Normal value of 1.0 in all patients and vessels
Independent of the microvasculature
Specific for the epicardial vessel
Not affected by hemodynamic changes
Accounts for collateral flow
Reproducible
Other indices have been introduced for the physiologic assessment of epicardial coronary artery disease. Coronary flow reserve (CFR) preceded FFR and is the best studied. It is defined as peak coronary flow divided by resting coronary flow [9]. CFR is limited by the fact that it interrogates the entire coronary circulation, both the epicardial vessel and the microvasculature. It does not have a clear normal value. It is affected by changes in resting hemodynamics, which limits its reproducibility. For these reasons, FFR has supplanted CFR as the reference standard for the invasively evaluating the physiology of the epicardial coronary artery [10].
Practical Aspects of Measuring FFR
FFR can be measured using one of two commercially available pressure wires. Both the St. Jude Medical and the Volcano Corporation systems use 0.014-in. wires with pressure sensors positioned 3 cm from the tip of the wire at the junction of the radiopaque and radiolucent portion of the wire. One begins by connecting the appropriate pressure wire to the system’s console. Most operators use 6-French guiding catheters, but FFR can be measured through 5- and 4-French systems [11].
After administering heparin at a dose of 50–70 units per kilogram and intracoronary nitroglycerin at 100–200 μg, the pressure wire is calibrated and advanced out of the guiding catheter such that the sensor is positioned at the catheter’s ostium. In this position the pressure wire recording is equalized to the guiding catheter pressure recording. The wire is then advanced to the distal two-third of the vessel being investigated. If one has difficulty advancing the wire, one can disconnect it from interface connector, to improve torqueability. Rarely, it is necessary to first wire the vessel with another wire and then position the pressure wire through an exchange catheter. If this is the case, the pressure wire must be equalized outside of the exchange catheter in the proximal vessel, removed from the vessel, and then positioned in the distal vessel through the exchange catheter.
Once the pressure wire is in position, hyperemia is achieved usually by administering intracoronary or intravenous adenosine. The dosage and advantages of each hyperemic agent will be discussed below. FFR is then measured as the mean distal pressure divided by the mean proximal pressure, during maximal hyperemia. The lowest value achieved is taken as the FFR.
In many cases, there is a focal lesion with some degree of diffuse coronary disease. In this setting, slowly pulling the pressure wire back during maximal hyperemia allows one to determine exactly where the pressure gradient is being generated and whether or not stenting the focal lesion will have a significant impact on the FFR. If there is a discrepancy between the pressure wire recording and the guiding catheter recording when the pressure sensor has been pulled back and positioned at the ostium of the guiding catheter, then drift in the pressure recording either from the wire or guiding catheter has occurred. Occasionally, flushing the guiding catheter with saline to remove contrast will eliminate this apparent drift. If not, then the FFR can be corrected by taking into account the drift, or the wire can be reequalized, and FFR can be remeasured once the wire is positioned again in the distal vessel.
If the lesion in question is deemed physiologically significant, percutaneous coronary intervention (PCI) can be performed using the pressure wire as the guidewire. After performing PCI, the pressure wire can be reconnected to the interface connector and FFR can be remeasured to assess the effect of PCI. It is important to wipe the distal end of the wire with a wet and then a dry gauze before reconnecting to the interface connector.
Inducing Hyperemia
One of the most important components to the accurate measurement of FFR is insuring that maximal hyperemia has been achieved. In order to fully assess the functional significance of a lesion, it is critical to maximize flow across the lesion and in that way simulates exercise. It is important to remember that the greater the flow across a particular stenosis, the greater the gradient and the lower the FFR.
FFR was originally validated using intravenous adenosine administered at 140 μg/kg/min via a central venous line (typically via the femoral vein); this method has been shown to induce maximal hyperemia, and for these reasons, intravenous adenosine is considered the reference standard and preferred approach [12]. In general, maximal hyperemia is achieved within 1 min after beginning the infusion. Typically, there will be a rise in mean aortic or proximal pressure, followed shortly thereafter by a decline in mean aortic pressure from the baseline. The distal pressure will not rise as much and will then decline more than the mean proximal pressure, depending on how severe the stenosis. FFR is measured at the lowest value during maximal hyperemia. Occasionally, heart block or other arrhythmias can create isolated drops in the FFR, which should be ignored.
When administered from a central vein, there is no advantage to increasing the dose of intravenous adenosine if one suspects inadequate hyperemia [12]. In this case, it is important to recheck the infusion to make sure it has been mixed and dosed correctly and that the stopcock on the venous sheath is positioned appropriately so that the infusion is entering the patient’s body. When administered from a peripheral vein, it may take longer before maximal hyperemia is achieved. In addition, there may be respiratory variation in the FFR due to variability of venous return from the peripheral vein. The lowest FFR value should be taken as the true FFR. From the peripheral vein, there may be an advantage to increasing the dose of adenosine to 280 μg/kg/min, if submaximal vasodilation is suspected, because of the short half-life of adenosine.
Intravenous adenosine is advantageous because hyperemia lasts as long as the infusion is continued, which allows for accurate measurement of FFR and the ability to perform a slow pullback of the pressure sensor in order to differentiate focal from diffuse disease or to assess serial stenoses in the same vessel. Intravenous adenosine does usually cause shortness of breath and/or chest discomfort, which is well tolerated, especially if the patient has been forewarned. It is also helpful, in a sense, because if the patient does not have this sensation, one should be concerned about whether maximal hyperemia has occurred, especially if the pressure gradient does not increase. Rarely, intravenous adenosine can cause bronchoconstriction in patients with severe asthma or chronic obstructive pulmonary disease. Another potential drawback to intravenous adenosine is that in the United States, it is more expensive than intracoronary adenosine.
Intracoronary adenosine should be administered through a guide catheter without sideholes and which is well seated in the coronary to insure that the medication is not spilling out into the aorta. Doses of at least 50 μg should be used at first and the operator should readily increase to at least 100 μg [13]. Occasionally, in the right coronary artery or dominant circumflex, transient heart block will occur. Hyperemia occurs immediately after administration so it is important to record pressure right after giving the adenosine. Peak hyperemia lasts on the order of 15–20 s. There are no significant side effects to intracoronary adenosine and in the United States, it is inexpensive. The main drawbacks to using intracoronary adenosine are that in a small portion of patients, maximal hyperemia is not achieved, when compared to intravenous adenosine [13]. In addition, because of the short half-life, a slow pullback of the pressure sensor cannot be performed.
Adenosine, delivered either via an intravenous or intracoronary route, is the most common drug used to achieve hyperemia. Outside of the United States, adenosine triphosphate is commonly used and acts in a similar fashion to adenosine. Papaverine is another medication that has been used in the past. In some respects, it is an ideal hyperemic agent. It is inexpensive and can be given easily using an intracoronary route. Maximal hyperemia lasts about 1 min, which allows one to get an accurate measurement of FFR and to perform a pullback of the pressure sensor. The major drawback to papaverine is that it can cause significant arrhythmias. In 1–2 % of patients, papaverine can cause ventricular tachycardia or torsades de pointes [14]. This side effect has limited the use of papaverine. In small studies, dobutamine and nitroprusside have been tested and shown to be useful hyperemic agents [8, 15]. The main drawback to dobutamine is the time it takes to achieve peak hyperemia, and the main drawback to nitroprusside is hypotension. Finally, there are a number of new selective adenosine 2 receptor agonists being tested or recently approved. These have the advantage of less dyspnea and chest pain when compared to adenosine [16]. More data and experience are necessary with the use of these agents (Table 15.2).
Table 15.2
Hyperemic agents
Agent | Administration | Peak effect | Side effects | Comments |
---|---|---|---|---|
Adenosine (or ATP) | IV at 140 μg/kg/min | Duration of infusion | Dyspnea, chest pain | Reference standard |
Adenosine (or ATP) | IC at 40 to >100 μg | 15 s | Transient AV block | Does not allow pullback |
Papaverine | IC at 10–20 mg | 60 s | Torsades de pointes | Not used commonly |
Nitroprusside | IC at 0.3–0.9 μg/kg | 30 s | Hypotension | Not well studied |
Dobutamine | IV at 20–50 μg/kg/min | Duration of infusion | Tachycardia | Slow onset |
Regadenoson | IV bolus of 0.4 mg | 2–3 min | Dyspnea, chest pain, headache | Not well studied with FFR |
Common Pitfalls of FFR Measurement
The most common pitfall when measuring FFR is not achieving maximal hyperemia. This usually occurs because the hyperemic agent is not delivered properly, for example, intracoronary adenosine entering the aorta predominantly, instead of the coronary due to poor seating of the guiding catheter or due to use of a guiding catheter with sideholes. Another example can occur when intravenous adenosine is given through a very small peripheral vein, resulting in metabolism before it reaches the heart. One should suspect inadequate hyperemia when the patient does not develop the typical dyspnea and chest heaviness with intravenous adenosine or when there is no change in the resting gradient with intracoronary adenosine.
The guiding catheter can be the source of another potential pitfall. If the coronary ostium is small or if there is some degree of ostial disease, then the guiding catheter, even if only 6 French, can sufficiently obstruct flow down the coronary so that maximal hyperemia does not occur [17]. This can be recognized by a ventricularized pressure tracing from the guiding catheter. It is easily corrected by simply unseating the guiding catheter, thereby allowing maximal hyperemia and an accurate measurement of FFR (Fig. 15.1).
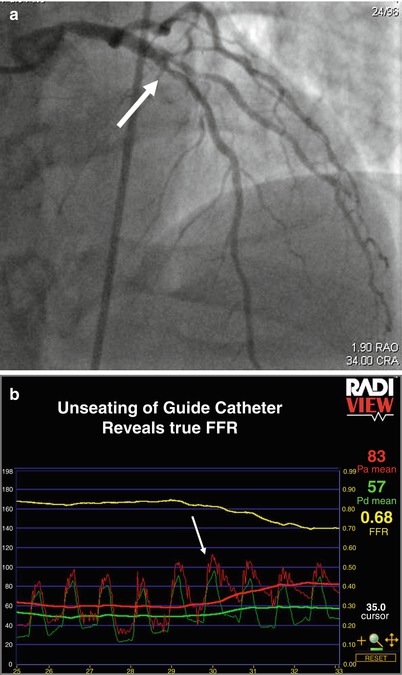
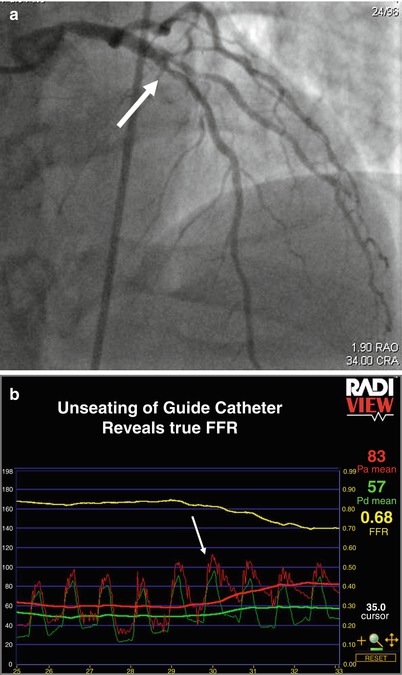
Fig. 15.1
(a) Deep-seated guide. Angiogram of the left coronary artery revealing mild ostial left main disease and moderate left anterior descending disease (white arrows). (b) Deep-seated guide. The red tracing represents pressure recorded from the guiding catheter, and the green tracing represents pressure recorded from the pressure wire. Initially, the guide is deep seated resulting in ventricularization of the pressure recording and blunting of the gradient and true FFR. After unseating the guiding catheter, maximal flow down the vessel can be achieved and the true FFR is displayed. After stenting the left anterior descending lesion, the FFR was 0.85, indicating that the combination of the ostial left main disease and the residual diffuse disease in the left anterior descending was not causing ischemia
The ingestion of caffeine soon before FFR measurement has been a concern because caffeine is a competitive inhibitor of the adenosine A2a receptor. However, in a small study, FFR was measured before and after patients were exposed to the equivalent of 2–3 cups of caffeine. The average FFR did not change significantly as a result of caffeine ingestion [18].
A final pitfall to consider when concerned about a false negative FFR is exercise-induced vasoconstriction. A patient may have a moderate stenosis, which itself is not ischemia-producing, but which contributes to a significant stenosis when the patient exercises and develops exercise-induced vasoconstriction. This scenario can result in angina and an abnormal exercise test. It is possible that a patient has this condition, but when FFR is measured in the catheterization laboratory with a pharmacologic agent inducing hyperemia, instead of exercise, vasoconstriction does not occur and the residual fixed stenosis is not severe enough to lead to an ischemic FFR [19].
FFR in Intermediate Coronary Disease
FFR was originally validated in patients with intermediate single-vessel coronary disease. In a landmark paper, Pijls and colleagues compared FFR in 45 consecutive patients with an intermediate coronary stenosis to a combination of three different noninvasive stress tests for reversible myocardial ischemia [20]. If anyone of the three stress tests was abnormal, the patient was diagnosed with ischemia. By incorporating all three stress tests into the definition of myocardial ischemia based on noninvasive testing, using Bayesian considerations, the authors established a reference standard with a very high accuracy to which FFR could be compared. In all 21 patients with an FFR < 0.75, at least one stress test was abnormal, giving FFR a specificity of 100 % using this cutoff. Of the 24 patients with an FFR ≥ 0.75, 21 had no ischemia on all 3 noninvasive stress tests, giving a sensitivity of 88 % and a diagnostic accuracy of 93 %.
Another important study establishing the safety of deferring PCI in patients with intermediate coronary disease and FFR values ≥0.75 was a randomized study evaluating 325 patients with moderate coronary lesions [21]. FFR was measured and if ≥0.75, the patient was randomized to either PCI or medical therapy. In the 91 patients randomized to deferral of PCI, the major adverse event rate at 2 years was 11 % compared to 17 % (p = 0.27) in the 90 patients with FFR ≥ 0.75 who were randomized to performance of PCI. Of note, the percentage of patients free from angina at 2 years was 70 % in the deferral group and 51 % in the performance group (p = 0.02). These findings were extended to 5-year follow-up in a subsequent publication which demonstrated continued safety of deferring PCI of lesions which are not hemodynamically significant [3]. The cardiac death and myocardial infarction rate at 5 years was 3.3 % in the deferral patients and 7.9 % in the performance group (p = 0.21) (Fig. 15.2). Other studies have confirmed the excellent outcomes of patients in whom PCI was deferred based on FFR values ≥0.75 [22].
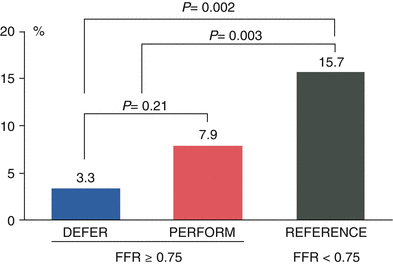
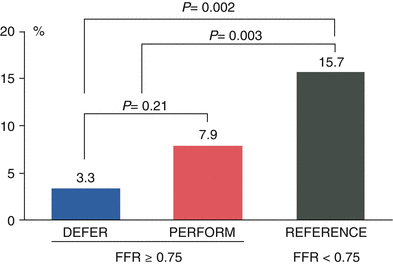
Fig. 15.2
DEFER study: 5-year cardiac death and myocardial infarction. Data from the DEFER trial showing low rates of cardiac death or myocardial infarction in patients with hemodynamically insignificant lesions assigned to medical therapy (DEFER arm), as compared to those subjected to percutaneous coronary intervention (PERFORM arm). The REFERENCE group represents patients with hemodynamically significant lesions treated with PCI (From Pijls et al. [3], with permission)
These data confirm that nonischemia-producing lesions when treated medically have a low event rate which is equal to or lower than the event rate when these lesions are revascularized with PCI. Ischemia-producing lesions (FFR < 0.75) appear to have a higher event rate when treated medically. For example, in a study of 107 patients with intermediate lesions and normal nuclear perfusion scans in the region subtended by the vessel with the intermediate lesion, the investigators measured FFR [23]. PCI was deferred on all of the lesions because the nuclear scan was normal. At 1 year, the major adverse event rate in the 14 % of patients in whom the FFR was <0.75 was significantly higher than in the patients in whom the FFR was ≥0.75 (27 % versus 9 %, p < 0.04). In another study of 407 patients with intermediate coronary lesions, the major adverse event rate was 21 % in the 34 patients with an FFR in the ischemic zone in whom revascularization was deferred compared to 6 % in the 99 patients with an ischemic FFR who were revascularized [24]. These data suggest that ischemia-producing lesions based on FFR measurement are at high risk for causing future events when treated with medical therapy alone.
FFR and the “Grey Zone”
Numerous studies from different investigators comparing FFR to a variety of noninvasive stress tests in a variety of patient populations have established a narrow cutoff value for FFR between 0.75 and 0.80 for identifying ischemia [25]. Most studies found that if the FFR was below 0.75, there is a 100 % specificity for ischemia. However, the sensitivity is less. By extending the cutoff value to 0.80, one loses a small amount of specificity, but gains sensitivity (fewer false negatives). From a practical perspective, if the FFR down a vessel is <0.75, one can be fairly certain myocardial ischemia is inducible, and if there is a focal narrowing, revascularization will improve symptoms and may improve prognosis. If the FFR is >0.80, then one can be fairly certain that significant ischemia is not present and multiple studies have shown excellent outcomes with medical therapy alone. When the FFR falls between 0.75 and 0.80, then one should apply clinical judgment. If the lesion is in the proximal left anterior descending artery and the patient has typical symptoms, then revascularization may be warranted. However, if, for example, the patient has atypical symptoms or no symptoms, an equivocal or negative noninvasive stress test, a diffuse pressure gradient on slow pullback of the pressure sensor without a focal step-up across the lesion, or is undergoing preoperative evaluation for noncardiac surgery, then one might opt for medical therapy.
FFR in Multivessel Coronary Disease
In patients with multivessel coronary disease, the diagnostic accuracy of noninvasive stress imaging studies is worse than in patients with single-vessel disease [4]. One of the advantages of an invasive assessment of coronary physiology with FFR is its unsurpassed spatial resolution. A patient with three-vessel coronary disease may have an unimpressive nuclear perfusion scan because of balanced ischemia [26]. However, FFR assessment of each vessel can determine the contribution of each to the patient’s ischemia and symptoms.
Another common scenario is a patient with inferior ischemia on a noninvasive stress test and an occluded right coronary artery, which receives collaterals from a left anterior descending coronary artery, which itself has a moderate proximal lesion. The nuclear scan tends to identify the area of greatest ischemia in the inferior region of the left ventricle and may not detect less severe ischemia in the anterior region. FFR assessment of the left anterior descending artery allows determination of whether the moderate lesion is also contributing to the patient’s symptoms and potentially compromising the collaterals to the right coronary artery.
A final scenario highlighting the superior spatial resolution of FFR is the vessel with tandem lesions or a focal lesion within diffuse disease. By performing a slow pullback of the pressure wire, one can often identify the region responsible for the majority of the pressure gradient and thereby focus PCI to the area. This will be discussed in further detail below.
Because of its excellent spatial resolution, one might anticipate that FFR would be useful in guiding PCI in patients with multivessel coronary disease. This was tested in the fractional flow reserve versus Angiography for Multivessel Evaluation (FAME) trial, a prospective, multicenter, international, randomized study comparing an FFR-guided strategy to an angiography-guided strategy for performing PCI in over 1,000 patients with multivessel coronary disease [6, 27]. In order to be included in this study, patients were required to have two or three major epicardial vessels with >50 % stenoses, which the operator felt required PCI with drug-eluting stents based on the angiographic appearance and the clinical scenario. Patients with previous coronary artery bypass graft surgery, significant left main disease, or within 5 days of an ST segment elevation myocardial infarction, were excluded from the study. Once the operator identified which lesions were to be stented, the patient was then randomized to either angiographic guidance or FFR guidance. If randomized to angiographic guidance, the operator stented all of the identified lesions. If randomized to FFR guidance, FFR was measured first and only if <0.80 was the lesion stented. The primary endpoint of the study was 1-year rate of major adverse events including death, myocardial infarction, or the need for repeat revascularization.
At 1 year, the average number of stents placed per patient in the 509 patients randomized to FFR guidance was significantly lower than the number placed in the 496 patients randomized to angiographic guidance (2.7 versus 1.9, p < 0.001). The amount of contrast required was also significantly less (302 versus 272 mL, p < 0.001). Yet, the time to perform the procedure was similar in both groups (70 versus 71 min, p = 0.51), likely because although measuring FFR may add sometime to the procedure, by decreasing the number of lesions which require PCI, it also significantly saves time and contrast.
The main finding from the FAME trial, however, was the significant decrease in major adverse events at 1 year; these occurred in 18.3 % of the angiography-guided patients and 13.2 % of the FFR-guided patients (p = 0.02). This was due to similar relative reductions of 30–40 % in the occurrence of each component of the primary endpoint, death, MI, and the need for repeat revascularization (Fig. 15.3). In addition, the rate of death and MI alone was significantly reduced by FFR guidance from 11.1 to 7.3 %, p = 0.04. These findings have been extended out to 2 years, in a subsequent publication showing a continued significant decrease in the rate of death and MI by FFR guidance from 12.9 to 8.4 %, p = 0.02 [28].
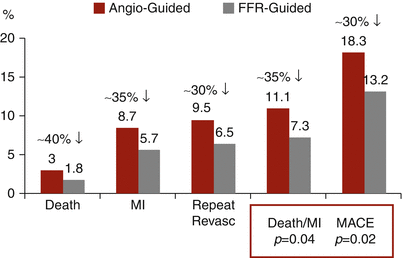
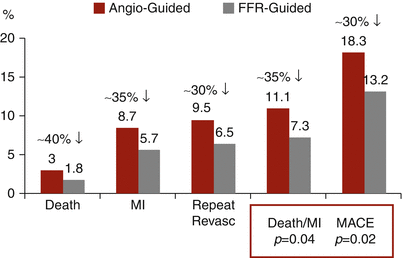
Fig. 15.3
FAME trial: 1-year outcomes. Data from the FAME trial [6] demonstrating a 30–40 % reduction of each endpoint with FFR-guided PCI as compared to angiography-guided PCI. The combination of death and myocardial infarction (MI) was significantly reduced, as was the primary endpoint, major adverse cardiac events (MACE)
The results of the FAME study support a new paradigm termed “functionally complete” revascularization, as opposed to the traditional anatomic complete revascularization. For many years we have focused on revascularizing every angiographically significant coronary stenosis. FAME has taught us that the functional significance of the lesion should dictate whether or not PCI is performed, not the angiographic appearance. In the FFR-guided patients in the FAME study, 20 % of those lesions between 70 and 90 % narrowed had an FFR > 0.80 and did not require PCI [29]. Of the lesions which were 50–70 % narrowed, only 35 % had an FFR ≤ 0.80 and required PCI. This resulted in over 500 angiographically significant appearing lesions being treated medically. At 2 years, the rate of MI and the rate of revascularization for these deferred lesions were only 0.2 and 3.2 %, respectively, providing further reassurance in the safety of this approach [28].
These data also help us to understand why the FFR-guided strategy was superior to the angiography-guided one. If the event rate in functionally nonsignificant lesions is so low with medical therapy, then PCI is unlikely to improve the situation. By performing PCI on these lesions anyway, one incurs the short-term and longer-term risks of PCI, such as myocardial infarction, stent thrombosis, and restenosis, without reaping the reward of relieving myocardial ischemia. FFR guidance allows the operator to achieve complete relief of ischemia (functionally complete revascularization), but avoids unnecessary PCI of nonischemia-producing lesions.
The additional information obtained from FFR may have important implications on our treatment decisions in patients with multivessel disease. The SYNTAX score, an angiography-based grading system, has been advocated as a means for deciding whether or not a patient with multivessel coronary disease would have a better outcome with coronary artery bypass graft surgery or with PCI [30]. This approach stems from data showing that patients with more complex coronary disease resulting in a high SYNTAX score tended to have better outcomes when randomized to coronary artery bypass grafting instead of PCI in the SYNTAX study [31]. However, the SYNTAX score is limited by the fact that it is based on the coronary angiogram. We have a greater appreciation now regarding the inability of one to determine if a stenosis is functionally significant when basing that decision on the angiogram alone.
Recently, a functional SYNTAX score was proposed, in which only lesions with an FFR ≤ 0.80 are scored when calculating the SYNTAX score, and functionally insignificant lesions are neglected [32
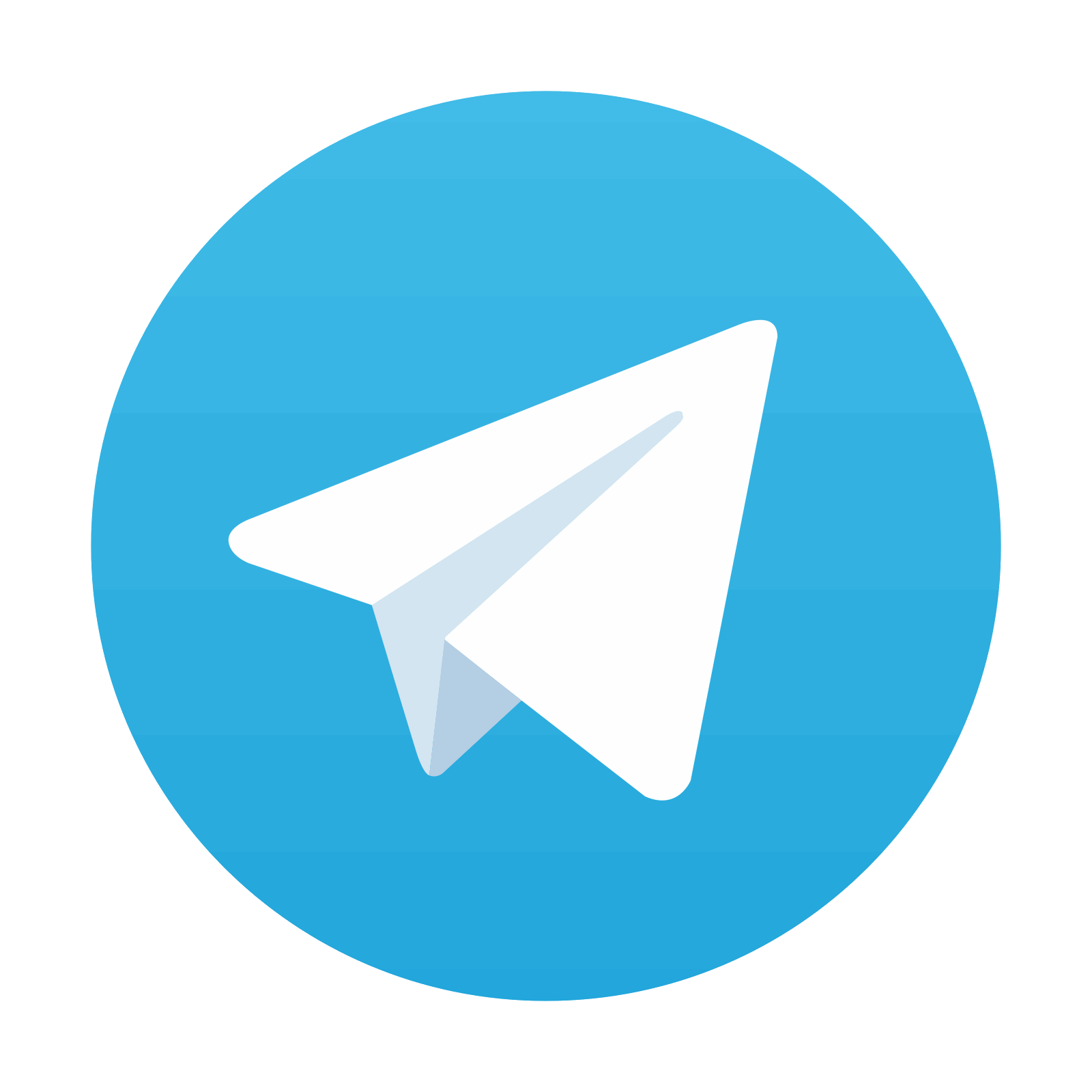
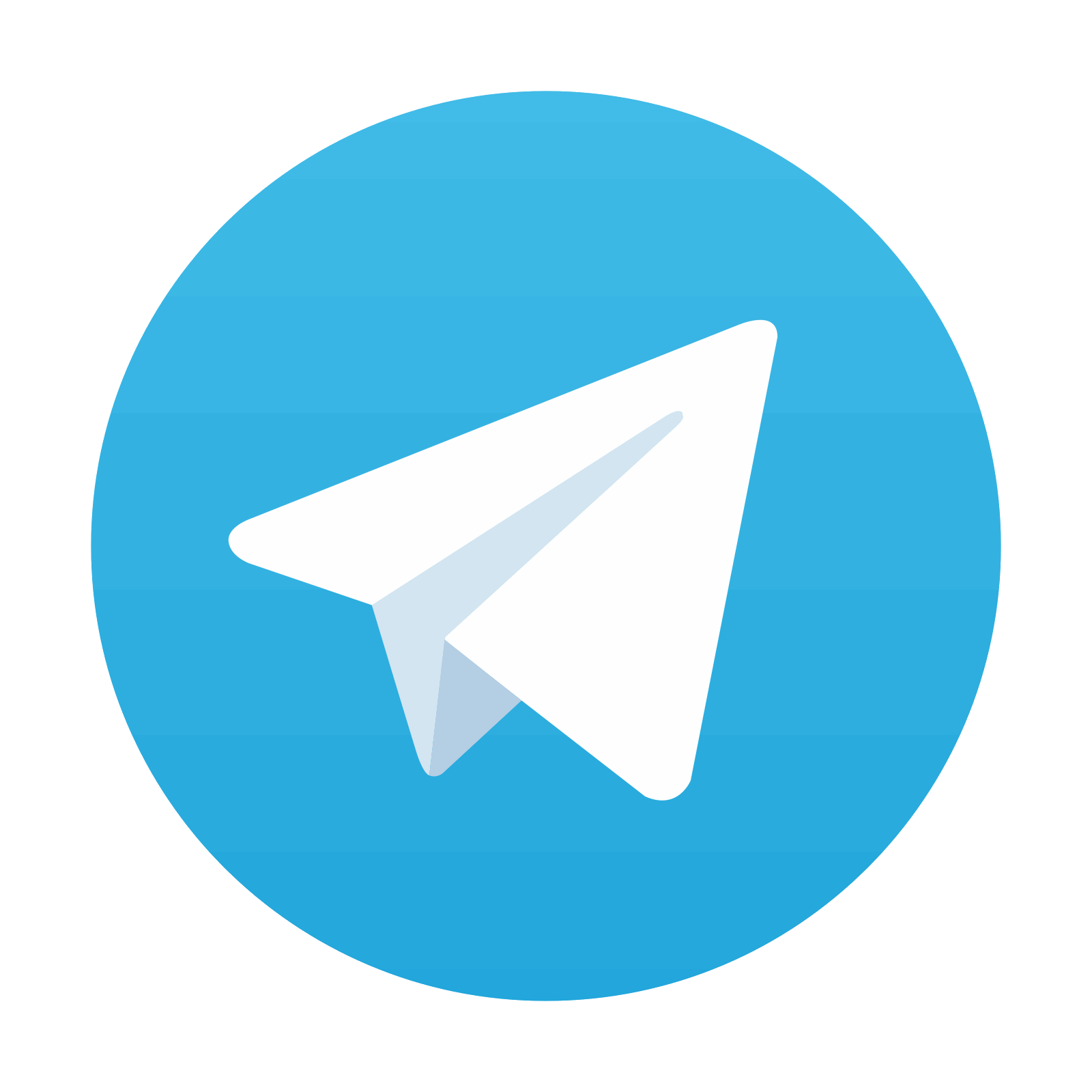
Stay updated, free articles. Join our Telegram channel

Full access? Get Clinical Tree
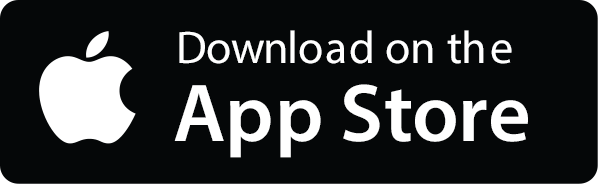
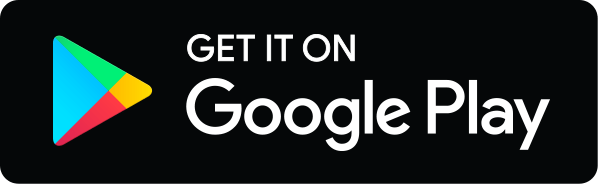