Neuroimaging technologies provide a powerful approach to exploring the genetic basis of individual differences in complex behaviors and vulnerability to neuropsychiatric illness. Functional MRI studies have established important physiologic links between genetic polymorphisms and robust differences in information processing within distinct brain regions and circuits that have been linked to the manifestation of various disease. Neuroimaging technologies represent a critical tool in efforts to understand the neurobiology of normal and pathologic behavioral states. Research capitalizing on neuroimagingbased integration will contribute to the identification of predictive markers and biologic pathways for neuropsychiatric disease vulnerability and the generation of novel targets for therapeutic intervention.
Conceptual basis and overview
Genes have an unparalleled potential impact on all levels of biology. In the context of disease states, in particular behavioral disorders, genes represent the cornerstone of mechanisms that, either directly or in concert with environmental events, ultimately result in disease. Moreover, genes offer the potential to identify at-risk individuals and biologic pathways for the development of new treatments. Although most human behaviors cannot be explained by genes alone, and much variance in aspects of brain structure and function will not be genetically determined directly, it is anticipated that variations in genetic sequence having an impact on function will contribute an appreciable amount of variance to these resultant complex biologic and behavioral phenomena. This conclusion is implicit in the results of studies of twins that have revealed heritabilities ranging from 40% to 70% for various aspects of cognition, temperament, and personality . In the case of psychiatric illness, genes seem to be the only consistent risk factor identified across populations, and the majority of susceptibility for major psychiatric disorders is accounted for by inheritance .
Traditionally, the impact of genetic polymorphisms on human behavior has been examined directly using clinical evaluation, personality questionnaires, and neuropsychologic batteries. Genetic epidemiologic investigations have examined directly the relationship between specific genetic polymorphisms and behaviors and reported equivocal results. This is not surprising for at least two reasons. First, there is considerable individual variability in dimensions of observable behavior and subjectivity in the assessment of behavior, necessitating large samples that often require an excess of several hundred subjects to identify even small gene effects. Moreover, it is apparent that there are etiologic subgroups within any given disease that obscure effects at the broader group level. Second, and perhaps most importantly, the effects of genes are not expressed directly at the level of behavior. As discussed in detail later, gene effects on behavior are mediated by their molecular and cellular effects on information processing in the brain. Thus, examining the effects of genes on the brain represents a critical step in understanding their ultimate contribution to variability in behavior.
Because genes are involved directly in the development and function of brain regions subserving specific cognitive and emotional processes, functional polymorphisms in genes may be related strongly to the function of these specific neural systems and, in turn, mediate their involvement in behavioral outcomes. This is the underlying assumption of the authors’ investigations examining the relation between genes and neural systems, initially called, “imaging genomics” and described more recently as “imaging genetics” , because it typically is used to explore variation in specific genes and not the genome broadly. The potential for marked differences at the neurobiologic level underscores the need for a direct assay of brain function. Accordingly, imaging genetics within the context of a “candidate gene association approach” provides an ideal opportunity to further understanding of biologic mechanisms potentially contributing to individual differences in behavior and personality. Moreover, imaging genetics provides a unique tool with which to explore and evaluate the functional impact of brain-relevant genetic polymorphisms with the potential to understand their impact on behavior. The relevance of the findings of imaging genetics studies for disease vulnerability will be made only once the variants under study are associated further with disease risk directly or if their impact on brain function is manifest, or even exaggerated, in the diseases of interest.
Neuroimaging techniques, especially those that are noninvasive, such as MRI, electroencephalography, and magnetoencephalography, typically require no more than a few minutes of subject participation to acquire substantial data sets, reflecting the acquisition of many hundreds of repeated measures of brain structure or function within a single subject. The efficiency of these techniques allows for the ability to investigate the specificity of gene effects by examining their influence on multiple functional systems (eg, prefrontal, striatal, and limbic) in a single subject in one experimental session. This capacity to assay the brain rapidly with power and sensitivity places neuroimaging at the forefront of available tools for the in vivo study of functional genetic variation.
Imaging genetics: basic principles
Selection of candidate genes
The protocol for imaging genetics typically involves first identifying a meaningful variation in the DNA sequence within a candidate gene. For the variant to be meaningful, it should have an impact on function at the molecular or cellular level (ie, be a functional variant) and the distribution of such effects at the level of brain systems should be predictable. Short of well-defined functional polymorphisms, candidate genes that have identified single nucleotide polymorphisms (SNPs) or other allele variants in coding or promoter regions with likely functional implications (eg, nonconservative amino acid substitution or missense mutation in a promoter consensus sequence) involving circumscribed neuroanatomic systems are attractive substrates. Recent imaging genetics studies have taken the lead in exploring the functionality of candidate variants by first describing their in vivo effects at the level of brain systems .
Consistent with the goal of genetic association studies to identify variation of impacts on individual differences in behavior and related risk for disease in the general population, candidate polymorphisms in imaging genetics studies also should be common. That is, the relative frequency of the less common or minor allele should be greater than 5%. In light of the considerable costs of neuroimaging in comparison with behavioral studies and the related sample size limitations, the minor allele frequency of candidate polymorphisms in imaging genetics studies ideally should be greater than 30%. In such cases, the relative contributions of all potential genotypes (ie, homozygotes for major and minor alleles and heterozygotes) to brain function can be examined using imaging genetics.
Control for nongenetic factors
The contribution of single genes to the structural and functional integrity of brain systems, although putatively more substantial than that to emergent behavioral phenomena, still is presumably small. Furthermore, typically large effects of age, gender, IQ, and environmental factors, such as illness, trauma, or substance abuse, on phenotypic variance easily can obscure these small potential gene effects. Because association studies in imaging genetics are susceptible to population stratification artifacts (ie, differing ancestral genetic backgrounds for specific candidate genotypes), as in any case-control association study, ethnic matching within genotype groups and genomic control also potentially is critical . Thus, the identification and contribution of genetic variation to specific phenotypes should be limited to studies in which other potential contributing and confounding factors are matched carefully across genotype groups. If the imaging protocol involves performance of a task, as is typical in functional MRI (fMRI), the groups also should be matched for level of performance, or at least any variability in performance should be considered in the analysis and interpretation of the imaging data. This is because task performance and the fMRI blood oxygenation level–dependent signal are linked tightly, and systematic differences in performance between genotype groups could obscure or masquerade a true gene effect.
Task selection
There has been a tremendous proliferation of functional neuroimaging studies accompanied by behavioral tasks designed specifically for this experimental setting. Many of these are modified versions of classic behavioral and neuropsychologic tests (eg, the Wisconsin Card Sorting Task) designed to tap neural systems critical to particular behaviors. More recent paradigms have emerged that focus on interactions of specific behaviors and disease states as these questions have become newly accessible with noninvasive imaging (eg, the emotion Stoop task and obsessive-compulsive disorder).
Because of the small effects of single genes, even after controlling for nongenetic and other confounding variables, imaging tasks must maximize sensitivity and inferential value. As the interpretation of potential gene effects depends on the validity of the information processing paradigm, it is best to select well-characterized paradigms that are effective at engaging circumscribed brain regions and systems, produce robust signals in every individual, and show variance across individuals. Imaging genetics studies typically are not the appropriate venue to design and test new functional tasks, and to do so might undermine their tremendous potential.
Imaging genetics: basic principles
Selection of candidate genes
The protocol for imaging genetics typically involves first identifying a meaningful variation in the DNA sequence within a candidate gene. For the variant to be meaningful, it should have an impact on function at the molecular or cellular level (ie, be a functional variant) and the distribution of such effects at the level of brain systems should be predictable. Short of well-defined functional polymorphisms, candidate genes that have identified single nucleotide polymorphisms (SNPs) or other allele variants in coding or promoter regions with likely functional implications (eg, nonconservative amino acid substitution or missense mutation in a promoter consensus sequence) involving circumscribed neuroanatomic systems are attractive substrates. Recent imaging genetics studies have taken the lead in exploring the functionality of candidate variants by first describing their in vivo effects at the level of brain systems .
Consistent with the goal of genetic association studies to identify variation of impacts on individual differences in behavior and related risk for disease in the general population, candidate polymorphisms in imaging genetics studies also should be common. That is, the relative frequency of the less common or minor allele should be greater than 5%. In light of the considerable costs of neuroimaging in comparison with behavioral studies and the related sample size limitations, the minor allele frequency of candidate polymorphisms in imaging genetics studies ideally should be greater than 30%. In such cases, the relative contributions of all potential genotypes (ie, homozygotes for major and minor alleles and heterozygotes) to brain function can be examined using imaging genetics.
Control for nongenetic factors
The contribution of single genes to the structural and functional integrity of brain systems, although putatively more substantial than that to emergent behavioral phenomena, still is presumably small. Furthermore, typically large effects of age, gender, IQ, and environmental factors, such as illness, trauma, or substance abuse, on phenotypic variance easily can obscure these small potential gene effects. Because association studies in imaging genetics are susceptible to population stratification artifacts (ie, differing ancestral genetic backgrounds for specific candidate genotypes), as in any case-control association study, ethnic matching within genotype groups and genomic control also potentially is critical . Thus, the identification and contribution of genetic variation to specific phenotypes should be limited to studies in which other potential contributing and confounding factors are matched carefully across genotype groups. If the imaging protocol involves performance of a task, as is typical in functional MRI (fMRI), the groups also should be matched for level of performance, or at least any variability in performance should be considered in the analysis and interpretation of the imaging data. This is because task performance and the fMRI blood oxygenation level–dependent signal are linked tightly, and systematic differences in performance between genotype groups could obscure or masquerade a true gene effect.
Task selection
There has been a tremendous proliferation of functional neuroimaging studies accompanied by behavioral tasks designed specifically for this experimental setting. Many of these are modified versions of classic behavioral and neuropsychologic tests (eg, the Wisconsin Card Sorting Task) designed to tap neural systems critical to particular behaviors. More recent paradigms have emerged that focus on interactions of specific behaviors and disease states as these questions have become newly accessible with noninvasive imaging (eg, the emotion Stoop task and obsessive-compulsive disorder).
Because of the small effects of single genes, even after controlling for nongenetic and other confounding variables, imaging tasks must maximize sensitivity and inferential value. As the interpretation of potential gene effects depends on the validity of the information processing paradigm, it is best to select well-characterized paradigms that are effective at engaging circumscribed brain regions and systems, produce robust signals in every individual, and show variance across individuals. Imaging genetics studies typically are not the appropriate venue to design and test new functional tasks, and to do so might undermine their tremendous potential.
Imaging genetics: applications of the principles
The following sections provide introductory examples of how imaging genetics can lead to insights about the biologic mechanisms underlying individual differences in complex behavioral traits. In many cases, the genetically driven variability in behavior represents an intermediate phenotype conferring risk for neuropsychiatric disease in the context of specific environmental influences. Exemplars include episodic memory, executive function, temperamental anxiety, and their respective neural underpinnings, namely the hippocampus, prefrontal cortex, and amygdala. In each group of studies, neuroimaging was used to identify the effects of genetic polymorphisms, often resulting in molecular and cellular alterations and those associated with specific behaviors or disease states, on discrete neural circuitries supporting the associated behavioral and clinical phenomena. In addition, each study incorporated the basic principles of imaging genetics (described previously), such as the implementation of rigorous controls for nongenetic factors, such as age, gender, population stratification, and performance on the experimental task. All of the studies capitalized on existing functional paradigms designed to explore physiologic aspects of distinct neural systems.
Common polymorphisms having an impact on the hippocampus and episodic memory
Brain-derived neurotrophic factor
Brain-derived neurotrophic factor (BDNF) is well known as playing a role in neuronal survival, differentiation, and synaptic plasticity. BDNF is highly expressed in the hippocampus, a brain region critical for episodic memory. A common SNP in the human BDNF gene, a valine (Val) to methionine (Met) substitution at codon 66 (Val66Met), is associated with abnormal intracellular trafficking and regulated secretion of pro-BDNF, which is the precursor to functional BDNF . Thus, the BDNF Met66 allele may have an impact on learning and memory supported by the actions of BDNF on local neuronal plasticity and synaptic reorganization. Consistent with the molecular effects of the Met66 allele, studies have documented impaired episodic memory in human subjects carrying the Met66 allele . Converging evidence from several neuroimaging studies using different modalities suggests further that the Met66 effects on memory are mediated in part by its impact on hippocampal structure and function. Magnetic resonance spectroscopy has revealed Met66 allele-associated reductions in hippocampal N-acetyl aspartate, an intraneuronal metabolite correlated closely with glutamate neurotransmission and neuronal integrity . Based on these findings, fMRI was used to study the effects of the BDNF Val66Met on hippocampal activation in healthy volunteers performing a simple declarative memory task. Consistent with the behavioral and NAA findings, Met66 allele carriers showed reduced hippocampal activation during encoding and retrieval stages of episodic memory . A subsequent study found that Met66 allele carriers also exhibited a 12% to 15% reduction in hippocampal volume . These collective neuroimaging studies demonstrate a remarkable consistency in the effects of the BDNF Met66 allele on hippocampal structure and function, thereby providing a detailed account of the underlying mechanisms contributing to the effects of the polymorphism on learning and memory.
Apolipoprotein
Apolipoprotein E (APOE) is essential for multiple brain functions, including neuronal growth and repair, neuroprotection, and inflammation. A common allelic variant of the human APOE gene is associated with late-onset familial Alzheimer’s disease . Specifically, the APOE ε4 allele has a dose-dependent effect on risk and age of onset for the disease . Positron emission tomography (PET) studies report deficits in resting cortical glucose metabolism in cognitively normal middle-aged subjects who have the APOE ε4 allele . In the first application of fMRI in imaging genetics, subjects carrying the APOE ε4 allele exhibited significantly greater activation in memory-related brain regions, such as the prefrontal cortex and hippocampus, during a challenging memory task in comparison to those homozygous for the APOE ε3 allele, which is not associated with increased risk for Alzheimer’s disease. In contrast to the strikingly different pattern of brain activation between the two groups, all subjects were cognitively intact and performed the memory task equally well. Such relatively increased neural activity in those who had the at-risk allele was interpreted as reflecting possible compensatory phenomena through the recruitment of additional cognitive resources in the face of greater task difficulty and demand. The magnitude of task-related brain activity was correlated significantly with subsequent memory decline. These data suggest that changes in brain information processing during declarative memory are associated with the biologic effects of APOE ε4, even if compensation is made at the level of observable behavior, and that such memory-related effects may provide a useful tool for predicting the course of cognitive decline.
KIBRA
A recent study found that a genomic locus encoding the newly discovered brain protein, KIBRA, is associated significantly with normal variability in memory performance . More specifically, a common thymine (T) to cytosine substitution within intron 9 of the human KIBRA gene is associated significantly with differential memory performance. Carriers of the KIBRA T allele exhibit greater free recall of words after short and long delay periods and better memory scores in tests of episodic memory. Molecular and cellular studies reveal that the KIBRA is highly expressed in brain regions involved in memory (eg, hippocampus). In addition, the polymorphism exists in a region that may be critical in allowing KIBRA to interact with other systems to regulate synaptic plasticity, such as that associated with learning and memory. Based on these findings, fMRI was used to explore the impact of the KIBRA T allele on memory-related brain activity. During retrieval of paired associates, noncarriers of the T allele showed significantly increased brain activation compared with T allele carriers in the hippocampus and the medial frontal gyrus and parietal cortex. As memory performance did not differ between these groups, the data suggest that noncarriers of the T allele need greater activation in these memory-related brain regions to reach the same level of retrieval performance as T allele carriers. Thus, the potential impact of the KIBRA T allele on synaptic plasticity may manifest as more efficient processing of information in memory-related neural circuits and subsequently superior memory ability.
Common polymorphisms having an impact on the prefrontal cortex and executive function
Catechol-o-methyltransferase
Because dopamine transporters virtually are absent at cortical synapses, dopamine regulation in the prefrontal cortex is coupled uniquely to inactivation mechanisms in postsynaptic neurons and glia . Catechol-o-methyltransferase (COMT), a methylation enzyme that converts released dopamine to inactive 3-methoxytyramine, is believed to play an important role in the inactivation of prefrontal dopamine . A common polymorphism (Val158Met) in the COMT gene affects enzyme activity, with the thermolabile Met allele having one fourth the activity of the thermostable Val allele. Thus, the COMT Val158Met polymorphism may have an impact on dopamine-regulated prefrontal cortical activity during executive and working memory tasks that tax this functional circuitry and are affected by variations in dopamine signaling . This polymorphism is linked to impairments in executive function and working memory in Val158 carriers , suggesting that genetically driven alterations in COMT enzymatic activity and, subsequently, in synaptic prefrontal dopamine concentrations may lead to diminished prefrontal function. fMRI reveals that the load of the high-activity Val158 allele consistently predicts an exaggerated prefrontal response during the performance of a well-characterized working memory test . These imaging genetics findings are interpreted to reflect an inefficient and, thus, exaggerated response, perhaps in an effort to maintain task performance or as a reflection of diminished prefrontal signal-to-noise ratio resulting from decreased concentrations of prefrontal dopamine associated with the high-activity Val158 allele. Because schizophrenia involves abnormal prefrontal function, the COMT Val158, especially in the context of environmental triggers, such as drug abuse , may reflect an important genetic mechanism contributing to this aspect of the disease.
Glutamate receptor 3
The metabotropic glutamate receptor 3 (GRM3), one of a class of receptors modulating synaptic levels of the excitatory neurotransmitter glutamate, is a promising schizophrenia susceptibility candidate gene. A common GRM3 haplotype was associated strongly with schizophrenia and within this haplotype, the A allele of SNP4 in intron 2 was overtransmitted slightly to probands and is associated with poorer performance on several cognitive tests of prefrontal and hippocampal function in volunteers and patients. The effects of this SNP on neurobiologic traits related to risk for schizophrenia and glutamate neurotransmission recently were examined using imaging genetics . fMRI showed deleterious activation patterns in the prefrontal cortex and hippocampus in control subjects homozygous for the SNP4 A allele. The investigators provided molecular data suggesting the SNP4 A allele alters prefrontal mRNA and protein levels of GRM3 and glutamate transporter, which is regulated by GMR3. Collectively, their data suggest that genetic variation in GMR3 affecting glutamate neurotransmission has an impact on prefrontal and hippocampal physiology and, in turn, related cognitive and mnemonic processes, which may be impaired in schizophrenia.
Disrupted-in-schizophrenia 1
As its name implies, disrupted-in-schizophrenia 1 (DISC1), which is expressed predominantly within the hippocampus, is another schizophrenia susceptibility candidate gene. Recently, a three-SNP haplotype of DISC1 was associated with schizophrenia in a family-based sample . A common nonsynonymous SNP resulting in a serine (Ser) to cytosine (Cys) substitution at codon 704 (Ser704Cys) within this haplotype was associated specifically with schizophrenia. In addition to overtransmission in schizophrenia, the Ser704 allele was associated with altered hippocampal structure and function in healthy subjects, including reduced hippocampal gray matter volume and altered engagement of the hippocampus during several cognitive tasks assayed with fMRI . These convergent data suggest that allelic variation within DISC1, at Ser704Cys or haplotypes monitored by it, increases the risk for schizophrenia and that the mechanism of this effect involves structural and functional alterations of the hippocampus.
Common polymorphisms having an impact on the amygdala and temperamental anxiety
Serotonin transporter gene
Individual differences in serotonin function repeatedly and consistently are associated with variability in affect and temperament in mice, monkeys, and humans . Moreover, abnormal serotonin neurotransmission is implicated in the pathophysiology of mood and anxiety disorders, and serotonin substrates are a key target of drugs used to treat these disorders. A common polymorphism in the promoter region (5-HTTLPR) of the serotonin transporter gene (5-HTT) is the most studied of genetic variants having an impact on serotonin neurotransmission. Such interest is, in part, mediated by the critical role of the 5-HTT in regulating serotonin signaling at pre- and postsynaptic receptors (via active clearance of released serotonin from the synapse) and the widespread use of antidepressant drugs that selectively block this reuptake mechanism. In comparison to the 5-HTTLPR long allele, the short (S) allele is associated with reductions in 5-HTT expression and serotonin reuptake in vitro . Although this in vitro effect initially was confirmed using in vivo single photon emission CT , recent PET studies offering more specific radiotracers and improved spatial resolution have failed to find altered 5-HTT levels associated with the 5-HTTLPR . Rather, effects of the 5-HTTLPR are documented in other serotonin subsystems, notably the 5-HT 1A receptor , and such downstream effects may be critical in mediating the neural and behavioral effects of the 5-HTTLPR .
At the behavioral level, possession of one or two copies of the S allele is associated with increased levels of temperamental anxiety , conditioned fear responses , and the development of depression , especially in the context of environmental stress . fMRI studies have provided a unique understanding of how the 5-HTTLPR may have an impact on temperamental anxiety and risk for depression. In a landmark study, fMRI revealed that the reactivity of the amygdala, a brain region critical in mediating emotional arousal, to threat-related facial expressions was exaggerated significantly in S allele carriers . Since this original study, there have been many replications of the association between the S allele and increased amygdala reactivity in healthy volunteers and patients who have mood disorders . In addition, the 5-HTTLPR S allele is linked further with reduced gray matter volumes in and functional coupling between the amygdala and medial prefrontal cortex . As the magnitude of amygdala reactivity (and its functional coupling with medial prefrontal cortex) is associated with temperamental anxiety, these imaging genetics findings suggest that the 5-HTTLPR S allele may be associated with increased risk for depression on exposure to environmental stressors because of its mediation of exaggerated corticolimbic reactivity to potential threat.
Monoamine oxidase A
Serotonin neurotransmission also is regulated through intracellular degradation via the metabolic enzyme, monoamine oxidase A (MAOA). A common genetic polymorphism in the MAOA gene, resulting in a low-activity enzyme, is associated with increased risk for violent or antisocial behavior. A recent fMRI study reported that the low-activity MAOA allele is associated with exaggerated amygdala reactivity and diminished prefrontal regulation of the amygdala . The magnitude of functional coupling between these regions predicted levels of temperamental anxiety, suggesting that the genetic association between the MAOA low-activity variant and abnormal behavior may be mediated through this circuit. The 5-HTTLPR S and MAOA low-activity alleles presumably result in increased serotonin signaling and exaggerated amygdala reactivity. As the directionality of these effects is consistent with animal studies documenting anxiogenic effects of serotonin , the imaging genetics data provide important insight regarding the neurobiologic and behavioral effects of serotonin.
Tryptophan hydroxylase 2
Recent imaging genetics studies examining the impact of variation on serotonin subsystems highlight the potential reciprocal nature by which functional imaging and molecular genetics approaches can be mutually informative in advancing understanding of the biologic mechanism of behavior. Tryptophan hydroxylase 2 (TPH2) is the rate-limiting enzyme in the synthesis of neuronal serotonin and, thus, plays a key role in regulating serotonin neurotransmission. A recent study found that a SNP in the regulatory region of the human TPH2 gene affects amygdala function . Specifically, the T allele of the relatively frequent G(-844)T polymorphism was associated with exaggerated amygdala reactivity. This report provides further insight into the biologic significance of TPH2 in the human central nervous system and offers a critical next step in the understanding of the importance of this newly identified second tryptophan hydroxylase isoform for human brain function. Moreover, it marks an important advance in the application of functional neuroimaging to the study of genes, brain, and behavior. In contrast to previous studies of genetic effects on brain function, where the molecular and cellular effects of the candidate variants previously was demonstrated (eg, 5-HTTLPR, MAOA, COMT, and BDNF), these fMRI data provide the first evidence of potential functionality of a novel candidate polymorphism. In this way, the initial identification of a systems-level effect of a specific polymorphism provides impetus for the subsequent characterization of its functional effects at the molecular and cellular level. Building on this initial imaging genetics finding (and a subsequent replication) , a recent molecular study demonstrated that the G(-844)T is in strong linkage with another promoter SNP that has an impact on transcriptional regulation of TPH2 and may affect enzyme availability and serotonin biosynthesis. Such scientific reciprocity between imaging and molecular genetics illustrates how the contributions of abnormalities in candidate neural systems to complex behaviors and emergent phenomena, possibly including psychiatric illnesses, can be understood from the perspective of their neurobiologic origins.
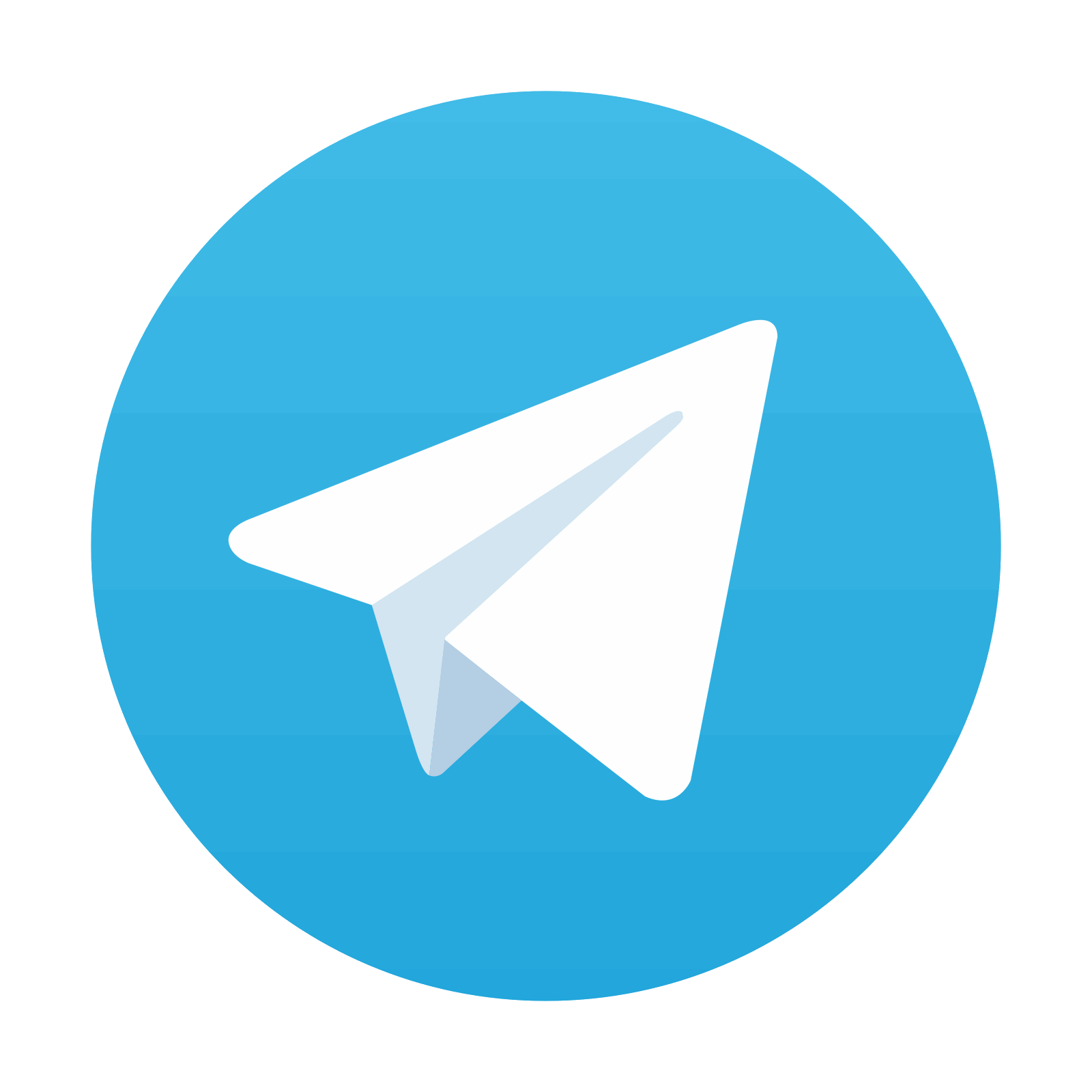
Stay updated, free articles. Join our Telegram channel

Full access? Get Clinical Tree
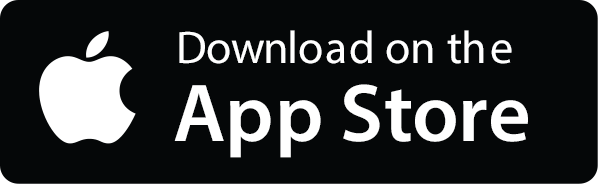
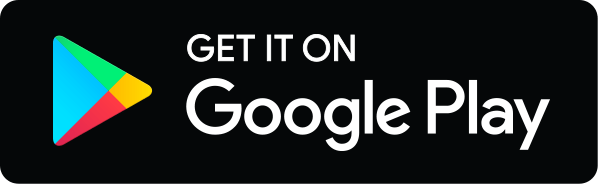