Chapter 33 Paediatric oncology
Introduction
Cancer in childhood is uncommon. In the UK, approximately 1500 children below the age of 15 develop cancer each year. Approximately one individual child in 500 will develop cancer before the age of 15. The range of childhood cancers is very different from that seen in the adult population. Table 33.1 summarizes data on relative incidence of the various tumour types from the US Surveillance, Epidemiology and End Results (SEER) programme.
Table 33.1 Surveillance, epidemiology and end results (SEER) programme registrations 1975–2001, annual incidence rate per 1 000 000 and proportion of children aged 0–14 with cancer
Disease group | Annual incidence rate per 1 000 000 | Percentage of total |
---|---|---|
Acute lymphoblastic leukaemia (ALL) | 32.8 | 23.6 |
Acute non-lymphoblastic leukaemia (ANNL) | 6.4 | 4.6 |
Other leukaemias | 3.0 | 2.2 |
All leukaemias | 42.2 | 30.3 |
Astrocytoma | 14.5 | 10.4 |
Medulloblastoma/PNET | 6.6 | 4.7 |
Ependymoma | 2.7 | 1.9 |
Intracranial germ cell tumours | 1.1 | 0.8 |
Other CNS tumours | 5.7 | 4.1 |
All CNS tumours | 29.4 | 21.1 |
Hodgkin’s disease | 6.0 | 4.3 |
Non-Hodgkin’s lymphoma | 7.8 | 5.6 |
Osteosarcoma | 3.5 | 2.5 |
Ewing’s sarcoma/peripheral PNET | 2.4 | 1.7 |
Rhabdomyosarcoma | 4.8 | 3.5 |
Other sarcoma | 5.3 | 3.8 |
Neuroblastoma | 10.3 | 7.4 |
Wilms’ tumour | 8.3 | 6.0 |
Total | 139.1 |
Toxicity of radiotherapy for children
Tolerance of critical organs to radiotherapy
The tolerance of critical organs frequently limits the dose of radiation that can be given. The critical organs and their ‘tolerance doses’ are listed in Table 33.2.
Table 33.2 Normal tissue tolerance doses
Tissue/organ | Tolerance dose (Gy) |
---|---|
Whole lung | 15–18 |
Both kidneys | 12–15 |
Whole liver | 20 |
Spinal cord | 50 |
Leukaemia
1. remission induction, usually with vincristine, corticosteroids and asparaginase
2. intensification with multidrug combinations. The number of intensification modules is dependent upon risk status at presentation
3. CNS prophylaxis with intrathecal methotrexate. Cranial radiotherapy is no longer employed except for patients presenting with CNS involvement by leukaemia
4. maintenance, usually based on a continuous low dose antimetabolite drug such as 6-thioguanine, with a total duration of therapy of approximately 2 years.
During the 1960s and 1970s, the routine use of prophylactic whole brain radiotherapy and intrathecal methotrexate reduced the risk of CNS relapse to less than 10%. Whole brain radiotherapy may be employed for patients who present with CNS involvement (Figure evolve 33.1 ). Patients are immobilized in a head shell and treated with lateral-opposed 4–6 MV megavoltage fields which may be centred on outer canthus to minimize divergence into the contralateral lens. Shielding will cover the face, dentition, nasal structures and lenses. The clinical target volume (CTV) includes the intracranial meninges extending inferiorly to the lower border of the second or third cervical vertebra. Great care is taken to include the cribriform fossa, temporal lobe and base of skull. Although the lens is shielded, as much of the posterior orbit as possible is included as ocular relapses occasionally occur. The CTV is localized with lateral simulator radiographs or, more recently, computed tomography (CT) simulation. The prescribed dose in current UK protocols is 24 Gy in 15 fractions of 1.6 Gy daily.
Boys who suffer a testicular relapse are treated with testicular radiotherapy (Figure 33.2). The technique employed is an anterior field, generally electrons or orthovoltage (200–300 kV). The CTV includes both testes, scrotum and inguinal canal superlaterally as far as the deep inguinal ring with shielding of non-target skin and perineum. The prescribed dose is 24 Gy in 12 fractions of 2.0 Gy daily.
Total body irradiation (TBI)
As an example of a TBI technique, the Leeds technique is described. The patient lies in the lateral position in an evacuated polystyrene immobilization bag. Hands are placed under chin to provide ‘lung compensation’ (Figure evolve 33.3 ). Dosimetry is determined using in-vivo measurements performed at a ‘test dose’ of 0.2 Gy for each field. For such a large and complex target volume, it is not feasible to adhere to the ICRU 50 guidelines of a range of −5% to +7%, and a range of −10% to +10% is more realistic. The standard TBI dose for children in the UK is 14.4 Gy in eight fractions of 1.8 Gy twice daily with a minimum interfraction interval of 6 hours.
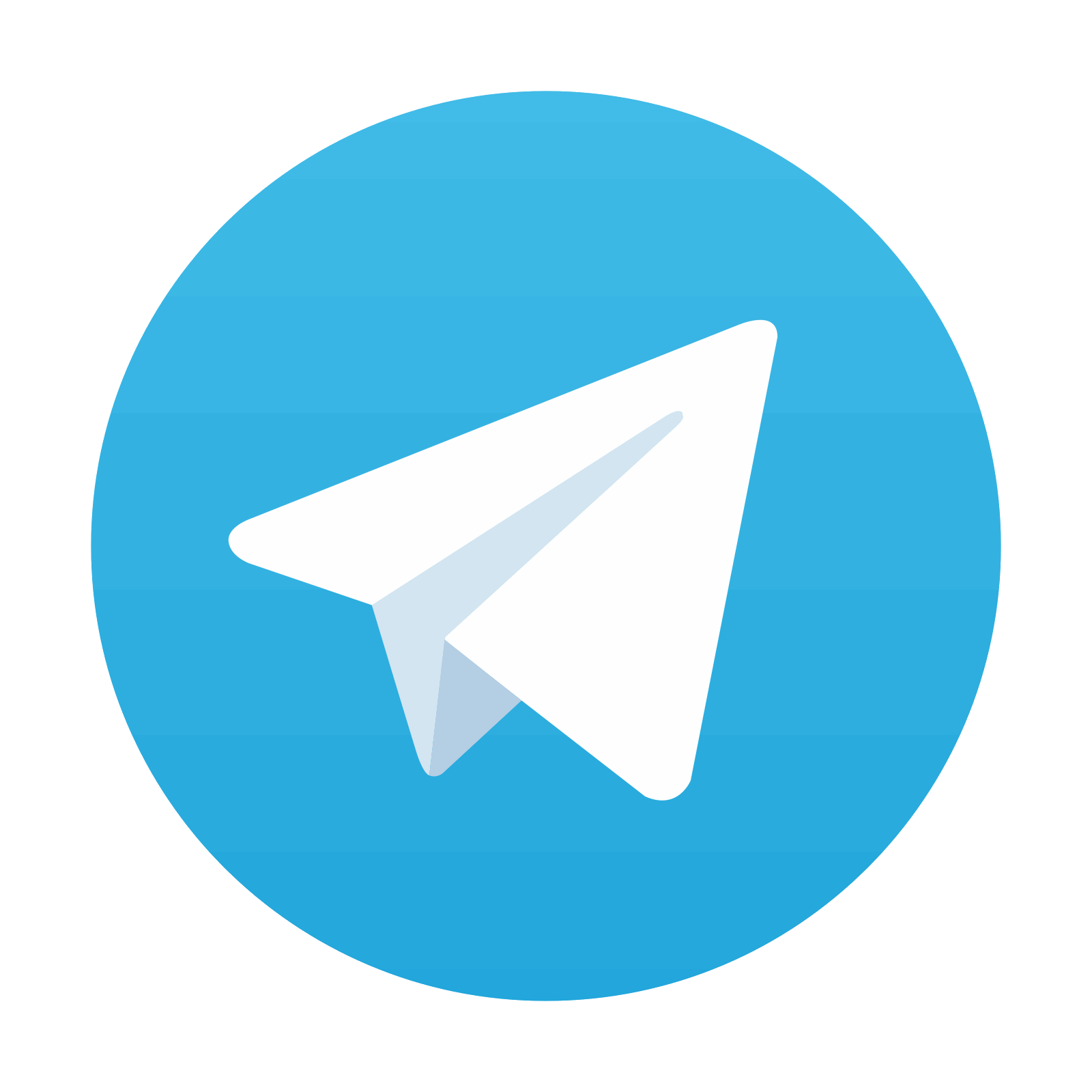
Stay updated, free articles. Join our Telegram channel

Full access? Get Clinical Tree
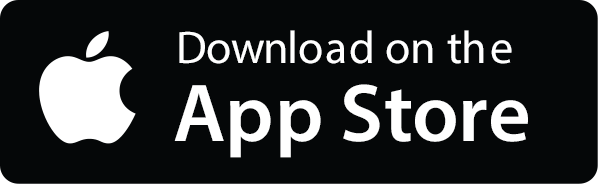
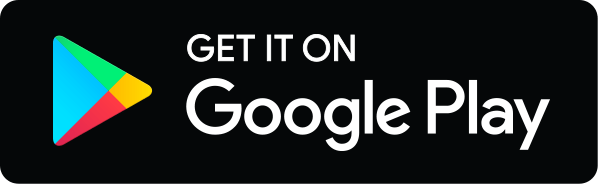