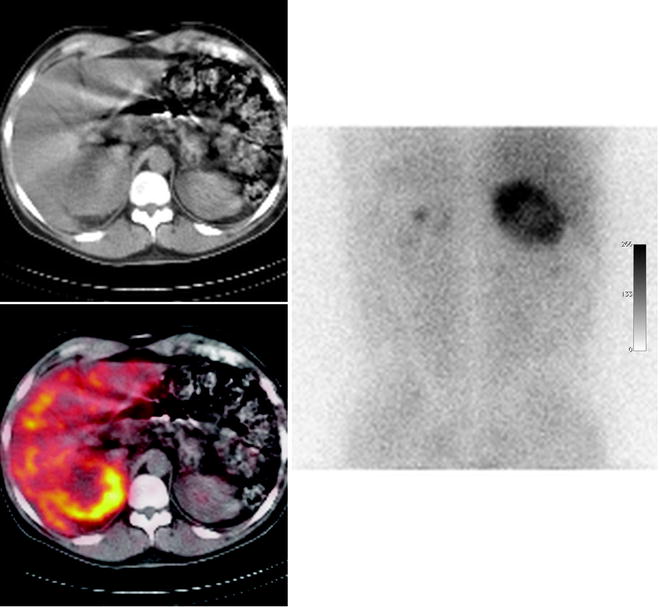
Fig. 18.12
123I-MIBG scintigraphy. The tracer uptake within a large tumor with central necrosis confirms the diagnosis of pheochromocytoma
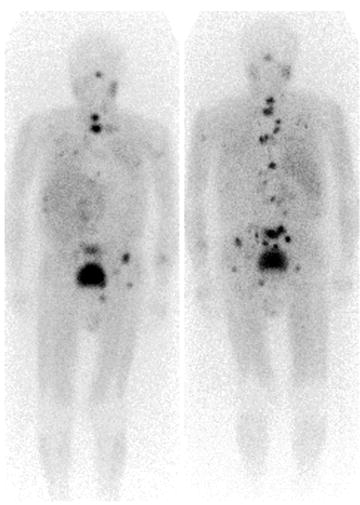
Fig. 18.13
123I-MIBG whole body scintigraphy. Multiple foci of tracer uptake indicating the presence of diffused bone metastases from a malignant pheochromocytoma
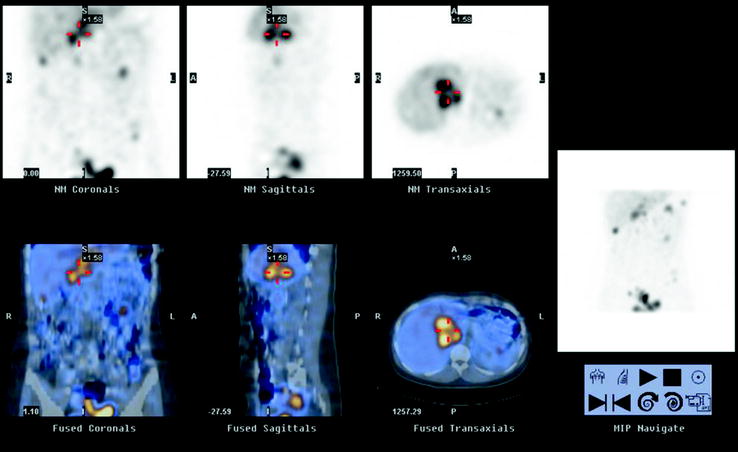
Fig. 18.14
Recurrence of adrenal pheochromocytoma. 123I-MIBG SPECT/CT demonstrates a large lesion close to the site of the previous left adrenal resection. Patient history shows a laparoscopic surgery complicated by intense hemorrhage within the pheochromocytoma, producing the tumor seeding into the whole abdominal cavity (multiple other lesion are clearly shown in the MIP image on the right)
Several factors can decrease the sensitivity of 123I-MIBG scintigraphy: (a) Some drugs interfere with MIBG uptake, resulting in false-negative results (Table 18.5). (b) False-negative scans can occur due to small lesion size or extra-adrenal location. 123I-MIBG imaging has a sensitivity of 58% for extra-adrenal tumors versus 85% for adrenal pheochromocytoma [99, 100]. Physiologic accumulation of MIBG in the urinary tract or bowel can be a source of false-positive results. 123I-MIBG SPECT evaluated with contrast CT or MRI or hybrid imaging with SPECT/CT allows more accurate anatomic localization of areas of uptake [101].
Table 18.5
Drugs that can decrease the sensitivity of 123I-MIBG scintigraphy
Drugs | Mechanism of interference (known or expected) | Suggested withdrawal prior to 123I-MIBG scintigraphy |
---|---|---|
Opioids, cocaine, tramadol | Uptake inhibition | 7–14 days |
Tricyclic antidepressants | Uptake inhibition | 7–21 days |
Sympathicomimetics | Depletion of granules | 7–14 days |
Antihypertensive/cardiovascular agents | ||
Labetalol, metoprolol, amiodarone | Inhibition uptake and depletion | 21 days |
Reserpine, bretylium, guanethidine | Depletion and transport inhibition | 14 days |
Calcium channel blockers and ACEI | Increased uptake and retention | |
Antipsychotics | ||
Phenothiazines, thioxanthenes | Uptake inhibition | 21–28 days |
Butyrophenones |
123I-MIBG imaging is not very useful for the detection of detection of paragangliomas (17–42%) developing in the head and neck region. While paragangliomas of abdomen and thorax can be functionally active, head and neck paragangliomas rarely are hormonally active and produce an excess of catecholamine secretion. Actually, these nonfunctioning paragangliomas are formerly referred to glomic tumors [102, 103]. In those settings where scintigraphic results could be affected by a lack of sensitivity of 123I-MIBG (size and site of the lesions; extra-adrenal, familial, and malignant pheochromocytomas), other radiopharmaceuticals should be considered [104, 105].
Therapy
Treatment of NETs is typically multidisciplinary and should be individualized according to the tumor histology, lesion extent, and symptoms. Surgery is useful to remove the primary and debulk metastatic lesions. A limitation of surgery is the frequent presence of metastatic disease. Surgery can be curative in only 20% of cases [106].
Tumors located in the head of pancreas or in the duodenum are treated with the Whipple pancreaticoduodenectomy procedure, while ileal carcinoids are usually treated with ileal resection and right hemicolectomy [107]. In bronchial carcinoids, lung resection surgery, including lymphadenectomy, is the option of choice. For the more aggressive categories of thoracic NETs (LCNEC and SCLC), surgery is seldom feasible, and the outcomes are quite poor [108].
Liver transplantation for GEP NETs remains controversial and can be proposed in selected situations when the liver is the sole site of disease, usually in patients with stable metastases from intestinal NETs and low Ki67 index [109].
The definitive treatment for pheochromocytoma/paraganglioma is surgical. Laparoscopic removal of intra-adrenal and extra-adrenal pheochromocytomas is now the surgical technique of choice [110]. Operative mortality is less than 1%, if adequate preparation with hormone blocking is carried out in patients with catecholamine-secreting tumors [111, 112]. Cortex-sparing adrenalectomy can be performed in hereditary, frequently bilateral, tumors, while malignant and diffusely spread tumors must be treated with conventional transabdominal resection.
Interventional radiology techniques are helpful to treat lesions in the liver. Liver metastases from NETs are typically hypervascular, and (chemo)embolization of the hepatic artery, performed mechanically by microspheres or chemically with cytotoxic agents, can lead to significant necrosis. Recently, radioembolization of liver metastases with 90Y-labeled microspheres has been tested in several clinical trials with excellent preliminary results [113, 114]. Other techniques still to be validated in NETs include “umbrella” radio-frequency ablation and the newest high-intensity focused ultrasound (HIFU) ablation [115, 116].
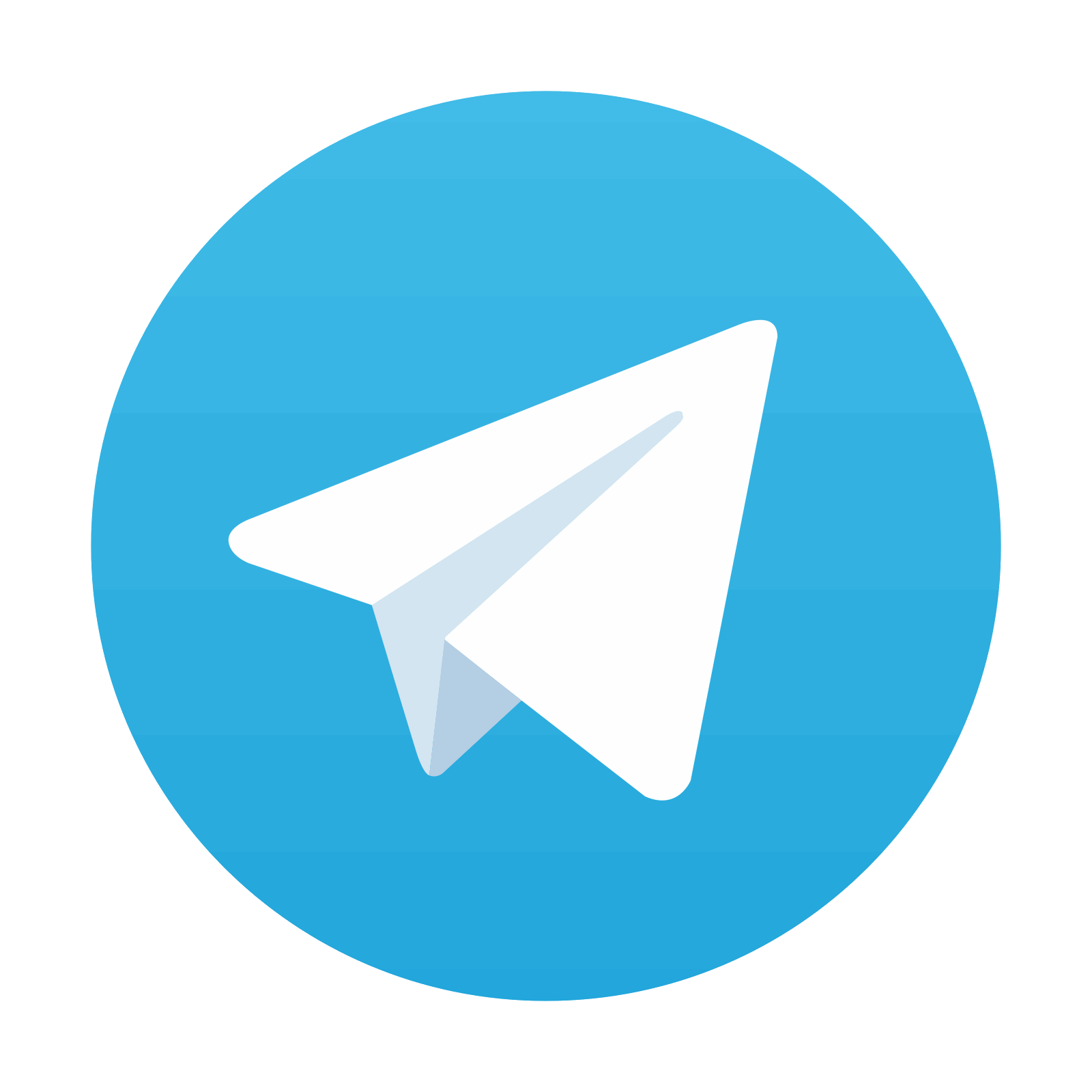
Stay updated, free articles. Join our Telegram channel

Full access? Get Clinical Tree
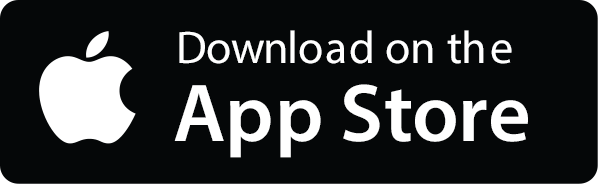
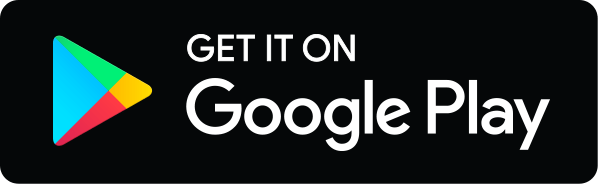