Fig. 3.1
18F-FDG PET is predictive of the outcome: patients with a highly metabolic active primary tumor show a significantly shorter overall survival. A 39-year-old patient with a high-grade malignant schwannoma in the right thigh (arrow, a + a’). SUVmax on the baseline 18F-FDG PET scan was 9.75. Within 6 months after resection of the primary lesion, 18F-FDG PET showed local recurrence (arrow, b), as well as metastases in the right lung (open arrow, b’) and in soft tissue (double arrow, b”)). Follow-up 18F-FDG PET showed progressive disease with the development of pleural (double arrow, c’), bone (open arrow, c’), and lymph node metastases (arrow, c”)
After correction for standard clinical prognostic factors such as histology, grade, age, and sex, SUV was found to be an independent predictor of survival and disease progression [14]. Also the heterogeneity in tissue uptake of 18F-FDG was found to be a strong predictor of the final outcome [15].
Since STS tend to be large and heterogeneous, there is a risk of sampling error when taking a biopsy. In the previous studies, diagnostic discrepancies in malignancy grade were found in about 10 % of cases when determined by fine-needle aspiration (FNA) and core-needle biopsy (CNB) compared with final histologic diagnosis on the resection specimen [11]. 18F-FDG accumulates in the cells in proportion to the rate of glucose metabolism, giving information on biological activity of the tumoral parts, and subsequent the identification of intratumoral heterogeneity, reflected as areas of high and low SUV (see also Fig. 3.2). In a study of Rakheja, a close correlation was not only found between SUVmax and mitotic count but also with the presence of tumor necrosis [44]. By identifying the most metabolically active portion of a tumor mass, nuclear medicine techniques can guide biopsy to a site most likely to contain tumor tissue of the highest grade present.
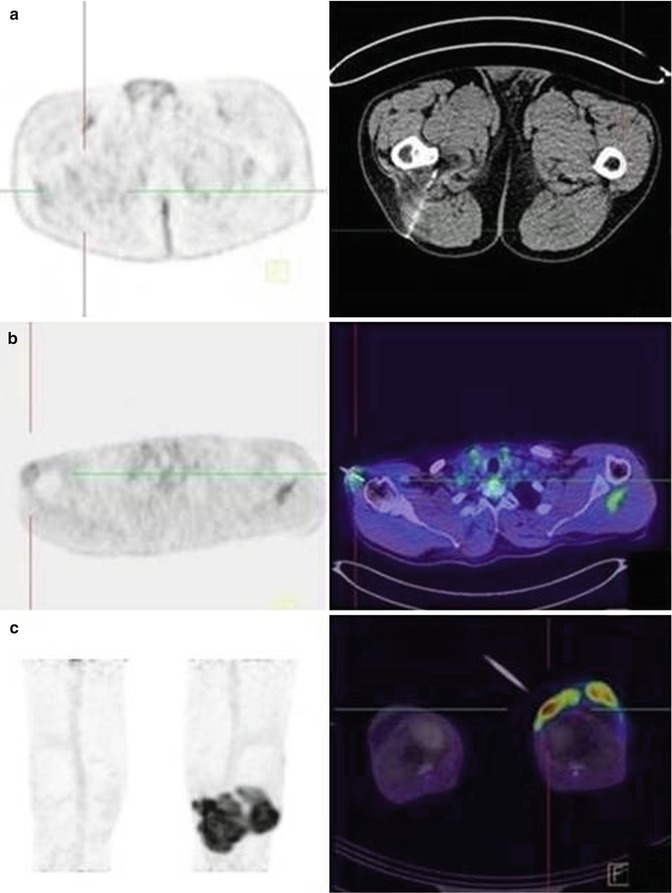
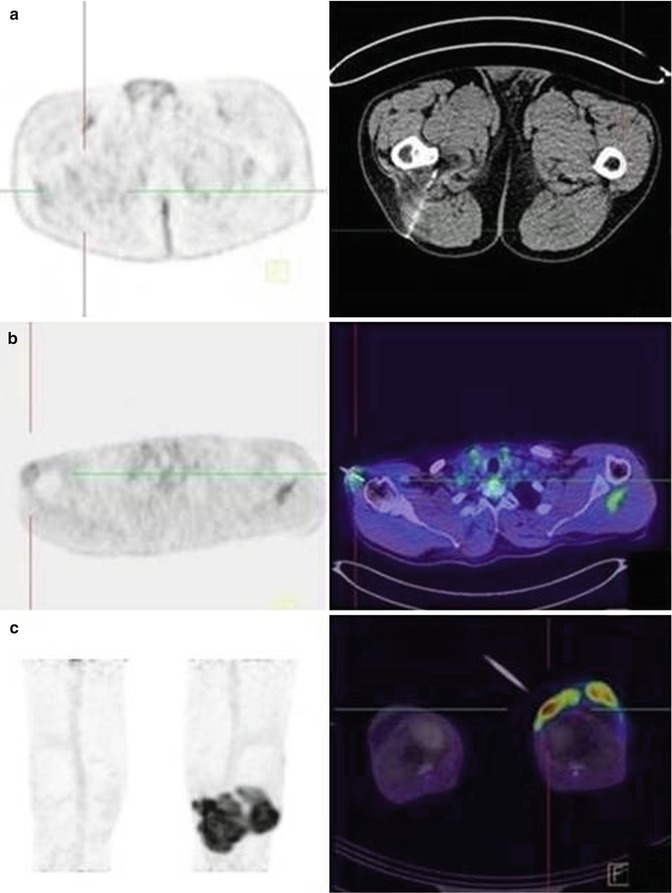
Fig. 3.2
18F-FDG accumulates in the cells in proportion to the rate of glucose metabolism, giving information on biological activity of the tumoral parts, and subsequent the identification of intratumoral heterogeneity, reflected as areas of high and low SUV. By identifying the most metabolically active portion of a tumor mass, nuclear medicine techniques can guide a biopsy to a site most likely to contain tumor tissue of the highest grade present. From our own database: a biopsy of a lesion under 18F-FDG PET guidance. A lipoma, showing no 18F-FDG uptake (a). A low-grade myxofibrosarcoma of the right shoulder showing only a discrete and homogenous 18F-FDG uptake (b). A high-grade myxofibrosarcoma of the left knee with an intense and heterogeneous 18F-FDG uptake (c)
In conclusion, the main role of the current imaging modalities is to recognize patients with typically benign disease, in whom further invasive staging can be omitted, and select those patients with a suspected malignancy, who should be referred for biopsy. Following appropriate imaging assessment, biopsy of the mass completes the staging process. Since STS tend to be large and heterogeneous, there is a risk of sampling error; imaging can help to guide the biopsy toward the most aggressive zone.
3.3 Evaluation of Disease Extent
As a result of its clear, detailed images of soft tissues, MRI represents an ideal imaging modality to evaluate the anatomical compartments being affected by a primary tumor originating in the mesenchymal tissues of muscles, fat, blood vessels, nerves, tendons, and synovial tissues and its relation to adjacent structures.
Approximately 20 % of all patients with a sarcoma will have metastatic disease at initial diagnosis, 75–80 % of the metastases arising intrathoracic [42, 46]. Völker et al. evaluated the impact of 18F-FDG PET for initial staging and therapy planning in 46 pediatric patients [57], demonstrating that 18F-FDG PET is a valuable tool for initial staging and additionally having a significant impact on therapy planning. In this study, 18F-FDG PET was clearly superior to conventional imaging modalities (CIMs) – including ultrasound, computed tomography (CT), magnetic resonance imaging (MRI) and bone scintigraphy – in the detection of lymph node involvement (sensitivity, 95 % for PET versus 25 % for CIMs, respectively) and bone lesions (sensitivity, 90 % versus 57 %, respectively). These findings are in accordance with other studies showing a higher sensitivity and specificity for 18F-FDG PET-CT in detecting malignant lesions than CIMs when excluding lung metastases (83 %, 98 % and 78 %, 97 %, respectively) [33].
With regard to the detection of lymph node metastases, the power of 18F-FDG PET exists in its ability to show tumoral involvement in normal-sized nodes and to exclude disease in reactively enlarged nodes [13]. In view of the fact that lymphatic spread is presumed rare in the natural course of STS, 18F-FDG PET is currently considered useful only in tumor types with a higher incidence of lymphatic spread (angiosarcoma, epithelioid sarcoma, and rhabdomyosarcoma). However, it is possible that with the growing use of 18F-FDG PET-CT, the prevalence of detectable lymph node metastases may increase [53].
In these same studies as mentioned above, 18F-FDG PET-CT had a higher specificity than CIMs in lung lesions (96 % compared to 87 %) but a lower sensitivity (80 % compared to 93 %), most likely due to a combination of factors such as lung lesions with a size below the limits of resolution of 18F-FDG PET (i.e., 4–5 mm), partial volume effects, and breathing motion [42, 46]. High-resolution CT remains the most sensitive imaging modality to detect lung metastases, although it was shown to be only cost-effective in patients with high-grade T2 lesions or T2 extremity lesions [41]. The higher specificity of 18F-FDG PET-CT emphasizes the complementary roles of these imaging modalities in the assessment of lung lesions [57].
Regarding the detection of bone lesions, whole 18F-FDG PET and bone scanning seem superior to conventional CIMs, although one should take into account the type of the primary lesion. Most of these studies are done in patients with bone sarcoma, showing a comparable or a clearly superior sensitivity of 18F-FDG PET and bone scanning compared to CIMs in patients with osteosarcoma and Ewing sarcoma, respectively [33, 57]. However, since tumor spread to bones is rare in STS, the use of bone scintigraphy seems only to be encouraged in symptomatic patients [28].
Liver metastases may be the first sign of dissemination in primary intra-abdominal STS and are usually visualized on the abdominal CT performed to evaluate the primary mass. To the best of our knowledge, no specific literature is available on the accuracy of PET for the detection of liver metastases in sarcoma. Several groups reported on the value of 18F-FDG PET, 18F-FDG PET-CT, and/or subsequent MRI for PET-MRI fusion for the detection of liver metastases in different kinds of tumors [3, 9, 12, 45]. All of them found a high sensitivity and accuracy for 18F-FDG PET, 18F-FDG PET-CT, as well as combined with subsequent MRI for PET-MRI fusion.
Although clinical experience with 18F-FDG PET/MRI is limited, first results seem promising. The main advantages of this one stop shop approach lies in combining the strength of the T staging equal to that of an MR alone, complemented by the N and M staging from the PET component [38].
In summary, the main power of 18F-FDG PET and 18F-FDG PET-CT lies in the ability to screen the entire patient for distant metastases without significantly increasing the radiation burden. The key role of 18F-FDG PET-CT lies largely in detecting metastases at unexpected sites, outside the standard field of view of CT and MRI, and in the exclusion of disease in equivocal results on conventional imaging. Although further studies are required, preliminary clinical experiences with 18F-FDG PET-MRI seem promising.
3.4 Response Evaluation
The purpose of therapy monitoring is to provide an early and still accurate differentiation between responders and nonresponders with the ultimate goal of adapting therapy to the information provided. In this way, responders can continue treatment, whereas therapy can be adapted in those patients in whom there is a lack of response.
Response to treatment in solid tumors is usually defined as a significant decrease in tumor dimensions by anatomic imaging techniques. In version 1.0 of the Response Evaluation Criteria in Solid Tumors (RECIST), partial response was defined as a decrease of at least 30 % in the sum of diameters of the target lesions, with the baseline sum of diameters as reference. However, these criteria are not always capable of distinguishing responders from nonresponders to neoadjuvant therapy [5, 18, 26, 50].
There are weaknesses in the evaluation of tumor response by volume changes, especially in STS. Firstly, precise measurement of tumor dimensions can be challenging in ill-defined lesions like bone, bowel and peritoneal lesions. Secondly, chemotherapy can induce profound tissue changes like necrosis and fibrosis [35]. As a result of this, a reduction in viable tumor cell fraction is not always associated with a reduction in volume. Moreover, volume changes are rather late events and therefore changes measured by computer tomography are often not significant when performed earlier than two to three months after the start of treatment. In this way, patients can be unnecessarily exposed to ineffective, toxic, or expensive treatments during a prolonged period. It can even reduce the chance of a curative resection by postponing surgery in the neoadjuvant setting. Finally, the goal of new anti-vascular and cytostatic agents is rather a stabilization of tumor growth than tumor shrinkage. Therefore, no major volume changes are to be expected. Recently the RECIST criteria have been revised (version 1.1) [16] to overcome these limitations, but clinical validation of these newer approaches is still required.
Several studies have shown promising results for 18F-FDG PET in treatment monitoring. In various tumors, such as breast cancer, lymphoma, esophageal, stomach, colorectal, head and neck and non-small-cell lung cancers, a good correlation between an early and significant decline in metabolic activity and response to therapy was found, as well as a good correlation between the early decrease in 18F-FDG uptake and patient outcome [36]. Since 18F-FDG PET can differentiate between viable cells and necrotic or fibrotic inactive tissue, one can tell apart responders and nonresponders early after the start of treatment [4, 49].
Evilevitch et al. evaluated whether changes in 18F-FDG uptake and size measured by a combined PET-CT scan before and after neoadjuvant chemotherapy in high-grade soft tissue sarcoma could accurately assess histopathologic response defined as ≥95 % pathologic necrosis on the resected tumor. Quantitative 18F-FDG PET outperforms the size-based criteria to distinguish responders from nonresponders to neoadjuvant therapy. Using a 60 % decrease in 18F-FDG uptake resulted in a sensitivity of 100 % and specificity of 71 % for PET, whereas for RECIST a sensitivity of 25 % and specificity of 100 % was seen [18].
Schuetze and coworkers measured 18F-FDG uptake before and after neoadjuvant chemotherapy in 46 patients with high-grade localized sarcomas. They found a significant correlation between the change in the maximum standardized uptake value (SUVmax) and the amount of residual viable tumor in the resected specimens. 18F-FDG was found to be a valuable tool to predict outcome, in this way that a SUVmax higher than 6 at baseline and less than 40 % decrease in 18F-FDG uptake were associated with a high risk for systemic disease recurrence, whereas patients with a ≥40 % decline in SUVmax after chemotherapy were at a significantly lower risk for recurrent disease and death after complete resection and adjuvant radiotherapy [47] (see also Fig. 3.1). A study of Fendler likewise reported PET response, determined by changes in peak SUV (SUVpeak) from baseline to follow up in accordance with modified PET Response Criteria in Solid Tumors (PERCIST 1.0), to be an independent predictor of progression-free survival as well as for local and distant progression in STS patients after 2–4 cycles of chemotherapy in combination with regional hyperthermia [19].
Clinical trials in gastrointestinal stromal tumors (GISTs) show a significant improvement in relapse-free survival and overall survival in high-risk patients treated with the molecular-targeted therapy imatinib as adjuvant treatment for 3 years [29], as well as a significant increase in progression-free survival and overall survival compared in locally advanced inoperable and metastatic patients compared to historical controls [10, 55].
Although tumor response occurs within a few days after start of treatment in responders, objective tumor shrinkage is minimal and usually occurs only after several weeks or months. Moreover, since tumor tissue can be replaced by necrotic or fibrotic tissue, a reduction in the viable tumor cell fraction is not always reflected by a volume reduction. Metabolic imaging with 18F-FDG PET has proven to be highly sensitive in detecting early tumor response to Glivec treatment and precedes tumor shrinkage by several weeks to months. A complete normalization of all hypermetabolic foci is seen within 1 week of treatment and is predictive for favorable outcome [52, 54]. Since RECIST underestimates the effect of imatinib on metastatic GIST, especially at the early stage of treatment, and is a poor predictor of clinical benefit, CT criteria were reevaluated. Given that a cystic-like appearance with “near”-fluid attenuation and loss of contrast enhancement is the typical appearance of responding lesion on CT, the use of Hounsfield unit (HU) measurements is proposed as an alternative response marker [1]. Using the CHOI criteria, where response is defined as a decrease in tumor size of more than 10 % or a decrease in tumor density of more than 15 %, the prognostic value of these new CT response criteria is comparable to the one obtained with 18F-FDG PET [6].
Once GISTs have responded to therapy, treatment should be continued ad infinitum, since interruption of treatment usually gives rise to a relatively rapid tumor progression. Thus, monitoring of tumor response should be done in the early phases of treatment, whereas follow-up should be continued throughout the treatment, due to a persistent risk of secondary progression over time [17].
In conclusion, 18F-FDG PET seems promising in treatment monitoring. A good correlation was found between an early and significant decline in metabolic activity and response to therapy in different types of sarcoma. Furthermore, 18F-FDG uptake is an independent predictor for overall and disease-free survival.
3.5 Detection of Recurrence
Although recurrences predominantly develop within 2 years of resection of the primary tumor, late recurrences (>5 years after treatment) are not uncommon [31, 40]. Main predictors for local recurrence are positive surgical margins, whereas grade, size, and tumor-node-metastasis (TNM) stage predict the development of metastatic disease and overall survival [51]. Early detection and treatment of local recurrence is desirable if surgical control is to be achieved, even if this does not necessarily influence the final outcome of the patient.
As a result of its high-contrast resolution, MRI is currently the technique of choice for the evaluation of suspected local recurrence in the extremities. However, radiotherapy- and chemotherapy-induced changes such as soft tissue trabeculation, increased fatty marrow, focal marrow abnormalities, and hemorrhage can complicate the evaluation of the affected region. On the other hand, the use of an organized systematic approach and certain algorithms can reduce the challenge [25, 56].
Although detection of local recurrence of STS is still a rather controversial indication for 18F-FDG PET-CT, preliminary results for the use of PET-CT for this purpose in bone sarcomas [43, 48], as well as STS, seem promising [7]. However, further studies are necessary.
Conclusion
Since no imaging technique can surpass pathology in the purpose of determining exact histology and grading of the primary tumor, the main role of the current imaging modalities is to recognize patients with typically benign lesions, in whom no further invasive staging is warranted, and to select those patients who should be referred for biopsy. In view of the fact that sarcomas can be large and heterogeneous, nuclear medicine techniques can guide a biopsy to a site most likely to contain tumor tissue of the highest grade present.
In most cases, conventional radiography, MRI, and CT still remain the first-line imaging modalities in the diagnostic workup of sarcoma. Due to its limited spatial resolution and its weak anatomical detail, 18F-FDG PET as a standalone technique is of no use in the evaluation of the local extent of the tumor. However, we have entered a new era of hybrid imaging machines, uniting the anatomical information of the CT and/or MR and the metabolic information of the PET, improving diagnostic accuracy, providing surgery and radiation therapy planning, and by merging all necessary information in one single procedure guiding biopsies even better. Still, studies are required to evaluate the impact of combining these imaging techniques on the overall diagnostic performance in sarcoma.
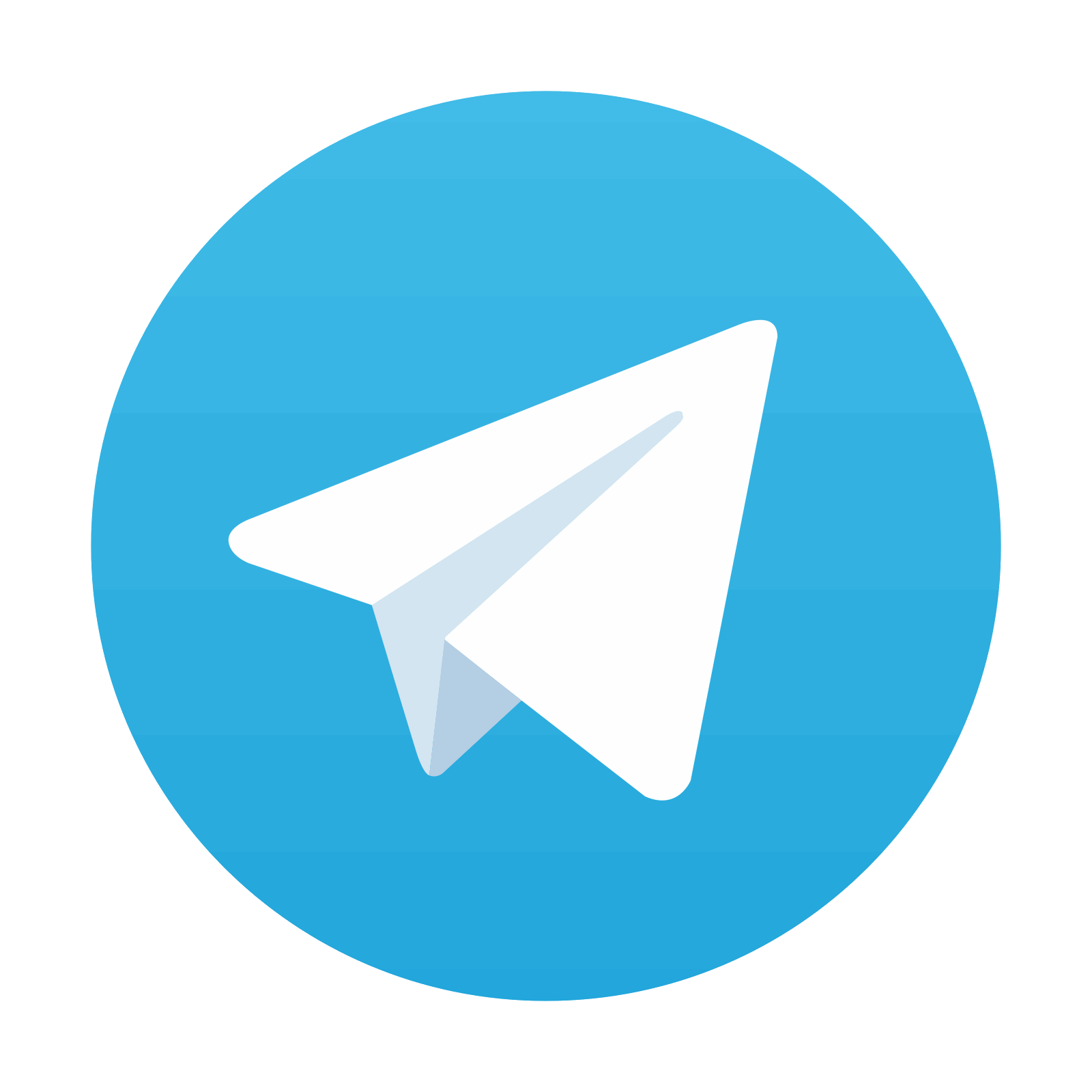
Stay updated, free articles. Join our Telegram channel

Full access? Get Clinical Tree
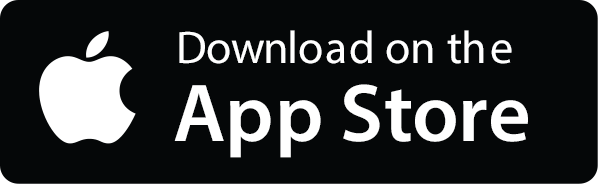
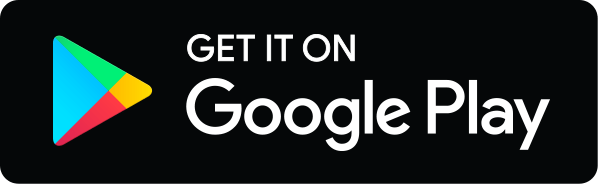