Technical Aspects of Nuclear Imaging
Ventilation and Perfusion Lung Imaging for Diagnosis of Pulmonary Embolism
Angiotensin-Converting Enzyme Inhibitor Renal Scintigraphic Imaging
Positron Emission Tomography and PET/CT
Nuclear medicine is an important medical specialty that uses measurements of radioactive tracer behavior in the body to detect and assess various types of diseases. The physiologic images generated by nuclear imaging procedures reveal less anatomic detail than radiologic studies. It is, therefore, often necessary to correlate the nuclear images with the corresponding radiologic images. Although there are also several therapeutic applications for radioactive agents, the primary emphasis of this chapter will be on diagnostic nuclear imaging.
TECHNICAL ASPECTS OF NUCLEAR IMAGING
When molecules with radionuclide components are prepared for administration to human beings, they are called radiopharmaceuticals. The radionuclide portion of the radiopharmaceutical typically emits radiation in the form of gamma rays and/or x-rays that can be detected and used to create the scintigraphic images (often loosely referred to as scans). Radiopharmaceuticals participate in, but do not alter, various physiologic processes. Specific radiopharmaceuticals with particular physiochemical properties are used to study an organ or organ system. Although radiopharmaceuticals can be introduced into the body in several different ways, the most common mode of administration is intravenously through a peripheral vein.
Side effects from the administration of radiopharmaceuticals for diagnostic imaging are extremely rare. The probability of an adverse reaction from the radiation exposure received by a patient or technologist during a diagnostic nuclear medicine procedure is so small that it has not been measurable to date.
Gamma ray imaging systems are used to detect the radiation emitted from the patient and to create images that depict the regional distribution of the radiopharmaceutical within the body. The most common of these imaging systems is the gamma camera. Gamma cameras use a sodium iodide crystal to detect the gamma rays and x-rays. Photons striking the crystal produce light scintillations that are converted to a digital signal. This digital information is stored in a computer and can be transferred to film or interpreted directly from the computer screen (Fig. 9.1). The resultant picture is often referred to as a scan or more appropriately a scintigraphic image. The image is essentially a physiologic map of the radiopharmaceutical distribution within the body. Table 9.1 presents the radiopharmaceuticals and the corresponding imaging procedures that are discussed in this chapter.
VENTILATION AND PERFUSION LUNG IMAGING FOR DIAGNOSIS OF PULMONARY EMBOLISM
Pulmonary thromboembolism (PTE) is a common disorder associated with significant mortality, which can be reduced with the appropriate detection and treatment. Establishing the diagnosis of PTE is often very difficult. Although dyspnea, tachypnea, and sinus tachycardia are present in the large majority of individuals with acute PTE, these symptoms and signs may result from any number of cardiopulmonary disorders. The diagnosis of pulmonary embolism cannot be made with a high degree of reliability based on clinical findings alone.
A chest radiograph should be obtained in all patients suspected of having pulmonary embolism. The chest x-ray is needed to rule out other causes of the patient’s symptoms such as pneumonia, pneumothorax, heart failure, and so on. However, the radiograph may appear entirely normal even when PTE is present. Even if the chest radiograph is abnormal and consistent with PTE, the results are never adequately predictive to form the basis of a therapeutic decision. Consequently, more specific diagnostic procedures are necessary for the workup of individuals who fall into this category.
Perfusion lung imaging is an important tool in the workup of PTE in which it has a very high sensitivity for detecting this condition. Images of regional pulmonary perfusion are obtained by intravenously injecting several hundred thousand tiny particles of macroaggregated human albumin that are radiolabeled with technetium-99m (Tc-99m). These particles have diameters of 10 to 40 μm. Because the diameter of pulmonary capillaries and precapillary arterioles is less than 10 μm, the radioactive particles lodge in these vessels throughout the lung fields in concentrations that are directly proportional to the regional pulmonary blood flow (Fig. 9.2). Because less than 0.1% of the total cross section of the pulmonary vasculature is occluded by the injected radiolabeled particles, complications are extremely rare.
FIGURE 9.1 The basic gamma camera imaging system. The gamma rays that exit the patient perpendicular to the surface of the camera are not absorbed by the collimator and reach the scintillation crystal where they are detected. The pattern of the gamma ray photons striking the crystal is used to create a digital image on the computer. NaI, sodium iodide.
Figure 9.3 shows an example of the normal pulmonary blood flow pattern. Patients with PTE often display diminished or absent blood flow to one or more lung segments (Fig. 9.4A). The emboli are often large enough to occlude the segmental pulmonary arteries, and hence the flow defects on the images will often appear segmental in configuration. However, thromboembolic occlusion of smaller arteries may occur, and the perfusion pattern may, therefore, reveal defects that are somewhat smaller.
RADIOPHARMACEUTICALS DISCUSSED IN THIS CHAPTER
Lung pathology other than thromboemboli may cause alterations in the regional pulmonary blood flow pattern as well. Disorders of the lung parenchyma such as pneumonia, chronic obstructive lung disease, and regional atelectasis may lead to abnormalities of lung perfusion due to reflex vasoconstriction in the region of the pathology. As a result, the presence of one or more focal blood flow abnormalities is not necessarily specific for a diagnosis of pulmonary thromboemboli. It is for this reason that scintigraphic ventilation imaging of the lungs is typically combined with the perfusion study in the evaluation of PTE. Determination of the ventilation status of a lung region that shows abnormal perfusion improves the specificity of the test for the diagnosis of PTE. Diseases of the lungs that lead to abnormal regional perfusion and corresponding abnormal regional ventilation are summarized in Table 9.2.
FIGURE 9.2 A Tc-99m macroaggregated albumin (MAA) particle as it becomes trapped in the pulmonary capillaries and emits gamma rays.
FIGURE 9.3 Normal perfusion lung images in six projections. Ant, anterior; Post, posterior; LPO, left posterior oblique; RPO, right posterior oblique; LL, left lateral; RL, right lateral. “H” designates area of absent activity due to the heart.
Images of regional pulmonary ventilation are obtained by having the patient breath either radioactive xenon gas or an aerosolized form of Tc-99m diethylenetriamine pentaacetic acid. A normal ventilation pattern with xenon is shown in Fig. 9.5.
PULMONARY DISEASES THAT CAUSE DECREASED REGIONAL LUNG PERFUSION WITH CORRESPONDING ABNORMAL VENTILATION
Pneumonia
Chronic obstructive lung disease
Atelectasis
Asthma
Areas of the lung with diminished perfusion secondary to nonembolic lung pathology are associated with matching ventilation defects (Fig. 9.6). In contrast, ventilation usually appears normal in regions of the lung that show perfusion defects caused by thromboemboli (Figs. 9.4 and 9.7).
Typically, results from the ventilation/perfusion (V/P) study are used to estimate the probability that acute PTE has occurred. An entirely normal perfusion pattern indicates virtually no chance that the patient has emboli, and the clinician should then focus on a search for other causes of the patient’s symptoms. Multiple vascular appearing perfusion defects with correspondingly normal ventilation indicate a very high probability that the patient has PTE.
FIGURE 9.4 A: Six-view perfusion scan showing numerous bilateral segmental flow defects. B: Single-breath ventilation images showing normal ventilation. This pattern is essentially diagnostic for PTE.
FIGURE 9.5 Normal xenon ventilation study. Posterior view of the initial breath hold image.
A computed tomography (CT) chest scan with contrast angiography is now considered the examination of choice (Fig. 2.49) to establish the diagnosis of pulmonary embolism. CT angiography has a sensitivity of 95% for the diagnosis of pulmonary embolism. It is considered by most as the gold standard although perfusion/ventilation scans continue to be performed under special circumstances such as when patients are allergic to intravenous contrast.
Patients with acute cholecystitis classically present with right upper quadrant pain/tenderness, fever, and leukocytosis. However, the signs and symptoms of acute cholecystitis often vary, and there are a number of pathologic conditions that occasionally present in a similar fashion. Consequently, the provisional diagnosis of acute cholecystitis typically requires confirmatory testing with ultrasound and/or hepatobiliary scintigraphy.
Hepatobiliary scintigraphic imaging is performed using a Tc-99m-labeled iminodiacetic acid derivative that is an analog of bilirubin. This radiopharmaceutical is actively transported into hepatocytes by the same system that transports bilirubin and is excreted unchanged into the biliary tract.
FIGURE 9.6 Patient with chronic obstructive pulmonary disease showing matching ventilation and perfusion defects in upper lobes (arrows). A: Posterior perfusion image. B: Posterior initial breath hold ventilation image. C: Later equilibrium ventilation image showing eventual filling in of defects seen on the initial ventilation image.
FIGURE 9.7 Top two images are posterior ventilation images with xenon-133 showing uniform ventilation to both lungs. Bottom four images are from the perfusion study showing multiple segmental defects. Arrow points to region of absent perfusion in what is probably all three segments of the right upper lobe. Note that the lung regions with flow defects have normal ventilation. This pattern indicates a very high probability for PTE.
In normal individuals, the hepatobiliary radiotracer will flow into the gallbladder within the first hour after intravenous administration (Fig. 9.8). However, in acute cholecystitis the gallbladder fails to fill with the radiotracer because of cystic duct obstruction by a stone. This test is extremely sensitive, and a normal result (i.e., visualization of the gallbladder) virtually excludes the possibility of acute cholecystitis. It is important to note that there are certain conditions that predispose to false-positive results. The most common false-positive result occurs in prolonged fasting of more than 2 or 3 days. Under this condition, the gallbladder rarely contracts and its contents begin to partially solidify into a gelatinous material that can impede or block the entry of the radioactive bile into the gallbladder. The gallbladder also fails to visualize in many patients who have ingested food within 2 hours of the hepatobiliary study as a result of the high level of circulating cholecystokinin causing gallbladder contraction, which limits entry of the radioactive bile. For this reason, scintigraphic hepatobiliary imaging should not be performed within 2 hours of a meal. Conditions that lead to false-positive (gallbladder nonvisualization) results are summarized in Table 9.3.
FIGURE 9.8 Normal hepatobiliary study. Images obtained in the anterior view every 2 minutes (moving from left to right and top to bottom) following injection of the hepatobiliary radiotracer show good extraction of the agent by the liver (L). The common bile duct (arrow CD) is seen along with the duodenum (arrow D) and gallbladder (arrow GB).
The use of morphine with hepatobiliary imaging has been found to be helpful in reducing the number of false-positive results, thereby improving the specificity of the test. Morphine causes constriction of the sphincter of Odi and leads to a rise in biliary system pressure. This augments bile movement through the cystic duct, improving gallbladder visualization. For this reason, the use of morphine has become standard practice with this procedure. Figure 9.9 shows a case in which a normal gallbladder became visible only after administration of morphine. Figure 9.10 shows a patient with acute cholecystitis and gallbladder nonvisualization both before and after administration of morphine.
Skeletal scintigraphic imaging, more commonly referred to as the bone scan, is a valuable tool for investigation of a number of disorders of the skeletal system. A Tc-99m-labeled diphosphonate derivative is used to perform skeletal scintigraphy because this radiolabeled agent is adsorbed onto the surface of newly forming hydroxyapatite crystal in the bone. New bone formation occurs in response to the presence of almost all skeletal pathology. Consequently, scintigraphic images will demonstrate increased gamma ray emissions localized to the site of bone abnormality.
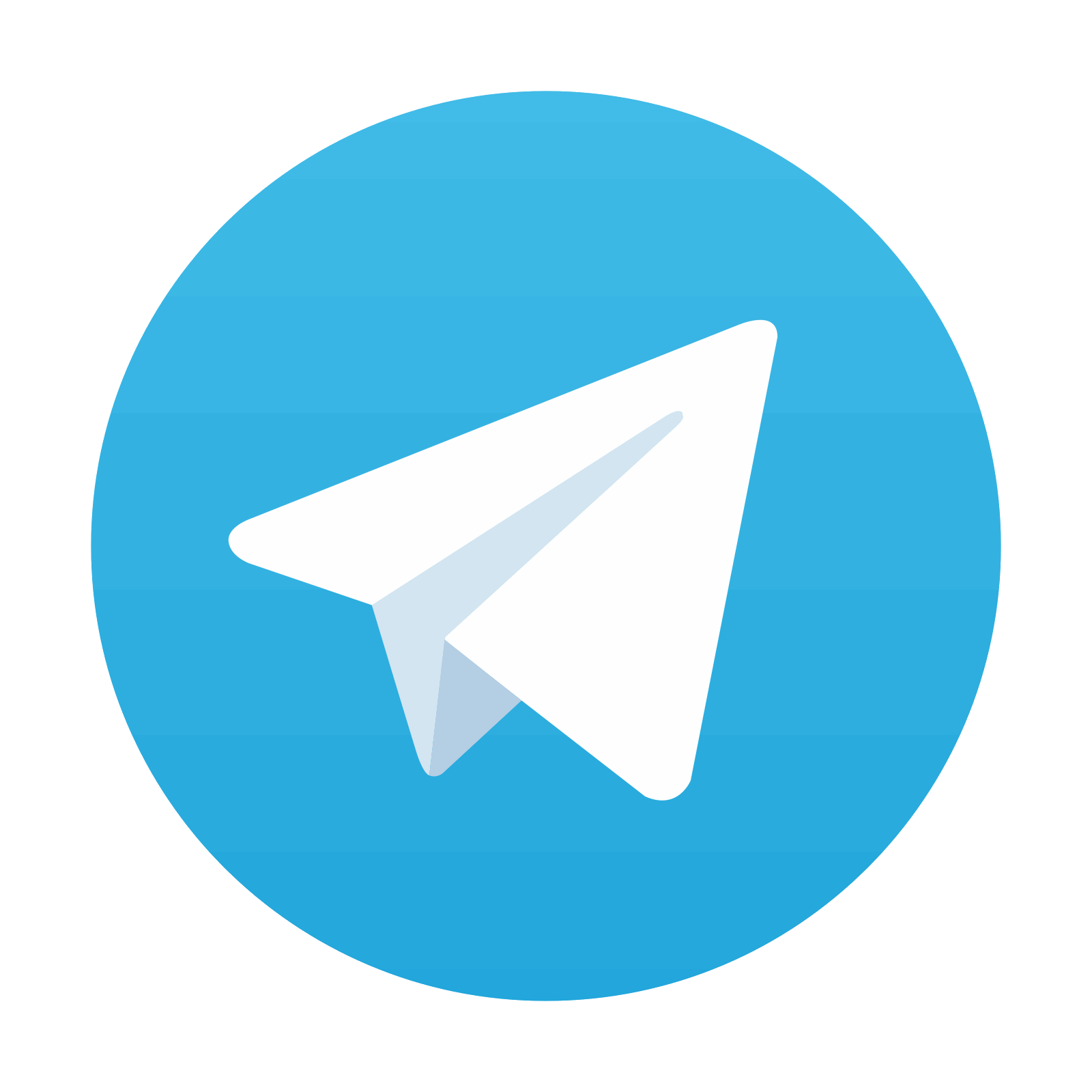
Stay updated, free articles. Join our Telegram channel

Full access? Get Clinical Tree
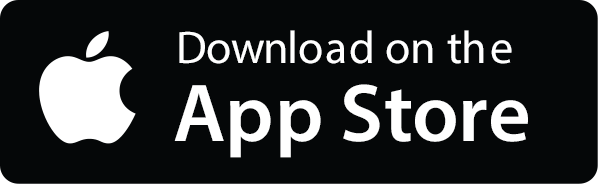
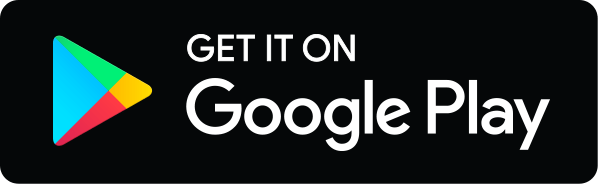