Anatomy
The soft tissue structures of the orbit are contained within a bony cavity and include the globe, extraocular muscles, optic nerve–sheath complex, lacrimal apparatus, and various vascular and nerve structures ( Figs. 20-1 to 20-4 ).
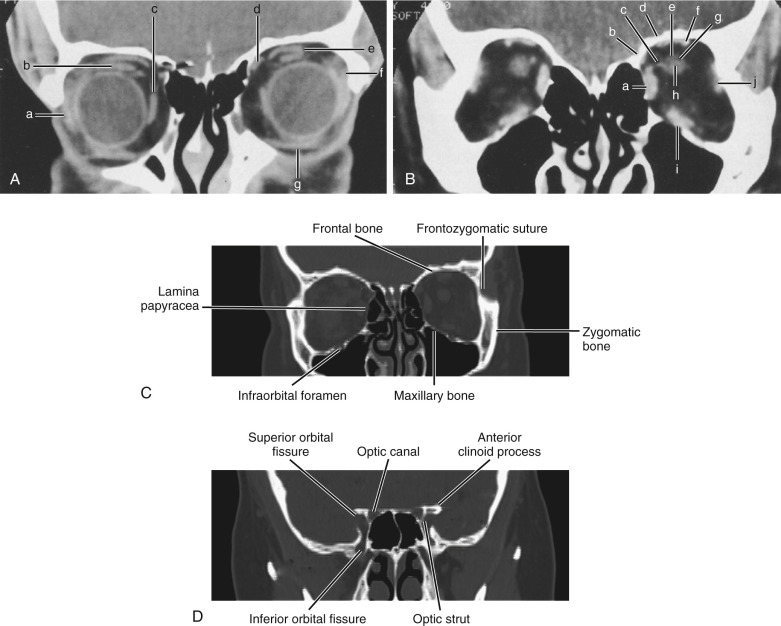
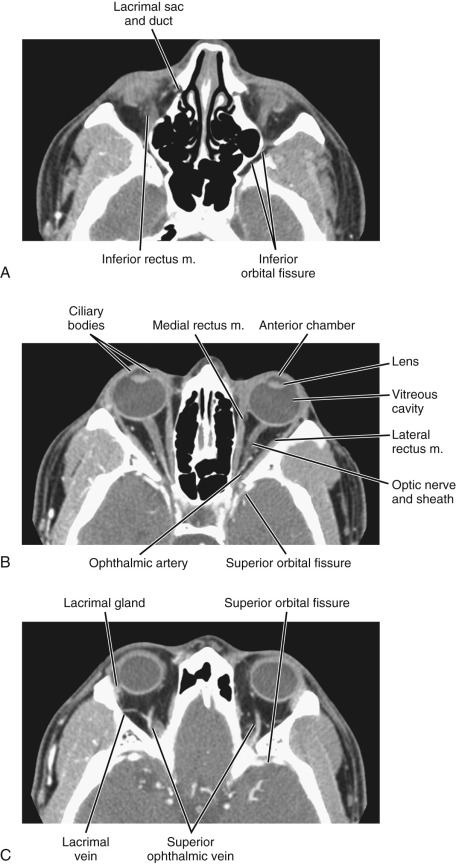
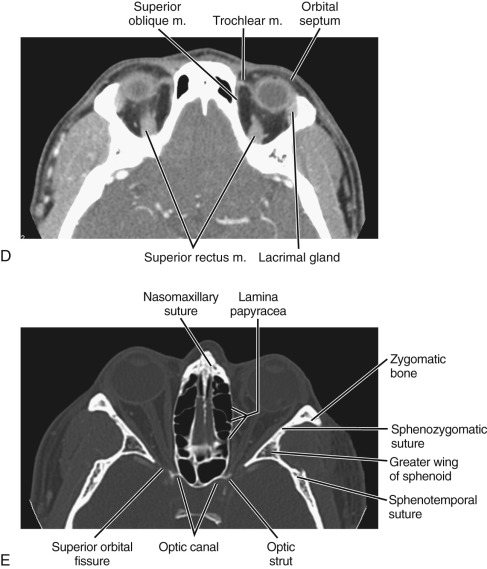
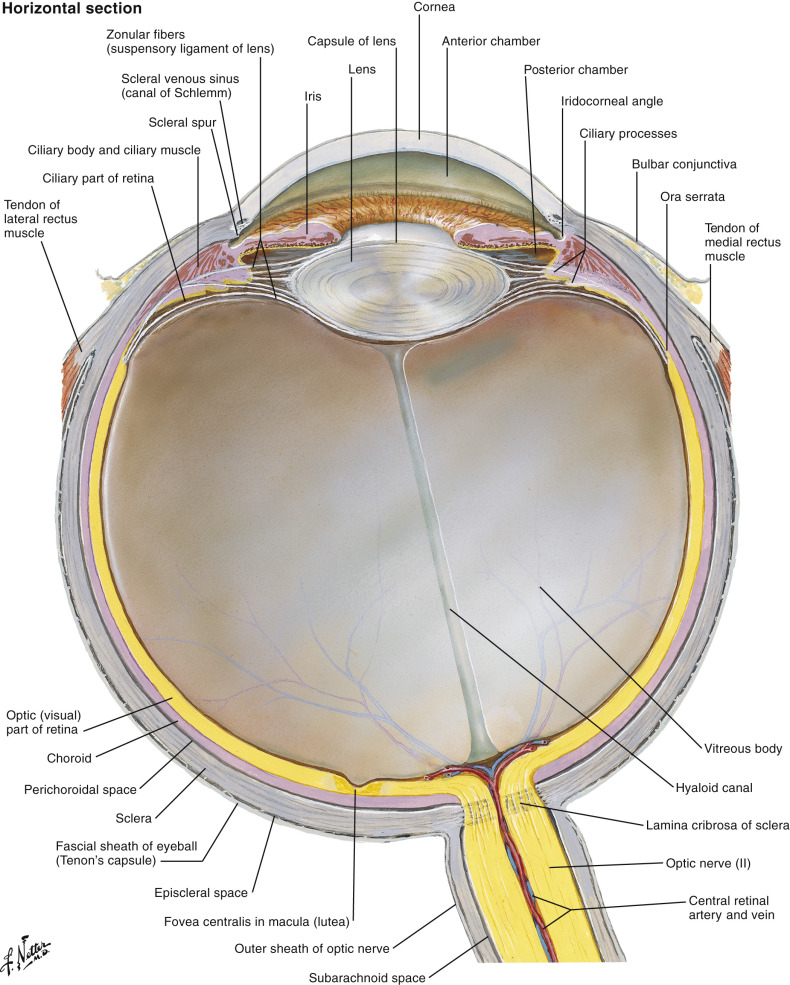
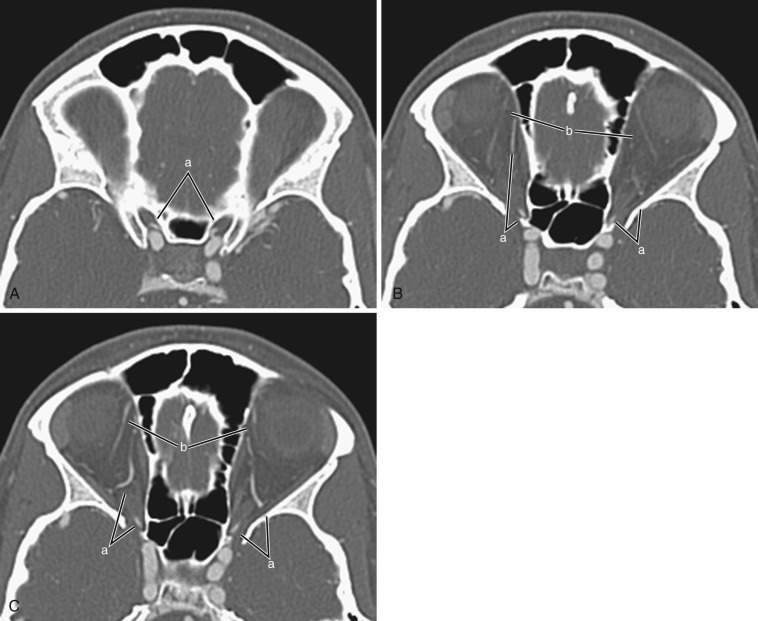
Bony Anatomy
The bony orbit is a conical structure with the apex pointing posteriorly. The orbital roof is composed of the frontal bone and is thinner anteriorly. The medial wall is composed of the frontal process of the maxillary bone anteriorly, the lamina papyracea of the ethmoid air cells at the midportion, and the sphenoid bone posteriorly. The lamina papyracea is very thin, and not surprisingly it is a common site of orbital blowout fracture and spontaneous dehiscence of orbital fat. The lateral orbital wall is formed by the orbital surface of the zygomatic bone. The orbital floor is formed by the orbital plate of the maxilla, the orbital process of the palatine bone, and the orbital surface of the zygomatic bone. The orbital plate of the maxilla is thin and a common site of inferior blowout fracture.
Multiple foramina and canals go through the bony orbits ( Box 20-1 ) (see Figs. 20-1 and 20-2 ). The optic canal (also called optic foramen ) is located at the orbital apex. It is bordered by two bony spikes of the lesser wing of the sphenoid bone, commonly referred to as the optic struts. The canal contains the optic nerve and ophthalmic artery, both of which are contained within a dural sheath.
The superior orbital fissure is located at the margin between the lateral wall and the orbital roof. The greater wing of the sphenoid bone forms its lateral boundary, and the lesser wing forms its medial boundary. The superior orbital fissure contains the superior ophthalmic vein; the oculomotor (III), trochlear (IV), and abducens (VI) nerves; and the ophthalmic division of the trigeminal nerve (V 1 ). The superior orbital fissure forms the largest communication between the orbit and intracranial structures and therefore forms a conduit for infectious or neoplastic processes between the orbital apex and the cavernous sinus.
The inferior orbital fissure is located at the margin between the lateral wall and the orbital floor. It contains the infraorbital (branch of V 2 ) and zygomatic nerves, the nerve branches from the pterygopalatine ganglion, and venous connection between the inferior ophthalmic vein and the pterygoid plexus. The inferior orbital fissure connects with the pterygopalatine fossa and the masticator space/infratemporal fossa, allowing the spread of deep facial infection and neoplasm to the orbital apex.
The globe is essentially a spherical structure, with the wall consisting of three layers: retina (innermost), choroids (middle), and sclera (outermost) ( Fig. 20-3 ). These layers cannot be resolved with current clinical computed tomography (CT) or magnetic resonance imaging (MRI) technology unless they are separated by pathologic processes (e.g., retinal detachment). The globe is divided into three fluid-filled cavities: anterior chamber, posterior chamber, and vitreous cavity. The anterior chamber and posterior chamber constitute the anterior segment, and the vitreous cavity constitutes the posterior segment. The anterior chamber extends from the cornea to the iris. The posterior chamber extends from the posterior surface of the iris to the anterior surface of the vitreous. The vitreous cavity is posterior to the posterior chamber.
The anterior border of the orbit is formed by the orbital septum (see Fig. 20-2D ), a fibrous structure adherent to the inner margin of the orbital rim, with central portions that extend into the tarsus of the eyelids. Although there are a few orifices for passage of vessels, nerves, and ducts, the septum forms an effective barrier to prevent superficial processes from extending into the orbit proper. A pathologic process such as cellulitis may be designated as preseptal versus postseptal . A postseptal process signals involvement of more critical structures of the orbit and the possibility of extension into the cavernous sinus and intracranial structures.
Soft Tissue Anatomy
There are seven extraocular muscles: the superior, inferior, medial and lateral rectus; the superior and inferior oblique; and the levator palpebrae superioris muscles. The levator palpebrae muscle can be seen immediately above the superior rectus muscle. With the exception of the inferior oblique muscle, all extraocular muscles originate from the annulus of Zinn, a tendinous ring in the orbital apex. They pass anteriorly and insert on the globe just behind the corneoscleral border. The four rectus muscles and the fibrous septa connecting between them form the muscle cone of the orbit. The intraconal space is filled with orbital fat. Orbital vessels, sensory and motor nerves to the extraconal muscles, and the optic nerve–sheath complex also traverse the intraconal space.
The optic nerve may appear straight or slightly tortuous depending on the eye position. It consists of three segments: orbital, canalicular, and intracranial. The orbital segment is covered by the same meningeal sheaths as the brain. The normal diameter of the optic nerve is up to 4 mm. A layer of cerebrospinal fluid can be seen between the meningeal sheath and optic nerve.
The extraconal space represents the area between the muscle cone and bony orbit. This space contains orbital fat and the lacrimal gland. The lacrimal gland is located superolateral to the globe. The upper margin of the gland is convex. The lower margin is concave and lies on the levator palpebrae and lateral rectus muscles. The lacrimal system drains through the lacrimal ductal system near the medial canthus. It consists of the superior and inferior puncta, their associated ducts, the lacrimal sac, lacrimal duct, and the valve of Hasner, which is a draining orifice inferolateral to the inferior nasal turbinate.
The vascular anatomy of the orbits can be well demonstrated on high-resolution MRI and CT angiography (CTA). The primary arterial supply to the orbit is the ophthalmic artery. It is superior to the optic nerve and can be seen crossing the optic nerve almost perpendicularly ( Fig. 20-4 ). The ophthalmic artery most often originates from the internal carotid artery. The origin is usually at the anteromedial aspect of the internal carotid artery as it exits the cavernous sinus. Variants of its origin include the cavernous segment of the internal carotid artery and the middle meningeal artery (i.e., external carotid artery branch). Secondary arterial supply to the orbits comes from the external carotid artery. Because the orbits receive blood supply from both the internal and external carotid arteries, orbital arteries may serve as anastomoses between the two arterial systems.
The largest orbital vein visualized on CT or MRI is the superior ophthalmic vein. It can be seen arising near the base of the nose, coursing anteromedially to posterolaterally, and draining into the cavernous sinus. It crosses over the optic nerve in its midcourse at approximately 20 degrees (see Fig. 20-4 ). The midportion of the superior ophthalmic vein is an intraconal structure that lies between the superior rectus muscle and the ophthalmic artery. The inferior ophthalmic vein is much smaller than the superior ophthalmic vein. It is usually not well visualized on CT or MRI studies. Both the superior ophthalmic vein and inferior ophthalmic vein receive tributaries from the veins of the face and nose.
Imaging Techniques
The major modalities for imaging the orbits include CT and MRI. The abundance of intraorbital fat provides good intrinsic soft tissue contrast on CT for most clinical applications. The advances of multidetector CT technology now make high-resolution CT imaging possible. The source images can be reformatted in different planes, providing high-resolution isotropic imaging. This renders the previous advantage of multiplanar capability of MRI obsolete. CT is superior to MRI for delineation of osseous structures and calcifications. It requires short imaging time and is therefore less sensitive to motion of the globe and eyelid. CT imaging can be completed quickly and requires less patient cooperation, making it ideal for imaging orbital trauma.
Compared to CT, MRI provides superior soft tissue contrast. It also provides better imaging details of the intracranial structures. When it is important to assess intracranial abnormalities, either as direct extension of orbital lesions or as associated lesions in certain diseases (e.g., in multiple sclerosis), MRI is superior to CT.
In the past, evaluation of suspected vascular lesions of the orbits required conventional angiography. The advances in CTA and MRA now allow many vascular lesions to be evaluated noninvasively. In some cases, conventional angiography can be foregone.
CT and MRI often provide complementary roles in orbital imaging. The choice of CT versus MRI for initial imaging of the orbits depends on the clinical problem. CT is usually preferred for trauma, for evaluation of the bony orbits or calcified lesions, and when MRI is contraindicated. For other applications, MRI is generally preferred because of the absence of radiation risks and its high soft tissue contrast. MRI is the initial imaging of choice for evaluation of the optic nerve, other cranial nerves, and intracranial lesions. Exceptions can be found in a small number of optic nerve meningiomas, which are very small and mostly calcified. These lesions may be missed by MRI and are better detected by CT.
Computed Tomography
The orbits are often included in routine CT head or maxillofacial CT examinations. These screening examinations are usually performed according to the standard head or maxillofacial CT protocols.
When dedicated orbital CT is performed, thin sections (usually < 3 mm and preferably < 1.5 mm) are acquired. Coronal images are especially important in that cross-sectional evaluation of all of the intraorbital structures is optimal (e.g., extraocular muscles, optic nerve–sheath–nasal complex, vessels, and globe) (see Fig. 20-1 ). This plane is also imperative for assessing spread of processes from surrounding structures (e.g., paranasal sinuses, trauma, tumor). A typical orbital CT protocol can be performed with scanning in the axial plane. This plane is usually chosen to be parallel to the orbital long axis. In practice, imaging is performed in the plane parallel to the infraorbital-meatal line. Coronal images should be included in the routine protocol and can be obtained by multiplanar reformation. This can be performed in the plane perpendicular to the axial plane. Parasagittal reformation in a plane parallel to the long axis of the optic nerve may also be added.
Intravenous contrast is often used in the evaluation of inflammatory, infectious, neoplastic, and vascular orbital diseases. For evaluation of vascular lesions, a bolus injection may be used for better depiction of its arterial blood supply.
When orbital varix is suspected, the CT study should be repeated without and with the Valsalva maneuver. Enlargement of a lesion with the Valsalva maneuver is indicative of an orbital varix. Less commonly, cavernous hemangiomas may enlarge with the Valsalva maneuver. In patients unable to cooperate, similar effects can be obtained by positioning the patient prone during scanning.
CTA can provide good depiction of the major vascular anatomy in the orbits. In addition to the ophthalmic artery and superior ophthalmic vein, their branches, tributaries, and many other smaller vessels can often be seen and traced. The study can be performed as part of a CT angiographic study of the head and neck (see Fig. 20-4 ). Bolus injection of iodinated contrast is required. It is important to use a field of view sufficiently wide to include extraocular pathology that may be associated with the vascular orbital lesions, such as carotid-cavernous fistula.
CT dacryocystograms can be performed by administration of contrast material into the nasolacrimal duct to evaluate for patency ( Fig. 20-5 ). This requires cannulation of the lacrimal duct, usually by an ophthalmologist.
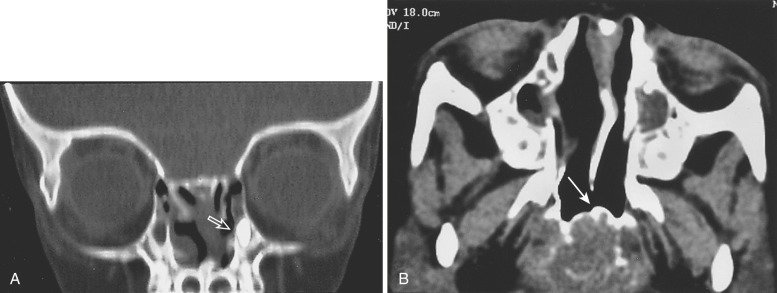
Magnetic Resonance Imaging
MRI of the orbits can be performed with the head coil. For high-spatial-resolution imaging of the anterior orbital structures, special orbital surface coils may be advantageous. However, the sensitivity of surface coils decreases rapidly with distance from the coils, leading to rapid signal falloff and inadequate coverage of deeper structures.
For routine imaging, the field of view should include the cavernous sinus, optic chiasm, optic tracts and radiations, and the nuclei of the oculomotor, abducens, and trochlear nerves in the midbrain and pons.
The protocol should include T1- and T2-weighted imaging in axial and coronal planes ( Fig. 20-6 ). Intravenous gadolinium (Gd) contrast is routinely used. For dedicated orbital imaging, fat suppression is usually performed for T2-weighted imaging and post-Gd imaging to prevent obscuration of enhancing lesions by the high intraorbital fat signal ( Figs. 20-7 and 20-8 ).
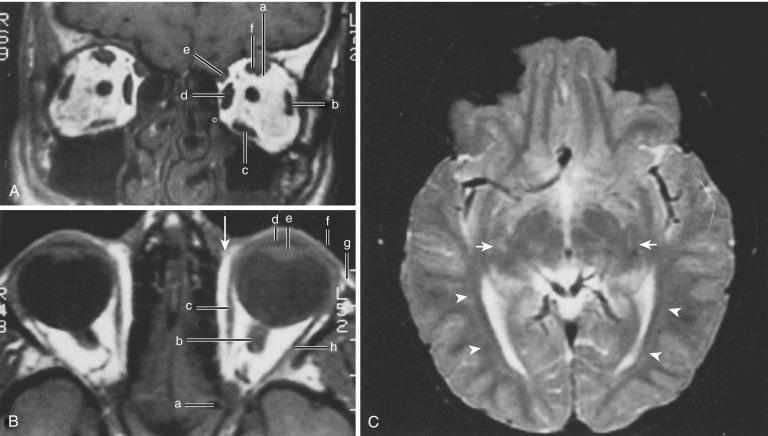
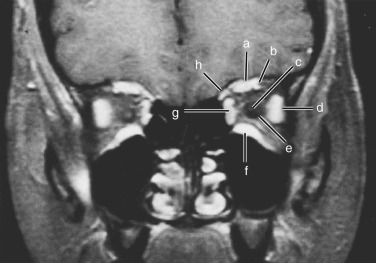
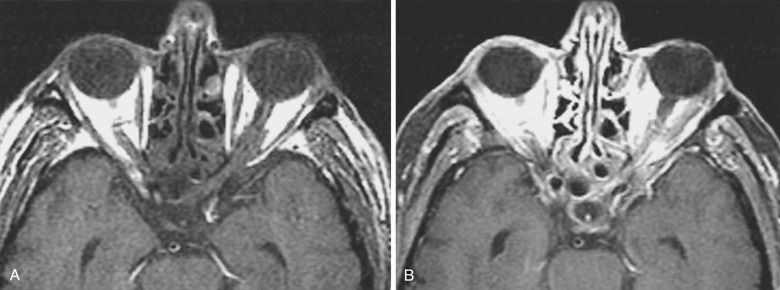
Fat suppression is often performed with standard frequency selective presaturation radiofrequency (RF) pulses. Alternative advanced fat-suppression techniques can provide more robust fat suppression in adverse conditions when magnetic field inhomogeneity is encountered. These techniques may be based on specialized RF pulses (e.g., adiabatic pulses) or modifications of the Dixon technique (e.g., iterative decomposition of water and fat with echo asymmetry and the least-squares estimation [IDEAL]). The fat suppression for fluid-sensitive imaging (i.e., T2-weighting) can also be performed effectively using inversion recovery ( Fig. 20-9 ).
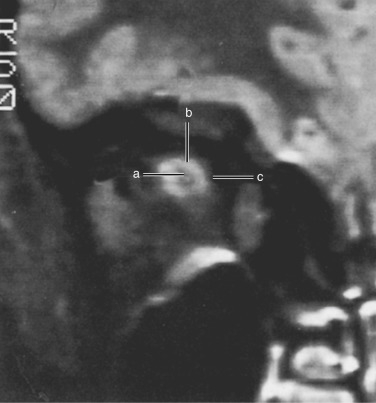
Orbital MRI is susceptible to image artifacts because of several factors. First, chemical shift artifacts may be seen at the interface of the orbital fat and the globe. Similar artifacts may also be present if silicone oil is used to fill the globe in treatment of retinal detachment. These chemical shift artifacts can be reduced by using fat or silicone saturation, using a higher gradient strength, or narrowing the bandwidth. Second, the proximity of orbital structures to the air cavities of paranasal sinuses makes orbital imaging susceptible to image artifacts. Exogenous metallic materials (e.g., cosmetics) can also lead to susceptibility artifacts. Third, motion artifacts may be present. To minimize motion of the globe, a patient can be asked to fixate his or her vision at a certain object when the eyes are open. Temporal averaging can also be performed.
MR dacrocystography can be performed in a similar fashion as CT dacrocystography, by cannulation and instillation of Gd contrast material into the nasolacrimal duct. It has comparable sensitivity to CT dacrocystography.
Approach to Differential Diagnosis
A large number of disease processes can involve the orbits, and orbital complaints such as proptosis, orbital pain, visual loss, and ophthalmoplegia are nonspecific. Proptosis is abnormal protrusion of the globe; exophthalmos is abnormal prominence of the globe. On imaging, proptosis is best evaluated at a level of the lens on axial images. A line connecting the most distal tips of the lateral orbital walls is drawn. The distance from the anterior margin of the globe to this line should not exceed 21 mm.
General Principle
Various characteristics of an orbital lesion can be used to help construct a differential diagnosis. These include its location, anatomic structure, and imaging features and the clinical presentation of the patient. Using a compartmental approach a lesion is first localized to one of the four compartments: globe, optic nerve–sheath complex, intraconal space, or extraconal space. Differential diagnosis of an extraconal lesion can be further refined if it can be determined to be associated with the lacrimal gland and apparatus. Once the primary location of a lesion is determined, other parameters including imaging features (e.g., characteristics of margin, associated bony changes, enhancement patterns), pathophysiologic basis, age of presentation, and chronicity can be considered to further reduce the differential diagnosis. The presence of calcification may also be helpful in refining the differential diagnosis, especially for globe lesions.
Obviously some lesions may extend over more than one compartment. Nevertheless this compartmental approach helps simplify the diagnostic thought process. The optic nerve–sheath complex, strictly speaking, is also an intraconal structure. However, because of its unique significance, it can be considered a separate compartment to improve the specificity of the differential diagnosis. Differential diagnosis of orbital lesions is summarized in Boxes 20-2 to 20-7 .
Congenital
Persistent hyperplastic primary vitreous
Coats’ disease
Coloboma
Globe hypoplasia/aplasia
Degenerative
Optic nerve drusen
Phthisis bulbi
Staphyloma
Trauma
Vitreous hemorrhage
Choroidal hematoma
Choroidal effusion
Foreign body
Inflammatory
Orbital pseudotumor (uveal/scleral thickening)
Sclerosing endophthalmitis (Toxocara canis)
Neoplasm
Uveal melanoma (adults)
Retinoblastoma (children)
Metastasis
Choroidal hemangioma
Medulloepithelioma
Congenital
Degenerative
Cataracts
Optic nerve drusen
Phthisis bulbi
Retinal detachment (chronic)
Retrolental fibroplasia
Calcification of ciliary muscle insertion
Iatrogenic (e.g., scleral banding)
Trauma
Foreign body
Inflammatory
Infection (cytomegalovirus, herpes simplex, rubella, syphilis, toxoplasmosis, tuberculosis)
Neoplasm
Astrocytic hamartoma (neurofibromatosis, tuberous sclerosis, von Hippel-Lindau syndrome)
Retinoblastoma (children)
Choroidal osteoma
Metabolic
Hypercalcemia
Sarcoidosis
Trauma
Contusion
Hematoma
Optic nerve avulsion
Infection
Toxoplasmosis
Tuberculosis
Syphilis
Noninfectious Inflammatory
Thyroid ophthalmopathy
Optic neuritis
Pseudotumor
Sarcoidosis
Vascular
Central retinal vein occlusion
Neoplasm
Optic nerve glioma
Meningioma
Neurofibroma
Schwannoma
Lymphoma/leukemia
Metastasis
Hemangioblastoma
Hemangiopericytoma
Miscellaneous
Increased intracranial pressure
Optic hydrops
Trauma
Hematoma
Foreign body
Infection
Cellulitis
Abscess
Noninfectious Inflammatory
Thyroid ophthalmopathy
Pseudotumor
Sarcoidosis
Wegener’s granulomatosis
Vascular
Carotid-cavernous fistula
Venous varix
Superior ophthalmic vein thrombosis
Venous angioma
Arteriovenous malformation
Cavernous hemangioma (adults)
Capillary hemangioma (children)
Lymphangioma
Neoplasm
Lymphoma
Metastasis
Rhabdomyosarcoma (children)
Hemangiopericytoma
Neurofibroma/schwannoma (cranial nerve III, IV, VI)
Ectopic meningioma
Trauma
Fracture
Hematoma
Infection
Cellulitis
Abscess
Noninfectious Inflammatory
Pseudotumor
Postviral syndrome (lacrimal gland)
Sjögren’s syndrome (lacrimal gland)
Mikulicz’s syndrome (lacrimal gland)
Neoplasm
Metastasis
Primary malignancy from adjacent structures
Benign mixed tumor (lacrimal gland)
Adenoid cystic carcinoma (lacrimal gland)
Non-Hodgkin’s lymphoma
Rhabdomyosarcoma (children)
Congenital
Cephalocele
Dermoid/epidermoid
Trauma
Hematoma
Infection
Dacryoadenitis
Noninfectious Inflammatory
Pseudotumor
Postviral syndrome
Sarcoidosis
Sjögren’s syndrome
Mikulicz’s syndrome
Wegener’s granulomatosis
Neoplasm
Papilloma
Benign mixed tumor (pleomorphic adenoma)
Adenoid cystic carcinoma
Mucoepidermoid carcinoma
Adenocarcinoma
Malignant mixed tumor
Undifferentiated carcinoma
Squamous cell carcinoma
Sebaceous carcinoma
Primary malignancy from adjacent structures
Non-Hodgkin’s lymphoma
Metastasis
Dermoid/epidermoid
Congenital
Dacryocele
Dacryocystocele
Apart from aiding differential diagnosis, localization of a lesion in the extraconal space versus the intraconal space may also have management implications. In general, intraconal lesions may require surgical attention, whereas extraconal lesions may be amenable to medical management.
Specific Clinical Scenarios
It may be helpful to give a few clinical scenarios their own differential consideration. The first is lesions of the lacrimal gland and apparatus. Lacrimal lesions are most often benign inflammatory processes, with tumors being less common. Viral adenitis is the most common acute process. More chronic inflammatory processes include sarcoidosis, Wegener’s granulomatosis, and Sjögren’s syndrome. Histologically the lacrimal gland is analogous to the minor salivary gland in other regions of the head and neck. They therefore share many common pathologic processes. Most lacrimal gland tumors are epithelial cell tumors, with half of these being benign mixed tumors and half carcinomas. Lymphoma also occurs commonly at the lacrimal gland fossa (see further discussion under “ Lacrimal Gland and Apparatus ”).
In a young patient presenting with leukokoria, one will need to exclude retinoblastoma. Other differential considerations include developmental and congenital conditions such as retinopathy of prematurity, Coats’ disease, persistent hyperplastic primary vitreous, toxocariasis, retina dysplasia, and congenital retinal fold.
Pathophysiology
Orbital diseases can be categorized based on their pathophysiology: trauma, infection, noninfectious inflammation, neoplasm, vascular lesions, congenital and developmental abnormalities, and degenerative conditions. A more popular approach for differential diagnosis is based on a compartmental approach (see earlier).
Trauma
CT is the imaging method of choice for evaluation of orbital trauma. The most common traumatic injury is fracture of the orbital walls. Less commonly, hemorrhage in the globe, globe rupture, perforation and penetrating injury, and contusion or avulsion of the optic nerve sheath may occur. A common type of orbital fracture is “blowout” fracture, which results from increased intraorbital pressure transmitted to the orbital walls secondary to blunt trauma ( Fig. 20-10 ). Blowout fractures most often involve the inferior and medial walls because they are the thinnest. Inferior blowout fracture commonly involves the infraorbital foramen, which is the weakest point of the orbital floor. Intraorbital soft tissue contents may herniate through the fracture. Muscle entrapment is a potential complication of orbital fractures. Because the extraocular muscles are tethered to the orbital walls by tiny fibrous strands that are too small to image on CT or MRI, muscle entrapment may occur even without herniation of the muscle itself.
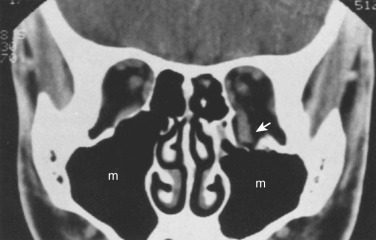
Evaluation for foreign bodies is best performed with thin-section CT ( Fig. 20-11 ). Wood fragments pose a challenge to CT evaluation because they may have variable densities owing to differences in hydration. Wood may appear hypodense, isodense, or hyperdense. Air may be present within a wood fragment. Therefore unusual air pockets should be evaluated carefully. In certain situations, wooden and organic foreign bodies may be better evaluated with MRI.
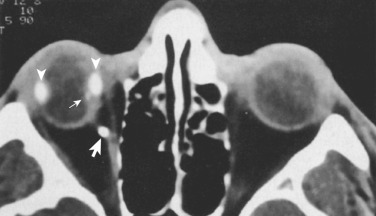
Infection
Orbital infection is most often caused by direct extension from adjacent structures; hematogenous infection is less common. It is important to localize orbital infection to the following compartments: (1) preseptal versus postseptal and (2) extraconal versus intraconal. Preseptal or extraconal infection can usually be treated by standard antimicrobial therapy. Postseptal or intraconal infection requires more aggressive management because of the risk of neurovascular injury and further intracranial spread. Identification of orbital abscesses is also crucial because they may require surgical intervention.
Orbital infection is most commonly caused by contiguous spread of sinusitis or a superficial periorbital cellulitis of the face. In children, infection is most commonly secondary to extension from ethmoid air cells ( Fig. 20-12 ), whereas in adults, extension from the frontal sinus is most common ( Fig. 20-13 ). Common organisms include Streptococcus pneumoniae and β-hemolytic streptococci. Haemophilus influenza e , staphylococci, and anaerobes are less common.
