© Springer International Publishing Switzerland 2016
Luigi Mansi, Egesta Lopci, Vincenzo Cuccurullo and Arturo Chiti (eds.)Clinical Nuclear Medicine in Pediatrics10.1007/978-3-319-21371-2_22. PET/MR in Children
(1)
SDN Foundation, Institute of Diagnostic and Nuclear Development, Naples, Italy
(2)
Department of Advanced Biomedical Sciences, University Federico II, Naples, Italy
2.1 Introduction
The rapid increase in incidence of diagnosed malignant diseases in children over the last decades, combined with innovations in molecular oncology, neuroimaging, and hybrid imaging, has encouraged researchers and physicians to make a special effort in optimizing technological resources to approach pediatric patients using high-resolution imaging devices with concern about radiation exposure. In this context, a truly hybrid imaging tool, such as simultaneous positron emission tomography/magnetic resonance (PET/MR), presents the appealing advantage to combine serial imaging technology (MR) and a volumetric (PET) method, at the same time under the same conditions, to define and to assess a pathophysiological pattern for each disease in every single patient aiming to customize therapeutic strategy, therefore improving survival rate. Furthermore, a simultaneous approach enables to overcome some of the limitations of current PET/computed tomography (CT) scan, such as misregistration of attenuation (CT) and emission (PET) images due to spatial and temporal mismatch between CT and PET acquisitions, thus reducing artifactual false-positive result percentage. In addition, the possibility of matching two powerful modalities such as MR and PET opens the way for new challenging clinical applications for disease characterization that are currently under investigation, e.g., multiorgan disorders. In this chapter we will focus on the potential clinical role of PET/MR in pediatric diseases.
2.2 Available Diagnostic Tools in Pediatric Diseases
Nuclear medicine procedures are able to address several childhood diseases in the manner of diagnosis, treatment planning, monitoring, and follow-up by using well-established diagnostic methods. Thanks to advanced single-photon emission CT (SPECT) and PET systems available and state-of-the art CT and MR devices combined with a wide range of radiopharmaceuticals and contrast agents developed, most childhood-specific disorders can be accurately studied. In particular, radionuclide techniques with the use of gamma camera have been widely validated and applied to investigate the kidney and urinary tract in children by using three categories of 99mTc-labeled radiopharmaceuticals: diethylene triamine pentaacetic acid for glomerular filtration rate measurement, mercaptoacetyltriglycine for tubular secretion assessment, and dimercaptosuccinic acid for evaluation of tubular retention. Bone scintigraphy with 99mTc-methylene diphosphonate as specific tracer is a valuable tool for identification of alterations of bone metabolism derived from benign or malignant pathologies. In addition, the recent introduction of 18F-sodium fluoride as PET tracer allows the achievement of higher-resolution images with similar dosimetry for pediatric bone malignancies evaluation. Regarding to neuroimaging, brain tumors and epilepsy can be investigated with MR and the support of SPECT or PET techniques by using 99mTc-ethyl cysteinate dimer, 99mTc-hexamethylpropylene amine oxime for SPECT, and 18F-fluorodeoxyglucose (FDG) for PET. Other brain PET receptor tracers have also been introduced, such as serotonin markers (5-HT1A, MPPF (4-(2’-methoxyphenyl)-1-[2’-[N-(2”-pyridinyl)-pfluorobenzamido]ethyl]piperazine), dopamine system receptors [18F]-fluoro-L-dopa, [18F]-fallypride), glutamate/NMD receptors (11C-S-ketamine, 11C-CNS 5161), and opiate receptors (11C-carfentanil). However some limitations, such as commercial availability, restrict their use in clinical practice. As regards imaging in oncology, in the last decades FDG-PET/CT has emerged as valuable method for metabolic characterization of hematologic and solid malignancies. Qualitative image evaluation combined with standardized uptake value assessment is a validated approach for staging, assessing response to therapy, and detecting disease recurrence. Novel developed tracers, such as 11C-methionine and 18F-fluorothymidine for gliomas, 18F-dihydroxyphenylalanine (DOPA), 68Ga-DOTANOC, and other analogues for neuroendocrine tumor investigation, may increase the accuracy of method.
2.3 PET/MR in Pediatric Patients
2.3.1 Neurological Disorders
2.3.1.1 Epilepsy
According to the last World Health Organization report (WHO fact sheet 999 October 2012), around 50 million people worldwide suffer from epilepsy. Therefore, the estimated proportion of the general population with active epilepsy (i.e., continuing seizures or the need for treatment) is between 4 and 10 per 1000 people. The most common type is idiopathic epilepsy with unknown etiology. Secondary epilepsy is caused by brain damage from prenatal or perinatal injuries, congenital abnormalities, or brain infections such as meningitis, encephalitis, or a brain tumor. Children with medically intractable epilepsy can be considered as candidates for surgery. However, the success of a surgical approach strongly depends on the precise presurgical identification of epileptogenic foci, for which MR is the most reliable tool [1–4] with T1 acquisition for imaging anatomy and various T2 sequence acquisitions for detecting tissue pathology, such as fast low-angle inversion recovery and gradient recalled echo. In addition, several details may be measured with ~1 mm of spatial resolution using a whole-brain T1- or T2-weighted MR scan, such as local gray matter volume, cortical thickness, and sulcal depth. The integration of provided information results in an extremely sensitive and specific tool (97 % and 83 %, respectively ) for a deep investigation of epilepsy. Nevertheless, a number of patients with temporal lobe epilepsy do not show any suspected lesion on MR scans (nonlesional epilepsy). In the pediatric population, this is a common occurrence because of the higher frequency of cortical dysplasia [5] that often shows false-negative on MR scans [6, 7].
FDG-PET has been shown to achieve high detectability regarding temporal lobe epilepsy seizure focus, with a decreased glucose uptake in the epileptogenic temporal lobe [8], and better surgical prognosis has been reported in cases with FDG-PET hypometabolism. In addition interictal PET with FDG can identify those brain malformations of cortical development that are invisible to MR but are confirmed histologically post-operation [9, 10]. Therefore, PET may have a clinical role in pediatric epilepsy practice [11], revealing abnormalities otherwise difficult to detect. PET sensitivity may increase by using specific statistical analysis methods, such as statistical parametric mapping, and by PET/MR co-registration. The ability of PET/MR to delimit the anatomic boundaries of hypometabolic areas should be noted, as this can help stereotactic neuronavigation-guided surgery [12]. The anatomical definition of the hypometabolic area suggests PET/MR as a useful tool for a complete and accurate resection. PET/MR as a pre-operatory modality has shown the same accuracy of PEt alone in detecting hypometabolic areas in pediatric nonlesional patients, showing a good concordance with electro-clinical data [12]. Postsurgical outcomes of patients supported the usefulness co-registered PET/MR images in neuronavigation systems. Furthermore, especially in younger children, the number of episodes and the length of sedation or anesthesia may be reduced when the procedures are performed simultaneously [13].
2.3.1.2 Tuberous Sclerosis Complex
Tuberous sclerosis complex is an autonomic-dominant neurocutaneous syndrome with multiorgan impairment. In the brain, subependymal giant-cell astrocytomas, subependymal nodules, and tubers are characteristic findings. With an early onset at infancy age, patients may develop intractable epilepsy in childhood due to single tuber. Given the medically refractory nature of the epilepsy, surgery should be considered for such cases. An accurate preoperative assessment of the culprit tuber is therefore necessary to guide a successful resection of the epileptogenic tuber. In this context a multimodal approach with FDG-PET/MR may lead to a detailed presurgical evaluation using a diffusion-weighted MR approach supported by interictal hypometabolism of FDG-PET in the region of detected tuber.
2.3.1.3 Brain Tumors
Central nervous system tumors are the most frequent solid neoplasm in children [14–16] accounting for 20 % of all malignancies [17] and for the majority of pediatric cancer deaths [18]. Among these, benign gliomas, primitive neuroectodermal tumors, and craniopharyngiomas account for the majority of brain tumors in children. Therefore, there is great interest in advanced imaging modalities for diagnosis, staging, and treatment monitoring of brain tumors in the pediatric population.
In the evaluation of central nervous system tumors, MR imaging with high soft-tissue resolution, multiplanar capability, and lack of ionizing radiation allows the determination of tumor size, location, and its characterization, but its use is limited in tumor physiology assessment and demonstration of tumor progression and recurrence. Contrast enhancement with MR imaging, a common finding in high-grade tumors, could be related to surgery and/or radiation therapy, thus misleading patient follow-up. On the contrary, PET can provide quantitative assessment of functional and metabolic changes of the tumor tissue providing additional information to estimate tumor proliferative activity. A large variety of protocols including dynamic contrast-enhanced imaging, diffusion imaging, functional MR imaging, T1 permeability, and MR spectroscopy coupled with the exceptionally high sensitivity of PET in the picomolar range allow PET/MR to provide anatomic, functional, and quantitative information [19]. Therefore, combined PET/MR is expected to improve diagnostic accuracy and to help guided biopsy, surgery, or radiation therapy planning through the precise localization of hypermetabolic, vital tumor tissue and the accurate definition of the target volume. Furthermore, the simultaneous acquisition of PET and MR data provides precise information about tumor biology and the tumor response after antitumor therapy [13].
FDG-PET is very sensitive in detecting poorly differentiated tumors such as high-grade gliomas and cerebral lymphoma. On the other side, PET imaging can be confounded by the high uptake normally seen in the cortex, resulting in less accurate detection of low-grade gliomas [20]. However, a variety of non-FDG-PET tracers with oncological brain applications have been developed. 11C-methionine, O-(2-18F-fluoroethyl)-L-tyrosine, and 18F-DOPA have higher accuracies compared with FDG for the detection of low- and high-grade gliomas and for assessment of the treatment response because of better tumor-to-nontumor ratio [21–24].
PET with methionine has been documented for the first time to be capable of diagnosing brain tumors that are histologically and/or anatomically characteristic in children and substantially different from the predominantly astrocytic tumors previously studied in adults. The intensity of amino acid uptake can be assessed reliably, even by qualitative inspection of the image, and appears to reflect the histological grade and malignancy of tumors [25].
2.3.2 Oncological and Hematological Disorders
2.3.2.1 Lymphoma
PET/CT imaging is well established as a valuable tool in pediatric oncology for diagnosis and follow-up. The greater value offered by MR compared to CT for pediatric oncologic studies due to high soft-tissue resolution [26] combined with reduced radiation exposure encouraged the introduction of PET/MR in children. Non-Hodgkin and Hodgkin lymphomas account for about 10 and 18 % of all pediatric tumors. Malignant lymphomas are staged using the Ann Arbor staging system, except for childhood non-Hodgkin lymphomas that are staged using the Murphy staging system [27, 28]. The use of FDG-PET for staging and treatment planning has been widely validated [29] above all for Hodgkin and high-grade non-Hodgkin lymphomas with sensitivity of 95 % and specificity of 99 % [30]. In addition, PET imaging shows great value in evaluation of response to therapy monitoring, allowing residual mass characterization as fibrosis or active disease [29]. On the other hand, whole-body MR imaging techniques and particularly whole-body diffusion-weighted imaging may be a good radiation-free alternative to CT with high sensitivity for the detection of lesions with a sensitivity of about 96 %. This method provides anatomical information about tumor site and tissue characterization and correctly identifies the biopsy site, essential for diagnosis and treatment planning. In addition, short TI inversion recovery approach allows detection of parenchyma and bone marrow lesions that show high signal intensity. The major weakness of MR imaging is its low specificity for evaluation of post-therapeutic changes due to persisting bone marrow edema, necrotic tissue, and contrast enhancement in successfully treated lesions especially in children with variations of bone marrow appearance related to age leading to misinterpretation [31]. The visualization of both nonmalignant and malignant lymph nodes has been described as another important limitation of functional MR diffusion-weighted imaging method. A combined approach by using hybrid PET/MR may overcome most limitations of these two modalities alone. For example, given that for the differentiation between a mediastinal mass and physiological thymic or increased post-therapeutic uptake the use of PET imaging alone in children reveals lower accuracy [32], PET/MR might better differentiate from recurrent lymphoma or thymic rebound.
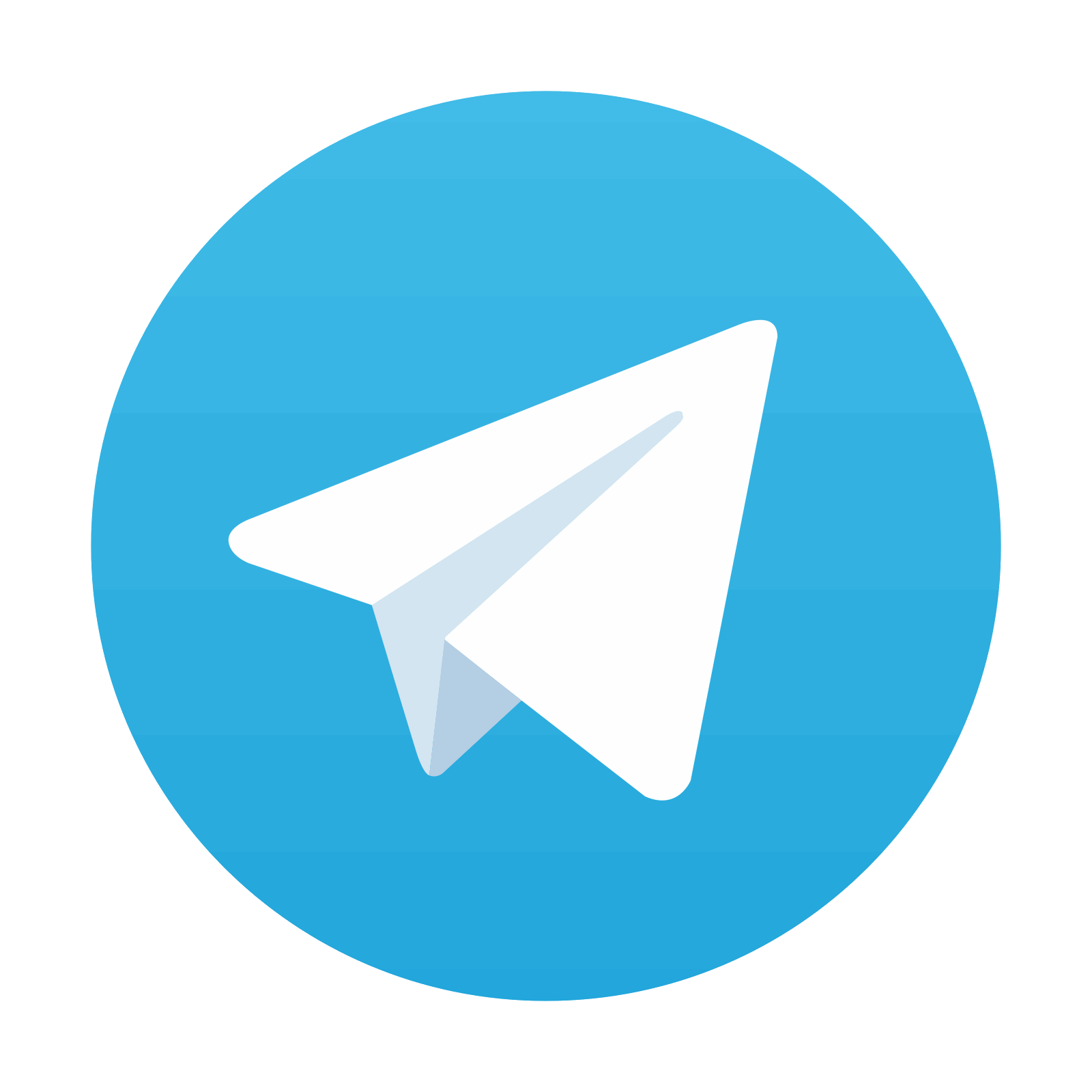
Stay updated, free articles. Join our Telegram channel

Full access? Get Clinical Tree
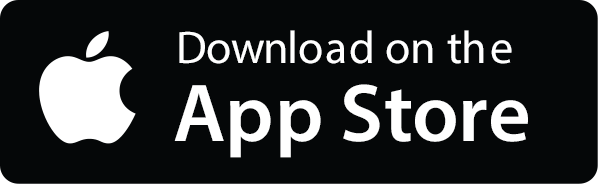
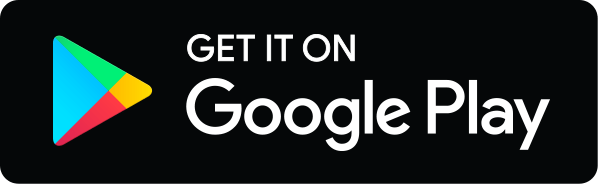
