Part 12 Quality Control
Case 135
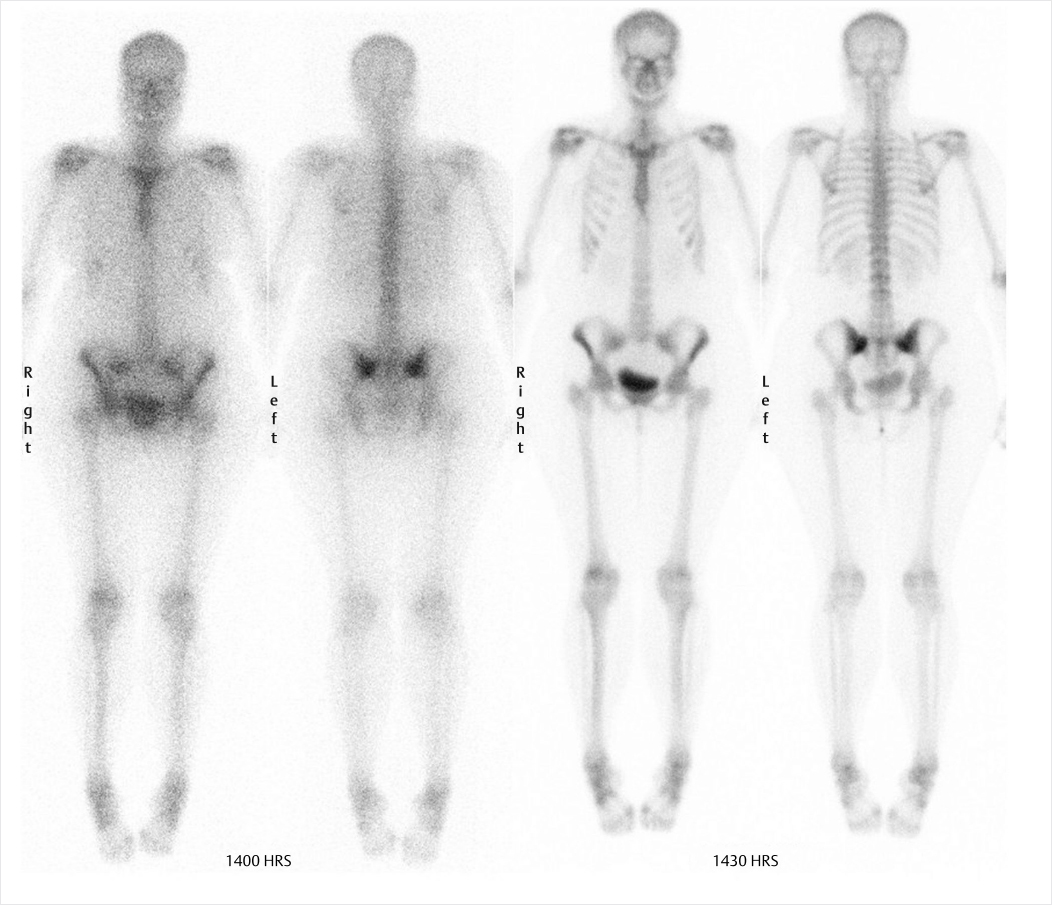
Clinical History
24-year-old female with chronic hip pain. What is the difference between these two scans performed on the same day? (▶Fig. 135.1)
Key Finding
Poor-quality bone scan
Top 3 Differential Diagnoses
Off-peak gamma camera. For image acquisition, gamma cameras employ an energy window centered about the photopeak of the desired radioisotope in order to reject scatter radiation. In case of Tc-99m a 20% energy widow is centered about the 140 keV photopeak. Daily morning QC involves extrinsic field uniformity testing using a cobalt 57 (Co-57) sheet source prior to camera use for clinical studies. The extrinsic field uniformity test requires an energy window for Co-57 (122 keV) which is lower than Tc-99m. Failure to readjust the window setting for Tc-99m prior to clinical use results in a poor resolution scan due to downscatter from 140 keV photons into the 122-keV window. This is referred to as “off-peak” imaging.
Poor camera positioning. Best image acquisition requires the gamma camera to be as close to the patient as possible. Minimizing the distance between source and detector allows for optimum performance of the external collimator, the largest limitation to overall resolution. As the detector is moved away from the target, photons with a greater angle of incidence will be allowed through the collimator because of the altered geometry. This leads to a blurry appearance of the obtained image. Using the wrong energy collimator could also similarly affect image quality.
Radiopharmaceutical preparation or administration error. Administration of an incorrect radiopharmaceutical, incorrect dose of radiopharmaceutical (>20% difference from prescribed dose), or utilizing an unprescribed route of administration are classified as “misadministration” and may account for unexpected imaging results. Technical errors in radiopharmaceutical preparation can lead to chemical or radionuclidic impurities that may result in image degradation and, in some cases, increased patient dose. Infiltrated dose can lead to inadequate accumulation in the organ of interest resulting in a poor target-to-background and quantum mottle (image noise).
Additional Considerations
Medical event: It is a subset of misadministration where the patient’s whole-body dose exceeds 5 rem (0.05 Sv) or a single-organ dose exceeds 50 rem (0.5 Sv) requiring verbal and written notification of the Nuclear Regulatory Commission (NRC).
Radiopharmaceutical quality: Regulations for radiopharmaceutical QC have been established by the NRC and the U.S. Pharmacopeia (USP). Examples for Tc-99m include less than 0.15 µCi of Mo-99 per 1 mCi of Tc-99m (at time of administration) and a maximum of 10 µg of aluminum break-through per 1 mL of Tc-99m eluate.
Diagnosis
Off-peak gamma camera.
✓ Pearls
Off-peak imaging results in poor resolution imaging due to improper radiotracer photopeak selection.
Poor resolution can also be seen with poor camera positioning and errors in radiotracer preparation/administration.
“Medical event” is the misadministration of radiotracer exceeding the allowable limits of radiation dose.
Suggested Readings
Cecchin D, Poggiali D, Riccardi L, Turco P, Bui F, De Marchi S. Analytical and experimental FWHM of a gamma camera: theoretical and practical issues. Peer J. 2015; 3:e722 Naddaf SY, Collier BD, Elgazzar AH, Khalil MM. Technical errors in planar bone scanning. J Nucl Med Technol. 2004; 32(3):148–153 Zanzonico P. Routine quality control of clinical nuclear medicine instrumentation: a brief review. J Nucl Med. 2008; 49(7):1114–1131Case 136
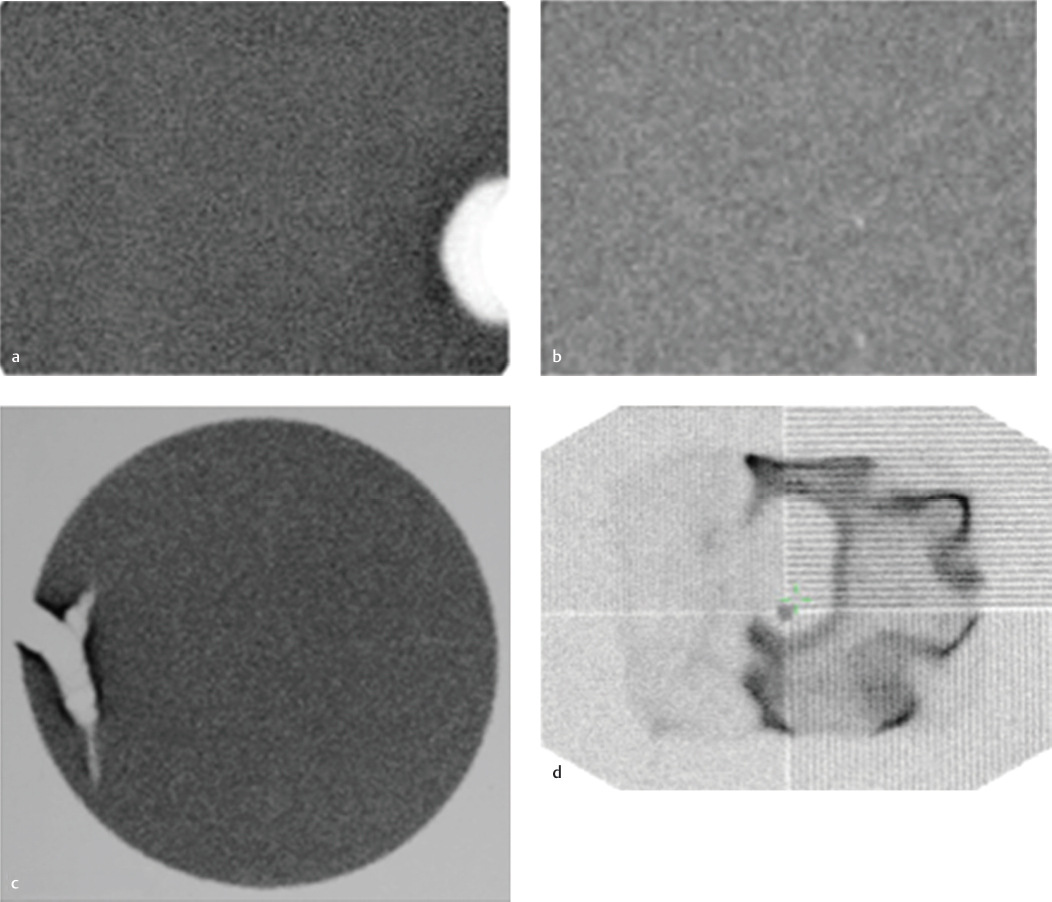
Key Finding
Focal defect on QC images
Top 3 Differential Diagnoses
PMT failure. An array of PMTs behind the sodium iodide crystal converts the light pulse from the scintillation event into an electrical signal. Malfunction of the PMTs can be caused by a damaged tube, decoupling of the gel between the crystal and PMT, or a loose electrical connection. Scintillation events occurring due to defective PMT will not result in a measurable electrical signal. A round defect will be seen in the field uniformity image, corresponding to the position of the malfunctioning PMT. A similar focal defect can also be caused by an overlying attenuating structure on the camera head or by an air bubble in the liquid flood phantom.
Crystal defect. Gamma ray photons produce a scintillation event within the sodium iodide crystal. A crack in the sodium iodide crystal on a flood-field image results in a branching white pattern due to lack of scintillations in this region. The surrounding dark edges are due to the edge-packing phenomenon.
Collimator defect. The collimator helps to localize the radionuclide in the patient by allowing only those photons that travel in an appropriate direction to interact with the crystal. The soft lead in the collimators can be dented resulting in bending and distorting of the septa. The defect appears as a focal area of decreased activity on the flood-field image. Repeating the flood image with the collimator removed will show resolution of the defect.
Additional Diagnostic Consideration
Electronic artifact: Complex computer circuitry mounted along each PMT calculates the position and strength of the scintillation event. Electronic malfunctions can result in various artifacts, depending upon which electronic system is involved. The findings on flood imaging are less characteristic than those seen with other flood-field artifacts.
Diagnosis
PMT failure (A); dented collimator (B); cracked crystal (C); electronic artifact (D).
✓ Pearls
Daily QC includes extrinsic flood with Co-57 sheet source placed on the camera with collimator.
Daily flood QC images should be reviewed if there is concern for external artifact on any diagnostic study.
Weekly spatial resolution/linearity is tested with a bar phantom between the Co-57 sheet and collimator.
Intrinsic flood (no collimator) and single-photon emission computed tomography (SPECT) QC are done monthly.
Suggested Readings
Mettler FA, Guiberteau MJ. Essentials of nuclear medicine imaging, 6th ed. Philadelphia, PA: Elsevier, 2012 Zanzonico P. Routine quality control of clinical nuclear medicine instrumentation: a brief review. J Nucl Med. 2008; 49(7):1114–1131 Ziessman HA, O’Malley JP, Thrall JH. Nuclear medicine: the requisites, 4th ed. Philadelphia, PA: Elsevier, 2014Case 137
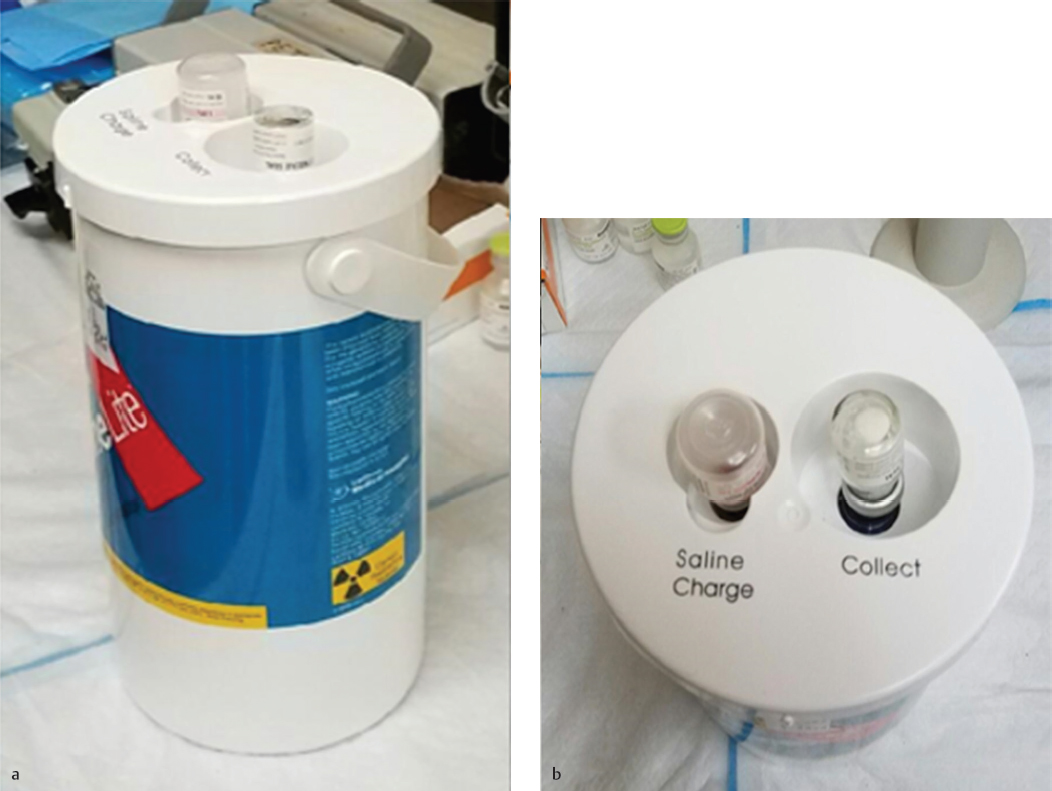
Clinical History
The nuclear medicine clinic has an Mo-99/Tc-99m generator shipped to the department on a weekly basis for providing readily available Tc-99m for imaging purposes. The technologist on shift elutes the generator each day to obtain a Tc-99m patient dose and administers it for a cardiac study. When the patient is placed on the scanner, the images acquired are not clinically usable (▶Fig. 137.1).
Key Finding
No clinically usable image can be obtained
Top 3 Differential Diagnoses
Wrong energy window. Before imaging a patient, the technologist can confirm that the energy window is centered on the correct photon energy with the correct energy window range. If the incorrect nuclide to be imaged is chosen on the scanner, it will be incorrectly throwing out counts from the Tc-99m 140 keV photons.
Wrong collimators. Most gamma cameras have an option to change the collimators in order to optimize image acquisition for the injected radionuclide. For Tc-99m, low-energy collimators are used. The definition for low, medium, or high energy is decided by the manufacturer, but it is correlated to the height and thickness of the lead septa within the collimator. If the collimators on the gamma camera are intended for a higher energy nuclide, it could take significantly longer time for an image to appear on the acquisition monitor and fully acquire.
Molybdenum-99 contamination. In accordance with 10 coronary flow reserve (CFR) 35.204, the first eluate from a Tc-99m generator is required to be tested to ensure that the Mo-99 contamination is not more than 0.15 µCi per 1 mCi of Tc-99m. Mo-99 has a half-life of 66 hours, primarily decays through a 1.214 MeV beta transmission as well as high-level gamma photons, and will concentrate in the liver, mineral bone, and kidneys if internalized. In addition to its radiation hazards, the high-energy gamma photons from Mo-99 could be a disruption to the imaging of Tc-99m uptake. The technologist should check that Mo-99 contamination levels are compliant.
Additional Diagnostic Consideration
Aluminum contamination: Generators should also be tested for any aluminum contamination in the eluate from the aluminum column contained in the generator.
Diagnosis
The technologist failed to check that the Mo-99 contamination level was within allowable limits. This failure to test and document the first eluate from a generator is a finding of noncompliance with 10 CFR 35.204 if identified by an inspector. At the time of writing this, the reporting of a generator that has failed the breakthrough test is not an Nuclear Regulatory Commission (NRC) reportable event but reporting requirements are being discussed. The unusable image from this patient was due to the Mo-99 contaminant level being beyond NRC thresholds with an activity of 2 to 3 mCi of Mo-99 for the patient administered dose of approximately 20 mCi Tc-99m. With this large Mo-99 dose being administered to the patient, the collimators on the gamma camera were not able to attenuate its many high-energy gamma photons. Furthermore, the energy spectrum analyzer of the gamma camera has an auto-ranging display setting that scales to its largest signal, so the Tc-99m signal was dwarfed by the Mo-99 energy spectrum component. This unexpected energy spectrum was not verified by the technologist and the image acquired included the many scattered Mo-99 photons.
✓ Pearls
The first eluate should not have Mo-99 levels above 0.15 µCi per 1 mCi of Tc-99m.
Technologists should verify the energy spectrum, energy level, and energy window width on a per-patient basis.
The Al3+ contamination test is no longer required by the NRC, but it is common practice to perform with each elution.
Suggested Readings
Pappas, Virginia, Re: Nuclear Regulatory Commission: 10 CFR Part 35 [NRC–2008–0175]; Medical Use of Byproduct Material, SNMI 18 November 2014 Shearer DR, Pezzullo JC, Moore MM, Coleman P, Frater SI. Radiation dose from radiopharmaceuticals contaminated with molybdenum-99. J Nucl Med. 1988; 29(5):695–700Case 138
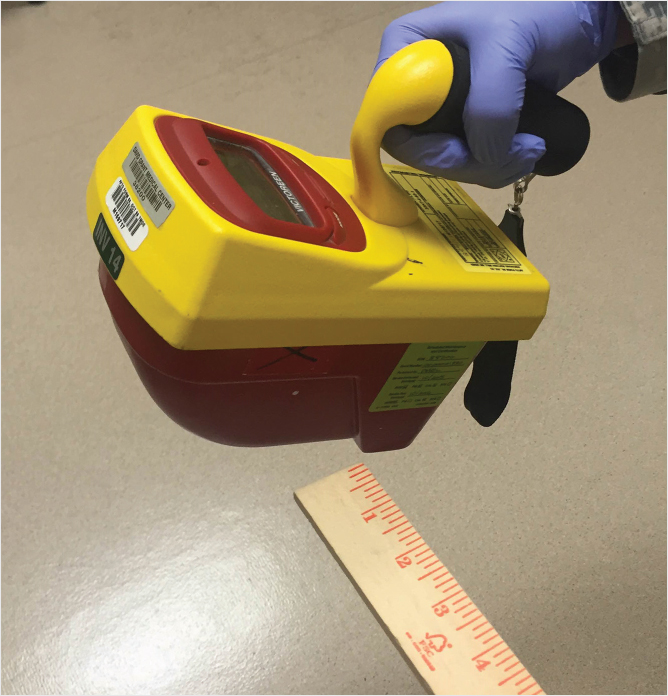
Clinical History
The radiation safety officer (RSO) takes surface and one-meter measurements from an inpatient who has received I-131 therapy. Based on calculations, the patient should be ready for release. The patient’s one-meter measurement is 17 mR/hour (▶Fig. 138.1).
Key Finding
Patient with higher than expected one-meter measurement of 17 mR/hour
Top 3 Differential Diagnoses
Ionization chamber measurement error. Prior to release from the facility, it must be determined that a patient who has been administered unsealed byproduct material will not overexpose a member of the public. In accordance with 10 coronary flow reserve (CFR) 35.75, which outlines patient release criteria, the total effective dose equivalent (TEDE) to a member of the public from a patient receiving unsealed byproduct material cannot exceed 500 mrem. Therefore, using calculations for I-131 taken from NUREG-1556 Volume 9 Revision 2, the dose rate at one meter at which a patient can be released is 7 mrem/hour (exposure rate of 7 mR/hour). Measurement occurs 1 m from the patient with an ionization chamber. In accordance with 10 CFR 35.61, survey instruments used for radiation surveys must be calibrated annually and “bump-checked” prior to use with a check source. If the indicated exposure rate differs from the anticipated exposure rate (determined during annual calibration) by more than 20%, then the survey meter must not be used.
Patient has compromised renal function. Patients with thyroid disease who receive I-131 treatment and have compromised renal function pose a challenge when it comes to radiation safety. The excretion rate of I-131 in these patients is significantly slowed. This can cause a delay in release from the facility due to inaccurate calculations using the typical effective half-life of I-131. Excretion of I-131 from the body can significantly vary from patient to patient; any I-131 calculations should be verified with measurements.
Incorrect distance for measurement. Due to the inverse square law, where intensity of the radiation beam decreases by 1/d2, where d is the distance from the source, the distance at which the measurement is taken can have a significant impact on the exposure rate measurement.
Additional Consideration
Public dose limits: Two other public dose limits exist: 100 mrem per year and 2 mrem in any 1 hour (i.e., 2 mR/hour) in an unrestricted area (10 CFR 20.1301). These dose limits exclude background radiation and exposure from individuals released under 10 CFR 35.75 as described above. To show compliance, record of radiation surveys for any areas where radioactive materials are stored, used, or prepared should be kept on file. If a member of the public is likely to receive a dose in excess of 100 mrem from a patient who has been administered unsealed byproduct material, the patient must be given written instructions outlining proper precautions and restrictions.
Diagnosis
The ionization chamber used for the release survey was giving exposure measurements 10 times higher than the actual levels. The chamber was sent out for repair.
✓ Pearls
Patients receiving unsealed byproduct material cannot give a member of the public a TEDE in excess of 500 mrem.
Release instructions must be given if a member of the public is likely to receive a TEDE in excess of 100 mrem.
Radiation intensity decreases by 1/d2, so the measurement distance is critical in showing that release criteria is met.
Suggested Readings
Lee JH, Park SG. Estimation of the Release Time from Isolation for Patients with Differentiated Thyroid Cancer Treated with High-dose I-131. Nucl Med Mol Imaging. 2010; 44(4):241–245 Ravichandran R, Binukumar J, Saadi AA. Estimation of effective half life of clearance of radioactive Iodine (I) in patients treated for hyperthyroidism and carcinoma thyroid. Indian J Nucl Med. 2010; 25(2):49–52Case 139
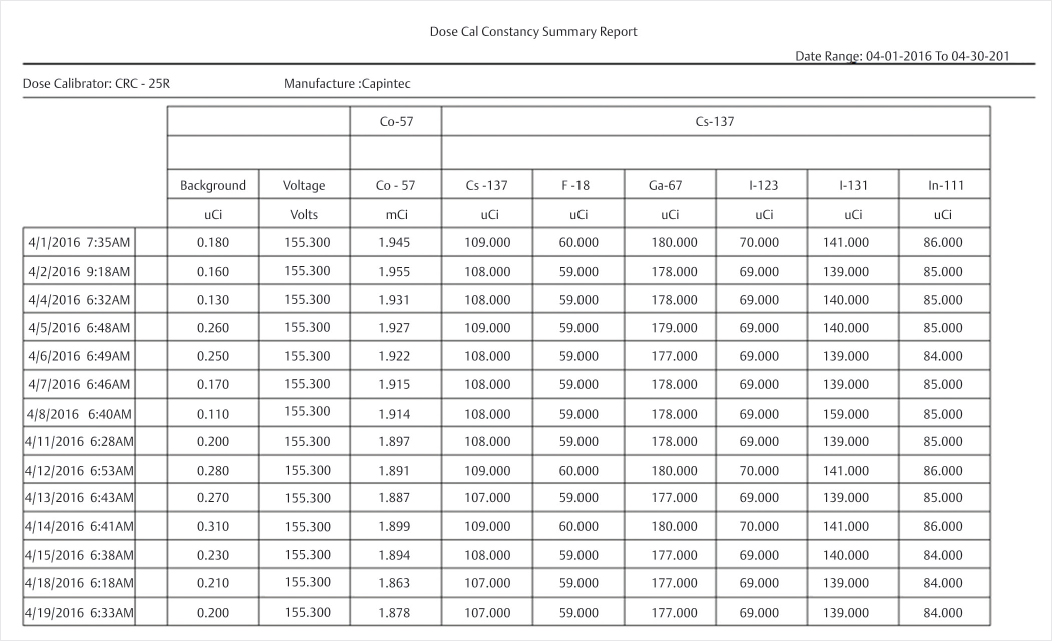
Clinical History
The RSO reviews dose calibrator QC records for compliance with the Nuclear Regulatory Commission (NRC) (▶Fig. 139.1).
Key Finding
Errors with records of dose calibrator QC
Top 3 Differential Diagnoses
Constancy. At installation and prior to daily use, the dose calibrator constancy test should be performed in order to determine that the precision of the instrument is within the permissible range (±10%), and that it is performing consistently from day to day. Constancy is typically performed with a Cs-137 check source, and the activity is measured on multiple radionuclide settings (gain settings) on the dose calibrator. One example would be checking the I-123 gain setting using a Cs-137 check source because Cs-137 is relatively long-lived and will provide constant measurement values each day in a properly functioning dose calibrator.
Linearity. Linearity should be performed on the dose calibrator at installation and at every quarter to ensure that it is providing an accurate reading across a wide range of activities, from µCi to mCi. There are two ways to perform linearity: the decay method and the sleeve method. The decay method involves placing a vial of radiopharmaceutical in the dose calibrator and taking readings at various time intervals to plot the measured activity versus time on a semi-logarithmic graph, thus producing a linear representation of the decayed activity. The sleeve method uses cylindrical sleeves of increasing lead thickness to simulate the decay of the source. These values could also be plotted on a semi-log graph to produce a linear response but are more commonly corrected with calibration factors and compared. Measurements must be within ± 10% of the expected value.
Accuracy. At installation and annually after, accuracy should be performed on the dose calibrator using a National Institute of Standards and Technology (NIST) source to determine that the dose calibrator is providing an accurate measurement of activity for a range of energies, within ±10% of the known activity value. Typically, Co-57, Ba-133, and Cs-137 are used to test low-, medium-, and high-energy ranges, respectively.
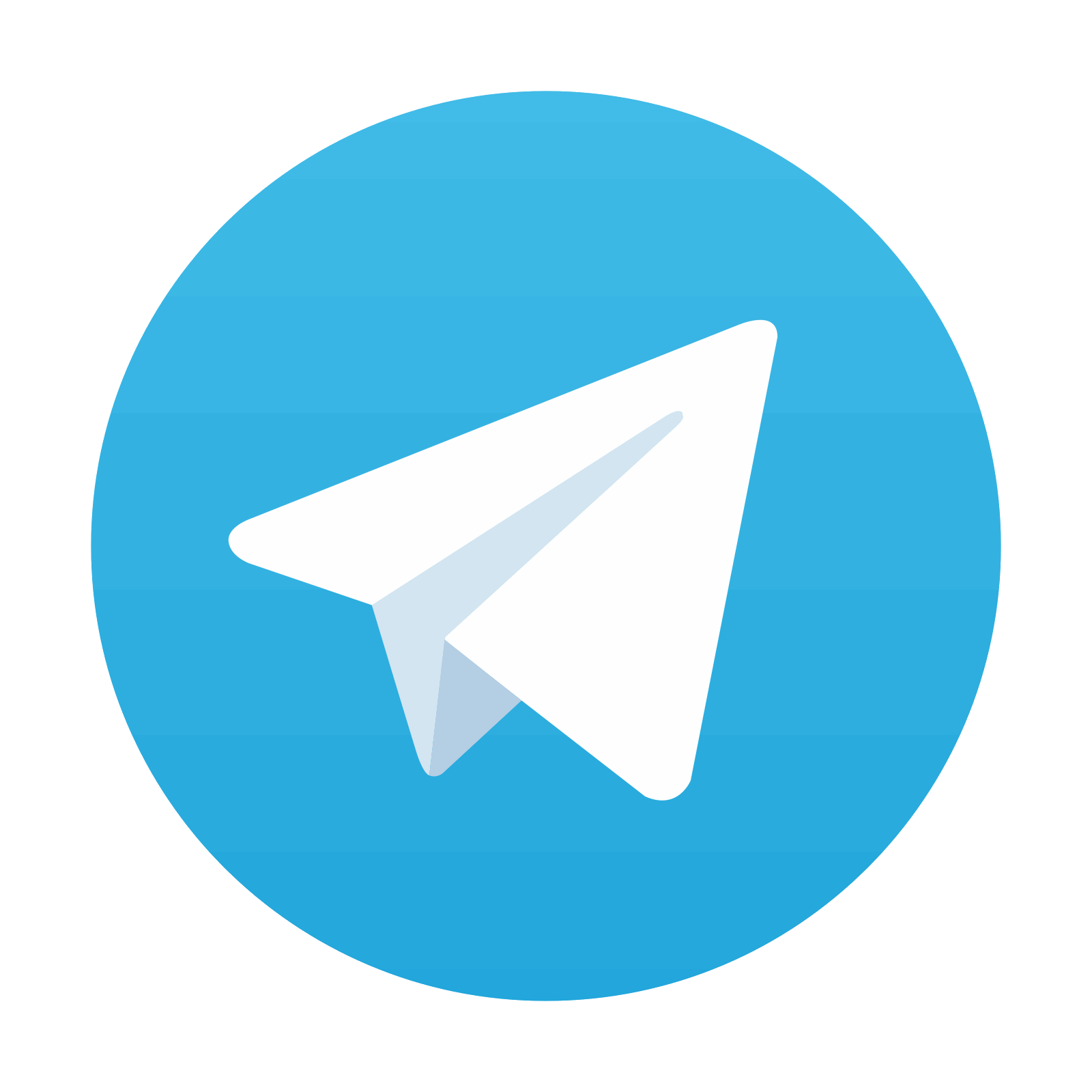
Stay updated, free articles. Join our Telegram channel

Full access? Get Clinical Tree
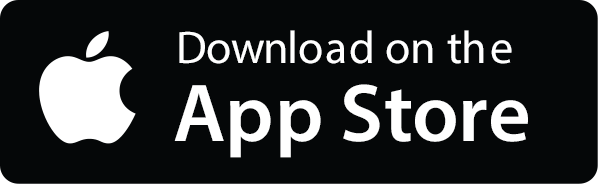
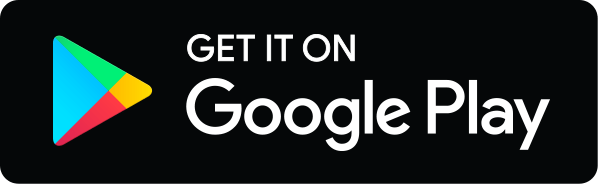
